CHAPTER 43 The Male and Female Reproductive Systems
The two most basic components of the reproductive system are the gonads and the reproductive tract. The gonads (testes and ovaries) perform an endocrine function, which is regulated within a hypothalamic-pituitary-gonadal axis. The gonads are distinct from other endocrine glands in that they also perform an exocrine function (gametogenesis). The reproductive tract is involved in several aspects of gamete development, function, and transport and, in women, allows fertilization, implantation, and gestation. Normal gametogenesis in the gonads and the development and physiology of the reproductive tract are absolutely dependent on the endocrine function of the gonads. The clinical ramifications of this hormonal dependence include infertility in the face of low sex hormone production, ambiguous genitalia in dysregulated hormone or receptor expression, and hormone-responsive cancers, especially uterine and breast cancer in women and prostate cancer in men.
THE TESTIS
Histophysiology
Unlike the ovaries, the testes reside outside the abdominal cavity in the scrotum (Fig. 43-1). This location maintains testicular temperature at about 2 degrees lower than body temperature, which is crucial for optimal sperm development. The human testis is covered by a connective tissue capsule and is divided into about 300 lobules by fibrous septa (Fig. 43-2). Within each lobule are two to four loops of seminiferous tubules. Each loop empties into an anastomosing network of tubules called the rete testis. The rete testis is continuous with small ducts, the efferent ductules, that lead the sperm out of the testis into the head of the epididymis on the superior pole of the testis (Fig. 43-2). Once in the epididymis, the sperm pass from the head, to the body, to the tail of the epididymis and then to the vas (ductus) deferens. Viable sperm can be stored in the tail of the epididymis and the vas deferens for several months.
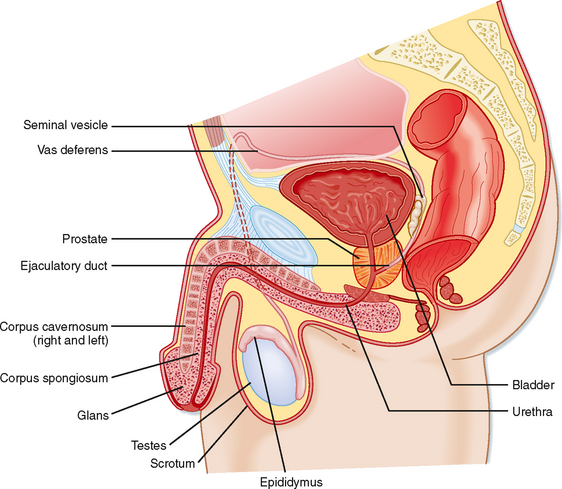
Figure 43-1 Anatomy of the male reproductive system.
(Modified from Drake RL et al: Gray’s Anatomy for Students. Philadelphia, Churchill Livingstone, 2005.)
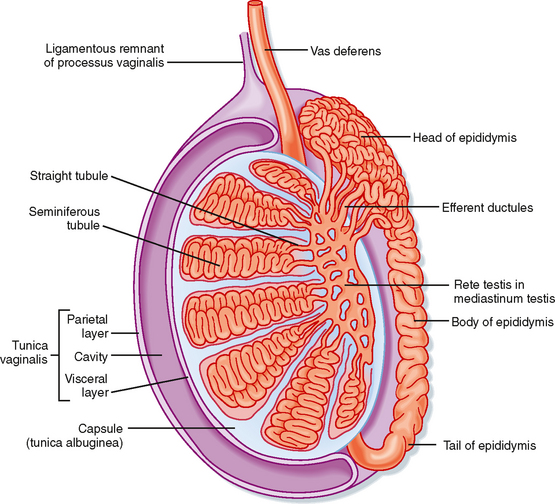
Figure 43-2 Anatomy and organization of the testis.
(Modified from Drake RL et al: Gray’s Anatomy for Students. Philadelphia, Churchill Livingstone, 2005.)
The presence of the seminiferous tubules creates two compartments within each lobule: an intratubular compartment, which is composed of the seminiferous epithelium of the seminiferous tubule, and a peritubular compartment, which is composed of neurovascular elements, connective tissue cells, immune cells, and the “interstitial cells of Leydig,” whose main function is to produce testosterone (Fig. 43-3).
The Intratubular Compartment
The seminiferous tubule is lined by a complex seminiferous epithelium composed of two cell types: sperm cells in various stages of spermatogenesis and the Sertoli cell, which is a “nurse cell” in intimate contact with all sperm cells (Fig. 43-4).
