CHAPTER 4 The Nervous System: Introduction to Cells and Systems
The nervous system is a communications and control network that allows an organism to interact in appropriate ways with its environment. The environment includes both the external environment (the world outside the body) and the internal environment (the components and cavities of the body). The nervous system can be divided into central and peripheral parts, each with further subdivisions. The peripheral nervous system (PNS) provides an interface between the environment and the central nervous system (CNS). It includes sensory (or primary afferent) neurons, somatic motor neurons, and autonomic motor neurons. Autonomic motor neurons are discussed in Chapter 11.
CELLULAR COMPONENTS OF THE NERVOUS SYSTEM
Neurons
The functional unit of the nervous system is the neuron (Fig. 4-1), and neural circuits are made up of synaptically interconnected neurons. Neural activity is generally coded by sequences of action potentials propagated along axons in the neural circuits (see Chapter 5). The coded information is passed from one neuron to the next by synaptic transmission (see Chapter 6). In synaptic transmission, the action potentials that reach a presynaptic ending usually trigger the release of a chemical neurotransmitter. The neurotransmitter can either excite the postsynaptic cell (possibly to discharge one or more action potentials), inhibit the activity of the postsynaptic cell, or influence the action of other axon terminals.
The typical neuron consists of a cell body, or soma, with a variable number of branch-like dendrites and a single process, the axon, that extend from the soma. The cell body (perikaryon, soma) of the neuron contains the nucleus and nucleolus of the cell and also possesses a well-developed biosynthetic apparatus for manufacturing membrane constituents, synthetic enzymes, and other chemical substances needed for the spe-cialized functions of nerve cells. The neuronal biosynthetic apparatus includes Nissl bodies, which are stacks of rough endoplasmic reticulum, and a prominent Golgi apparatus. The soma also contains numerous mitochondria and cytoskeletal elements, including neurofilaments and microtubules. In contrast to most cells in the body, neurons have an enormous variety of shapes and sizes. Neurons with similar morphologies often characterize specific regions of the CNS. Morphological variation is produced by differences in the branching pattern of dendrites and the axon.
Dendrites are tapering and branching extensions of the soma and generally convey information toward the cell body. A neuron’s branched set of dendrites is termed its dendritic tree. In some neurons the dendrites are longer than 1 mm, and they may account for more than 90% of the surface area. The proximal dendrites (near the cell body) contain Nissl bodies and parts of the Golgi apparatus. However, the main cytoplasmic organelles in dendrites are microtubules and neurofilaments. Because the dendrites are the major area that receives synaptic input from other neurons, the shape and size of the dendritic tree, as well as the population and distribution of channels in the dendritic membrane, are important determinants of how the synaptic input will affect the neuron. Synaptic input to dendrites can be passively conducted to the cell body, but these signals usually diminish as they pass to the soma and, in large cells, would have little influence on it. However, the dendrites of large neurons may have active zones, often using Ca++-dependent, voltage-dependent channels, that can produce voltage spikes important in the integration of multiple synaptic input to a single neuron (see Chapter 6).
The axon is an extension of the cell that conveys the output of the cell to the next neuron or, in the case of a motor neuron, to a muscle. In general, each neuron has only one axon, and it is usually of uniform diameter. The length and diameter of axons vary with the neuronal type. Some axons do not extend much beyond the length of the dendrites, whereas others may be a meter or more long. Axons may have orthogonal branches en passant, but they often end in a spray of branches called terminal arborization (Fig. 4-1). The size, shape, and organization of the terminal arborization determine which other cells it will contact. The axon arises from the soma (or sometimes from a proximal dendrite) in a specialized region called the axon hillock. The axon hillock and axon differ from the soma and proximal dendrites in that they lack rough endoplasmic reticulum, free ribosomes, and Golgi apparatus. The axon hillock is usually the site where action potentials are generated because it has a high concentration of the necessary channels (see Chapters 5 and 6). Because the soma is the metabolic engine for the axon, it is only reasonable that a large soma is required to support large, long axons and that very small neurons are associated with short axons. Thus, axons not only transmit information in neural circuits but also convey chemical substances toward or away from the synaptic terminals by axonal transport. For this reason also, axons degenerate when disconnected from the cell body.