Developing Sperm Cells
Spermatogenesis involves the processes of mitosis and meiosis. Stem cells, called spermatogonia, reside at the basal level of the seminiferous epithelium (Fig. 43-4). Spermatogonia divide mitotically to generate daughter spermatogonia (spermatocytogenesis). One or more spermatogonia remain within the stem cell population, firmly adherent to the basal lamina. However, the majority of these daughter spermatogonia enter meiotic division, which results in haploid spermatozoa on completion of meiosis. These divisions are accompanied by incomplete cytokinesis such that all daughter cells remain interconnected by a cytoplasmic bridge. This configuration contributes to the synchrony of development of a clonal population of sperm cells. Spermatogonia migrate apically away from the basal lamina as they enter the first meiotic prophase. At this time they are called primary spermatocytes (Fig 43-4). During the first meiotic prophase, the hallmark processes of sexual reproduction involving chromosomal reduplication, synapsis, crossing over, and homologous recombination take place. Completion of the first meiotic division gives rise to secondary spermatocytes, which quickly (i.e., within 20 minutes) completes the second meiotic division. The initial products of meiosis are haploid spermatids (Fig. 43-4). Spermatids are small, round cells that undergo a remarkable metamorphosis called spermiogenesis (Fig. 43-5). The products of spermiogenesis are the streamlined spermatozoa. As the spermatid matures into a spermatozoon, the size of the nucleus decreases and a prominent tail is formed. The tail contains microtubular structures that propel sperm, similar to a flagellum. The chromatin material in the sperm nucleus condenses, and most of the cytoplasm is lost. The acrosome is a membrane-enclosed structure on the head of the sperm that acts as a lysosome and contains hydrolytic enzymes that are important for fertilization. These enzymes remain inactive until the acrosomal reaction occurs (see later).
Spermatozoa (Fig. 43-4) are found at the luminal surface of the seminiferous tubule. Release of sperm, or spermiation, is controlled by Sertoli cells. The process of spermatogenesis takes about 72 days. A cohort of adjacent spermatogonia enter the process every 16 days so that the process is staggered at one point along a seminiferous tubule. In addition, the process is staggered along the length of a seminiferous tubule (i.e., not all spermatogonia enter the process of spermatogenesis at the same time along the entire length of the tubule or in synchrony with every other tubule; there are about 500 seminiferous tubules per testis; see later). Because the seminiferous tubules within one testis are about 400 m in length, spermatozoa are continually being generated at many sites within the testis at any given time.
The Sertoli Cell
Sertoli cells are the true epithelial cells of the seminiferous epithelium and extend from the basal lamina to the lumen (Fig. 43-4). Sertoli cells surround sperm cells and provide structural support within the epithelium, and they form adhering and gap junctions with all stages of sperm cells. Through the formation and breakdown of these junctions, Sertoli cells guide sperm cells toward the lumen as they advance to later stages of spermatogenesis. Spermiation requires the final breakdown of Sertoli–sperm cell junctions.
Another important structural feature of Sertoli cells is the formation of tight junctions between adjacent Sertoli cells (Fig. 43-6). These Sertoli-Sertoli cell occluding junctions divide the seminiferous epithelium into a basal compartment containing the spermatogonia and early-stage primary spermatocytes and an adluminal compartment containing later-stage primary spermatocytes and all subsequent stages of sperm cells. As early primary spermatocytes move apically from the basal to the adluminal compartment, the tight junctions need to be disassembled and reassembled. These tight junctions form the physical basis for the blood-testis barrier (Fig. 43-6), which creates a specialized, immunologically safe microenvironment for developing sperm. By blocking paracellular diffusion, the tight junctions restrict movement of substances between blood and the developing germ cells through a trans–Sertoli cell transport pathway and, in this manner, allow the Sertoli cell to control the availability of nutrients to germ cells.
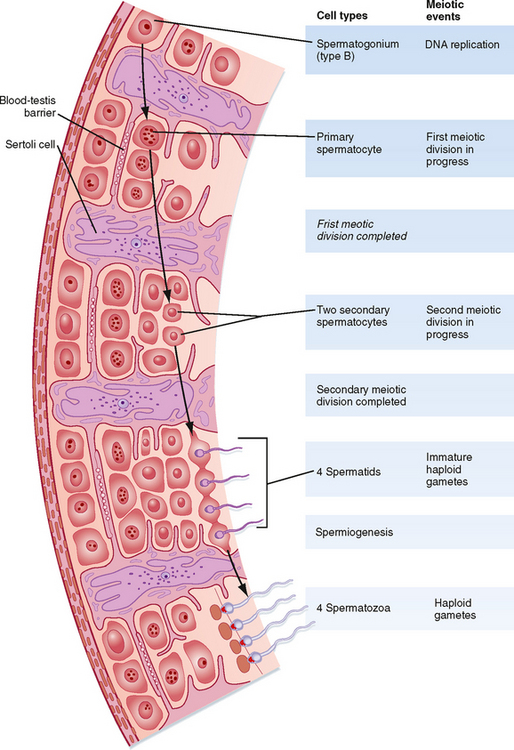
Figure 43-6 Interactions among the various cells of the testis in the hormonal regulation of spermatogenesis.
(From Carlson BM: Human Embryology and Developmental Biology. Philadelphia, Mosby, 2004.)
Healthy Sertoli cell function is essential for sperm cell viability and development. In addition, spermatogenesis is absolutely dependent on testosterone produced by peritubular Leydig cells (see later), yet it is the Sertoli cells that express the androgen receptor, not the developing sperm cells. Similarly, the pituitary hormone follicle-stimulating hormone (FSH) is also required for maximal sperm production, and again, it is the Sertoli cell that expresses the FSH receptor, not the developing sperm. Thus, these hormones support spermatogenesis indirectly through stimulation of Sertoli cell function.
The Peritubular Compartment
The peritubular compartment contains the primary endocrine cell of the testis, the Leydig cell (Fig. 43-7). This compartment also contains the common cell types of loose connective tissue and an extremely rich peritubular capillary network that provides nutrients to the seminiferous tubules (by way of Sertoli cells) while conveying testosterone away from the testes to the peripheral circulation.
The Leydig Cell
Leydig cells are steroidogenic stromal cells. These cells synthesize cholesterol de novo, as well as acquire it through low-density lipoprotein (LDL) receptors and high-density lipoprotein (HDL) receptors (also called scavenger receptor BI [SR-BI]), and store cholesterol as cholesterol esters, as described for adrenocortical cells (see Chapter 42). Free cholesterol is generated by a cholesterol ester hydrolase and transferred to the outer mitochondrial membrane and then to the inner mitochondrial membrane in a steroidogenic acute regulatory (StAR) protein–dependent manner. As in all steroidogenic cells, cholesterol is converted to pregnenolone by CYP11A1. Pregnenolone is then processed to progesterone, 17-hydroxyprogesterone, and androstenedione by 3β-hydroxysteroid dehydrogenase (3β-HSD) and CYP17 (Fig. 43-8). Recall from Chapter 42 that CYP17 is a bifunctional enzyme with 17-hydroxylase activity and 17,20-lyase activity. CYP17 displays a robust level of both activities in the Leydig cell. In this respect the Leydig cell is similar to the zona reticularis cell, except that it expresses a higher level of 3β-HSD, so the Δ4 pathway is ultimately favored. Another major difference is that the Leydig cell expresses a Leydig cell–specific isoform of 17β-hydroxysteroid dehydrogenase (17β-HSD type 3), which converts androstenedione to testosterone (Fig. 43-8).
FATES AND ACTIONS OF ANDROGENS
Intratesticular Androgen
The testosterone produced by Leydig cells has several fates and multiple actions. Because of the proximity of Leydig cells to the seminiferous tubules, significant amounts of testosterone diffuse into the seminiferous tubules and become concentrated within the adluminal compartment by ABP (Fig. 43-8). Testosterone levels within the seminiferous tubules that are greater than 100 times more concentrated than circulating testosterone levels are absolutely required for normal spermatogenesis. As mentioned earlier, Sertoli cells express the enzyme CYP19 (aromatase), which converts a small amount of testosterone into the highly potent estrogen estradiol-17β. Human sperm cells express at least one isoform of the estrogen receptor, and there is some evidence from aromatase-deficient men that this locally produced estrogen optimizes spermatogenesis in humans.
Peripheral Conversion to Estrogen
In several tissues (especially adipose tissue), testosterone is converted to estrogen (Fig. 43-8). Studies involving men with aromatase deficiency have shown that an inability to produce estrogen results in tall stature because of the lack of epiphyseal closure in long bones and osteoporosis. Thus, peripheral estrogen plays an important role in bone maturation and biology in men. These studies also implicated estrogen in promoting insulin sensitivity, improving lipoprotein profiles (i.e., increasing HDL, decreasing triglycerides and LDL), and exerting negative feedback on pituitary gonadotropins.