Axonal Transport
Most axons are too long to allow efficient movement of substances from the soma to the synaptic endings by simple diffusion. Membrane and cytoplasmic components that originate in the biosynthetic apparatus of the soma must be distributed to replenish secreted or inactivated materials along the axon and, especially, to the presynaptic elements at the terminal end. A special transport mechanism called axonal transport accomplishes this distribution (Fig. 4-2).
Several types of axonal transport exist. Membrane-bound organelles and mitochondria are transported relatively rapidly by fast axonal transport. Substances that are dissolved in cytoplasm, such as proteins, are moved by slow axonal transport. In mammals, fast axonal transport proceeds as rapidly as 400 mm/day, whereas slow axonal transport occurs at about 1 mm/day. Synaptic vesicles, which travel by fast axonal transport, can travel from the soma of a motor neuron in the spinal cord to a neuromuscular junction in a person’s foot in about 2.5 days. In comparison, the movement of some soluble proteins over the same distance can take nearly 3 years.
Axonal transport requires metabolic energy and involves calcium ions. Microtubules provide a system of guide wires along which membrane-bound organelles move (Fig. 4-2). Organelles attach to microtubules through a linkage similar to that between the thick and thin filaments of skeletal muscle fibers. Ca++ triggers movement of the organelles along the microtubules. Special microtubule-associated motor proteins called kinesin and dynein are required for axonal transport.
THE SUPPORTIVE MATRIX OF THE CENTRAL NERVOUS SYSTEM
Neuroglia
The major nonneuronal cellular elements of the nervous system are the neuroglia (Fig. 4-3), or supportive cells. Neuroglial cells in the human CNS outnumber neurons by an order of magnitude: there are about 1013 neuroglia and 1012 neurons.
Neuroglia do not participate directly in the short-term communication of information through the nervous system, but they do assist in that function. For example, some types of neuroglial cells take up neurotransmitter molecules and in this manner directly influence synaptic activity. Others provide many axons with myelin sheaths that speed up the conduction of action potentials along axons (see Chapter 5) and thereby allow some axons to communicate rapidly over relatively long distances.
Neuroglial cells in the CNS include astrocytes and oligodendroglia (Fig. 4-3) and, in the PNS, Schwann cells and satellite cells. Microglia and ependymal cells are also considered to be central neuroglial cells.
Astrocytes (named for their star shape) help regulate the microenvironment of the CNS. Their processes contact neurons and surround groups of synaptic endings, isolating them from adjacent synapses and the general extracellular space. Astrocytes also have foot processes that contact the capillaries and connective tissue at the surface of the CNS, the pia mater (Fig. 4-3). These foot processes may help mediate the entry of substances into the CNS. Astrocytes can actively take up K+ ions and neurotransmitter substances, which they metabolize, biodegrade, or recycle. Thus, astrocytes serve to buffer the extracellular environment of neurons with respect to both ions and neurotransmitters. The cytoplasm of astrocytes contains glial filaments, which provide mechanical support for CNS tissue. After injury, the astrocytic processes that contain these glial filaments hypertrophy and form a glial “scar.”
Many axons are surrounded by a myelin sheath, which is a spiral multilayered wrapping of glial cell membrane (Fig. 4-4; see also Fig. 4-1). In the CNS, myelinated axons are ensheathed by the membranes of oligodendroglia (Fig. 4-4, A), and unmyelinated axons are bare. In the PNS, unmyelinated axons are surrounded by Schwann cells (Fig. 4-4, C), whereas myelinated axons are ensheathed by multiply wrapped membranes of Schwann cells, much as the oligodendroglia ensheath central axons. One major distinction is that many central axons can be myelinated by a single oligodendroglial cell, whereas in the periphery, each Schwann cell myelinates only one axon. Myelin increases the speed of action potential conduction, in part by restricting the flow of ionic current to small unmyelinated portions of the axon between adjacent sheath cells, the nodes of Ranvier (Fig. 4-4, B; see also Chapter 5).