Peripheral Conversion to Dihydrotestosterone
Testosterone can also be converted into a potent, nonaromatizable androgen, 5α-dihydrotestosterone (DHT), by the enzyme 5α-reductase (Fig. 43-8). There are two isoforms of 5α-reductase, type 1 and type 2. Major sites of 5α-reductase 2 expression are the male urogenital tract, genital skin, hair follicles, and liver. 5α-Reductase 2 generates DHT, which is required for masculinization of the external genitalia in utero and for many of the changes associated with puberty, including growth and activity of the prostate gland (see later), growth of the penis, darkening and folding of the scrotum, growth of pubic and axillary hair, growth of facial and body hair, and increased muscle mass (Fig. 43-9). The onset of 5α-reductase 1 expression occurs at puberty. This isozyme is expressed primarily in the skin and contributes to sebaceous gland activity and the acne associated with puberty. Because DHT has strong growth-promoting (i.e., trophic) effects on its target organs, the development of selective 5α-reductase 2 inhibitors has benefited the treatment of prostatic hypertrophy and prostatic cancer.
Peripheral Testosterone Actions
Testosterone has a direct action (i.e., without conversion to DHT) in several cell types (Fig. 43-9). As mentioned earlier, testosterone regulates Sertoli cell function. It induces development of the male tract from the mesonephric duct in the absence of 5αreductase. Testosterone has several metabolic effects, including increasing very low density lipoprotein (VLDL) and LDL while decreasing HDL, promoting the deposition of abdominal adipose tissue, increasing red blood cell production, promoting bone growth and health, and exerting a protein anabolic effect on muscle. Testosterone is sufficient to maintain erectile function and libido.
Mechanism of Androgen Action
Testosterone and DHT act through the same androgen receptor (AR). The AR resides in the cytoplasm bound to chaperone proteins in the absence of ligand. Testosterone-AR binding or DHT-AR binding causes dissociation of the chaperone proteins, followed by nuclear translocation of the androgen-AR complex, dimerization, binding to an androgen response element (ARE), and recruitment of coactivator proteins and general transcription factors to the vicinity of a specific gene’s promoter. It remains unclear how testosterone and DHT differ in their ability to activate the AR in the context of different cell types, although the presence of different coactivator proteins in different cell types is probably involved.
HYPOTHALAMIC-PITUITARYTESTICULAR AXIS
The testis is regulated by an endocrine axis (Fig. 43-10) involving parvicellular hypothalamic gonadotropin-releasing hormone (GnRH) neurons and pituitary gonadotropes that produce both luteinizing hormone (LH) and follicle-stimulating hormone (FSH).
Regulation of Leydig Cell Function
The Leydig cell expresses the LH receptor, which acts on Leydig cells much like adrenocorticotropic hormone (ACTH) does on zona fasciculata cells in the adrenal cortex (see Chapter 42). Rapid effects include hydrolysis of cholesterol esters and new expression of StAR protein. Less acute effects include an increase in steroidogenic enzyme gene expression and expression of the LDL receptor and SR-BI (the HDL receptor). Over the long term, LH promotes Leydig cell growth and proliferation.
Testosterone negatively feeds back on LH production by the pituitary gonadotrope as testosterone and its metabolites, DHT, and estradiol-17β. All three steroid hormones inhibit the expression of LH-β and the GnRH receptor. These steroids also inhibit the release of GnRH by the hypothalamic neurons (Fig. 43-10).
Regulation of Sertoli Cell Function
The Sertoli cell is stimulated by both testosterone and FSH. In addition to stimulating the synthesis of proteins involved in the “nurse cell” aspect of Sertoli cell function (e.g., ABP), FSH stimulates synthesis of the dimeric protein inhibin. Inhibin is induced by FSH and negatively feeds back on the gonadotrope to selectively inhibit FSH production (Fig. 43-10).
THE MALE REPRODUCTIVE TRACT
Once spermatozoa emerge from the efferent ductules, they leave the gonad and enter the male reproductive tract (Fig. 43-1). The segments of the tract are as follows: the epididymis (head, body, and tail), the vas deferens, the ejaculatory duct, the prostatic urethra, the membranous urethra, and the penile urethra. Unlike the female tract, there is a contiguous lumen from the seminiferous tubule to the end of the male tract (i.e., the tip of the penile urethra), and the male reproductive tract connects to the distal urinary tract (i.e., male urethra). In addition to conveying sperm, the primary functions of the male reproductive tract are as follows:
There is an important “loophole” in the male reproductive axis that is based on the fact that intratesticular levels of testosterone need to be greater than 100-fold higher than circulating levels of the hormone to maintain normal rates of spermatogenesis; however, it is the circulating levels of testosterone that provide the negative feedback to the pituitary and hypothalamus. This means that exogenous administration of testosterone can raise circulating levels sufficient to inhibit LH but not sufficient to accumulate in the testis at the required concentration for normal spermatogenesis. However, the decreased LH levels will diminish intratesticular production of testosterone by Leydig cells, which results in reduced levels of spermatogenesis (Fig. 43-11). This “loophole” is currently being investigated as a possible strategy for developing a male oral contraceptive. It is also the basis for sterility in some cases of steroid abuse in men.
Erection is a neurovascular event. The penis is composed of three erectile bodies: two corpora cavernosa and one corpus spongiosum (Fig. 43-12, A). The penile urethra runs through the corpus spongiosum. These three bodies are composed of erectile tissue—an anastomosing network of potential cavernous vascular spaces lined with continuous endothelia within a loose connective tissue support. During the flaccid state, blood flow to the cavernous spaces is minimal (Fig. 43-12, A). This is due to vasoconstriction of the vasculature (called the helicine arteries) and shunting of blood flow away from the cavernous spaces. In response to sexual arousal, the parasympathetic cavernous nerves innervating the vascular smooth muscle of the helicine arteries release nitric oxide (NO). NO activates guanylyl cyclase, thereby increasing cGMP, which decreases intracellular [Ca++] and causes muscular relaxation (Fig. 43-12, B). The vasodilation allows blood to flow into the cavernous spaces to induce engorgement and erection. It also presses on veins in the penis and reduces venous drainage (Fig. 43-12, B).
An inability to achieve or maintain an erection is termed erectile dysfunction (ED) and is one cause of infertility. Multiple factors can lead to ED, including insufficient androgen production; neurovascular damage (e.g., from diabetes mellitus, spinal cord injury); structural damage to the penis, perineum, or pelvis; psychogenic factors (e.g., depression, performance anxiety); and prescribed medications and recreational drugs, including alcohol and tobacco. A major development in the treatment of some forms of erectile dysfunction is the use of selective cGMP phosphodiesterase inhibitors (e.g., Viagra), which assist in the maintenance of an erection (Fig. 43-12, B).
THE OVARY
The ovary is located within a fold of peritoneum called the broad ligament, usually close to the lateral wall of the pelvic cavity (Fig. 43-13). Because the ovary extends into the peritoneal cavity, ovulated eggs briefly reside within the peritoneal cavity before they are captured by the oviducts.
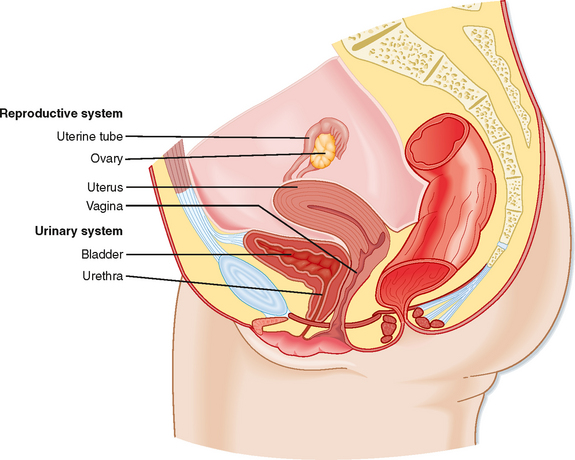
Figure 43-13 Anatomy of the female reproductive system.
(Modified from Drake RL et al: Gray’s Anatomy for Students. Philadelphia, Churchill Livingstone, 2005.)
The ovary is divided into an outer cortex and inner medulla (Fig. 43-14). Neurovascular elements innervate the medulla of the ovary. The cortex of the ovary is composed of a densely cellular stroma. Within this stroma reside the ovarian follicles (Fig. 43-14), which contain a primary oocyte surrounded by follicle cells. The cortex is covered by a connective tissue capsule, the tunica albuginea, and a layer of simple epithelium consisting of ovarian surface epithelial cells. There are no ducts emerging from the ovary to convey its gametes to the reproductive tract. Thus, the process of ovulation involves an inflammatory event that erodes the wall of the ovary. After ovulation, the ovarian surface epithelial cells rapidly divide to repair the wall.