< div class='tao-gold-member'>
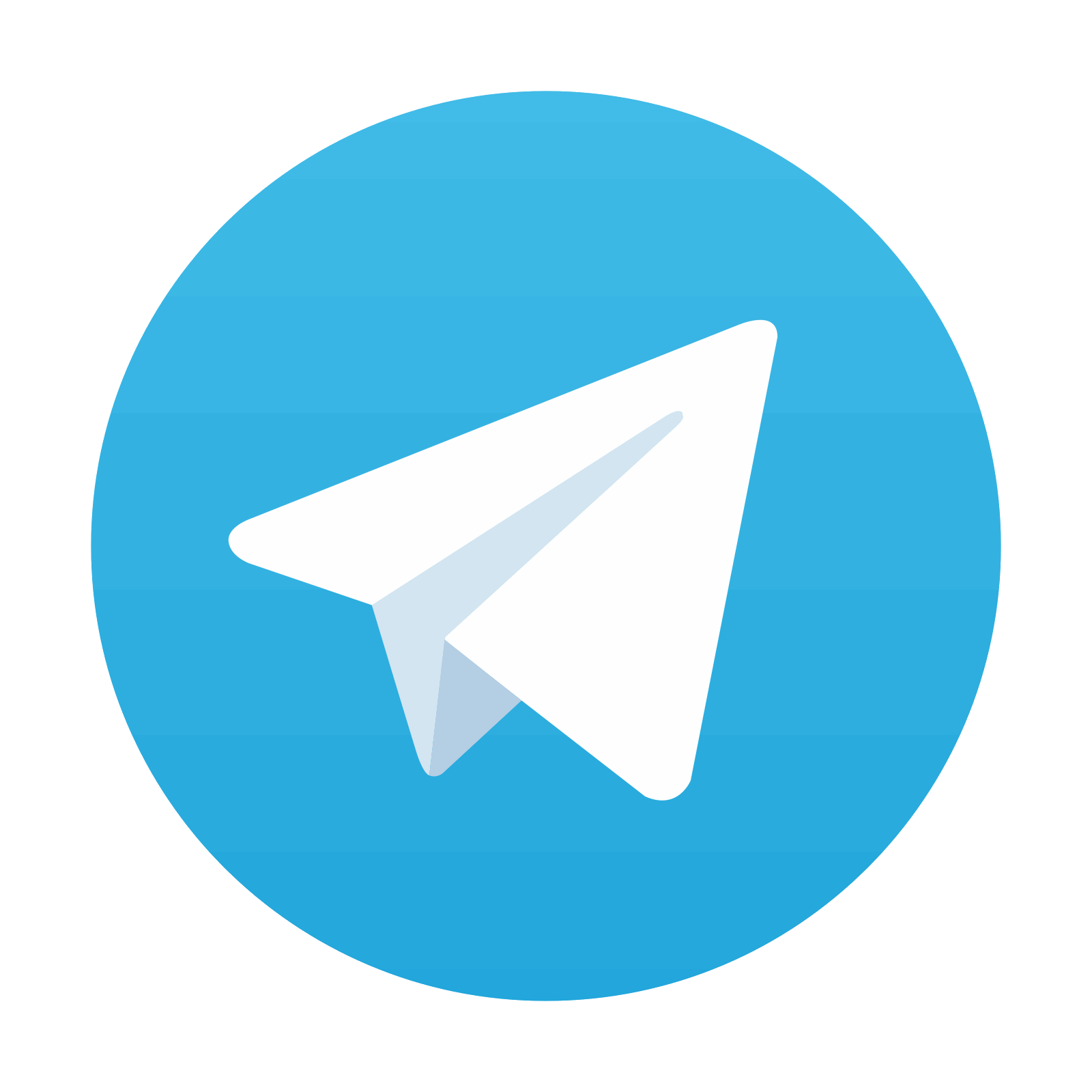
Stay updated, free articles. Join our Telegram channel

Full access? Get Clinical Tree
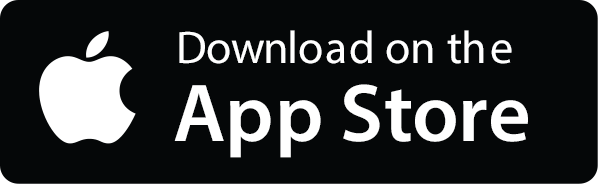
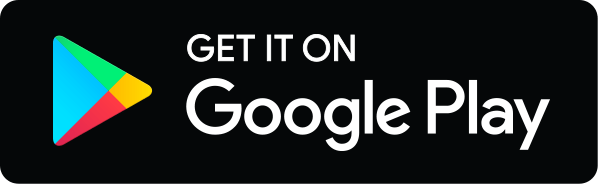