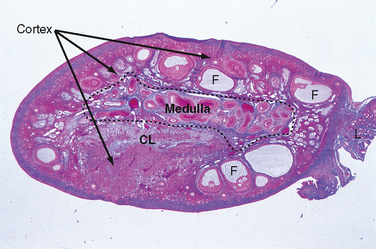
Figure 43-14 Histology of the ovary. CL, corpus luteum; F, follicle;
(Modified from Young B et al: Wheater’s Functional Histology. A Text and Colour Atlas, 5th ed. London, Churchill Livingstone, 2006.)
Growth, Development, and Function of the Ovarian Follicle
Resting Primordial Follicle
Growth and Structure.
Resting primordial follicles (Fig. 43-15) represent the earliest and simplest follicular structure in the ovary. Primordial follicles appear during midgestation through the interaction of gametes and somatic cells. Primordial germ cells that have migrated to the gonad continue to divide mitotically as oogonia until the fifth month of gestation in humans. At this point the approximately 7 million oogonia enter the process of meiosis and become primary oocytes. During this time the primary oocytes become surrounded by a simple epithelium of somatic follicle cells, thereby creating the primordial follicles (Fig. 43-15). The follicle cells establish gap junctions with each other and the oocyte. The follicle cells themselves represent a true avascular epithelium surrounded by a basal lamina. Similar to Sertoli cell–sperm interactions, a subpopulation of granulosa cells remains intimately attached to the oocytes throughout their development. Granulosa cells provide nutrients such as amino acids, nucleic acids, and pyruvate to support oocyte maturation.
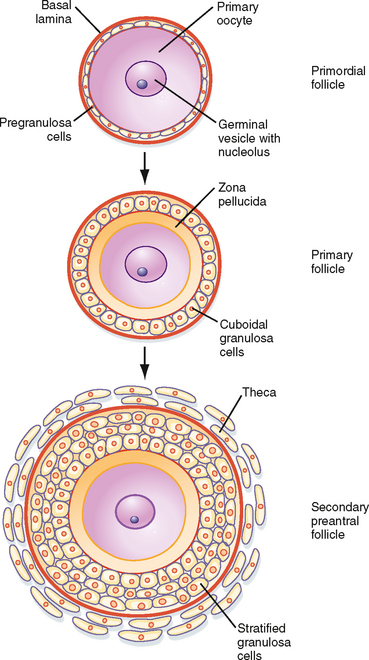
Figure 43-15 Development of a primordial follicle up to a secondary, preantral follicle.
(Modified from Porterfield SP, White BA: Endocrine Physiology, 3rd ed. Philadelphia, Mosby, 2007.)
The primordial follicles represent the ovarian reserve of follicles (Fig. 43-16). This reserve is reduced from a starting number of about 7 million to less than 300,000 follicles at reproductive maturity. Of these, a woman will ovulate about 450 between menarche (first menstrual cycle) and menopause (cessation of menstrual cycles). At menopause, less than 1000 primordial follicles are left in the ovary. Primordial follicles are lost primarily from death as a result of follicular atresia. However, a small subset of primordial follicles will enter follicular growth in waves. Because the ovarian follicular reserve represents a fixed, finite number, the rate at which resting primordial follicles die or begin to develop (or both) will determine the reproductive life span of a woman. Age at the onset of menopause has a strong genetic component but is also influenced by environmental factors. For example, cigarette smoking significantly depletes the ovarian reserve. An overly rapid rate of atresia or development will deplete the reserve and give rise to premature ovarian failure.
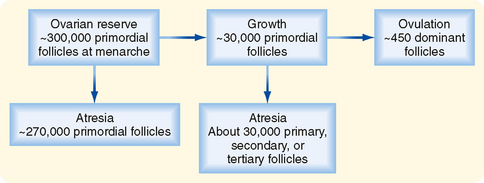
Figure 43-16 Fate of ovarian follicles.
(Modified from Porterfield SP, White BA: Endocrine Physiology, 3rd ed. Philadelphia, Mosby, 2007.)
Growing Preantral Follicles
Growth and Structure.
The first stage of follicular growth is preantral, which refers to the development that occurs before the formation of a fluid-filled antral cavity. One of the first visible signs of follicle growth is the appearance of cuboidal granulosa cells. At this point the follicle is referred to as a primary follicle (Fig. 43-15). As granulosa cells proliferate, they form a multilayered (i.e., stratified) epithelium around the oocyte. At this stage the follicle is referred to as a secondary follicle (Fig. 43-15).
Once a secondary follicle acquires three to six layers of granulosa cells, it secretes paracrine factors that induce nearby stromal cells to differentiate into epithelioid thecal cells. Thecal cells form a flattened layer of cells around the follicle. Once a thecal layer forms, the follicle is referred to as a mature preantral follicle (Fig. 43-15). In humans it takes several months for a primary follicle to reach the mature preantral stage.
The Gamete.
During the preantral stage, the oocyte begins to grow and produce cellular and secreted proteins. The oocyte initiates secretion of extracellular matrix glycoproteins, called ZP1, ZP2, and ZP3, that form the zona pellucida (Fig. 43-15). The zona pellucida increases in thickness and provides a species-specific binding site for sperm during fertilization (see later). Importantly, granulosa cells and the oocyte maintain gap junctional contact via cellular projections through the zona pellucida. The oocyte also continues to secrete paracrine factors that regulate follicle cell growth and differentiation.
Growing Antral Follicles
Growth and Structure.
Mature preantral follicles develop into early antral follicles (Fig. 43-17) over a period of about 25 days, during which they grow from a diameter of about 0.1 mm to a diameter of 0.2 mm. Once the granulosa epithelium increases to six to seven layers, fluid-filled spaces appear between cells and coalesce into the antrum. Over a period of about 45 days, this wave of small antral follicles will continue to grow to large, recruitable antral follicles that are 2 to 5 mm in diameter. This period of growth is characterized by about a 100-fold increase in granulosa cells (from about 10,000 to 1,000,000 cells). It is also characterized by swelling of the antral cavity, which increasingly divides the granulosa cells into two discrete populations (Fig. 43-17).
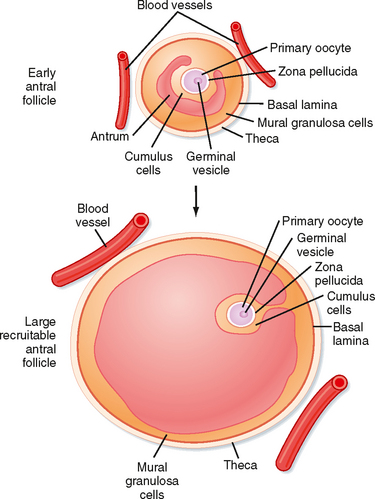
Figure 43-17 Development of an early antral follicle to a mature preovulatory follicle.
(Modified from Porterfield SP, White BA: Endocrine Physiology, 3rd ed. Philadelphia, Mosby, 2007.)
Early antral follicles are dependent on pituitary FSH for normal growth. Large antral follicles become highly dependent on pituitary FSH for their growth and sustained viability. As discussed later, 2- to 5-mm follicles are recruited to enter a rapid growth phase via the transient increase in FSH that occurs toward the end of the previous menstrual cycle.
The Gamete.
The oocyte grows rapidly in the early stages of antral follicles—growth then slows in larger follicles. During the antral stage, the oocyte synthesizes sufficient amounts of cell cycle components so that it becomes competent to complete meiosis I at ovulation (note that the human egg arrests after ovulation at a second point, metaphase II, until it is fertilized by sperm). Thus, in early primary and secondary follicles, the oocyte fails to complete meiosis I because of a dearth of specific meiosis-associated proteins. However, larger antral follicles gain meiotic competence but still maintain meiotic arrest until the midcycle LH surge. Meiotic arrest is achieved by maintenance of elevated cAMP levels in the mature oocyte (Figs. 43-18 and 43-19).
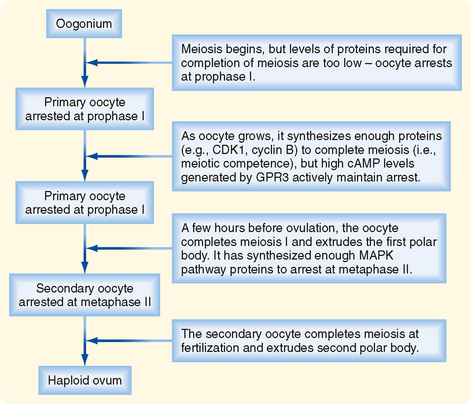
Figure 43-18 Events involved in meiotic arrest and maturation of the oocyte. MAPK, mitogen-activated protein kinase.
(Modified from Porterfield SP, White BA: Endocrine Physiology, 3rd ed. Philadelphia, Mosby, 2007.)
Dominant Follicle
Growth and Structure.
At the end of a previous menstrual cycle, a crop of large (2 to 5 mm) antral follicles (Fig. 43-17) are recruited to begin rapid, gonadotropin-dependent development. The total number of recruited follicles in both ovaries can be as high as 20 in a younger woman (<33 years old) but rapidly declines at older ages. The number of recruited follicles is reduced to the prolifera quota (one in humans) by the process of selection. As FSH levels decline, the rapidly growing follicles progressively undergo atresia until one follicle is left. Generally, the largest follicle with the most FSH receptors of the recruited crop becomes the dominant follicle. Selection occurs during the early follicular phase. By midcycle, the dominant follicle becomes a large preovulatory follicle that is 20 mm in diameter and contains about 50 million granulosa cells by the midcycle gonadotropin surge.
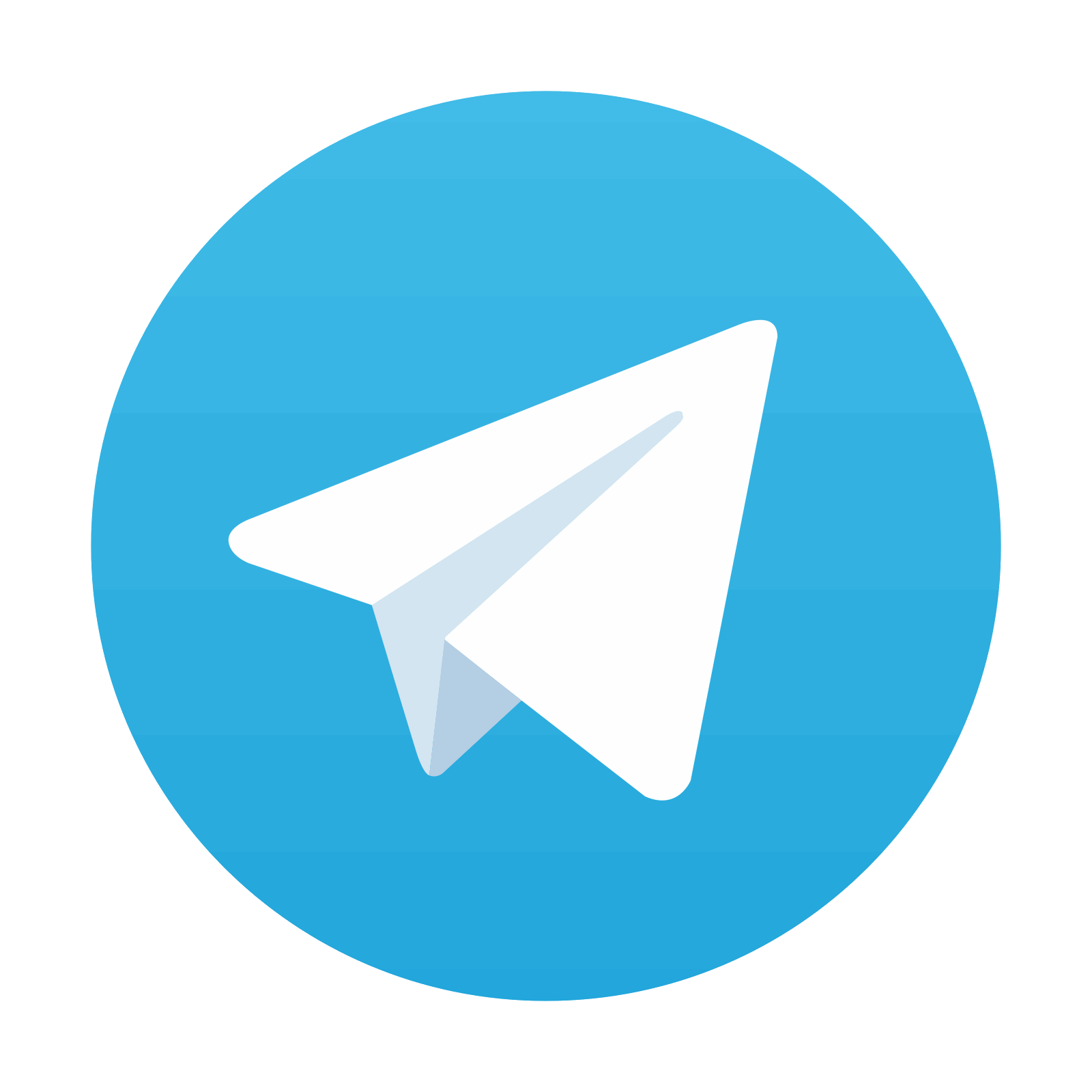
Stay updated, free articles. Join our Telegram channel

Full access? Get Clinical Tree
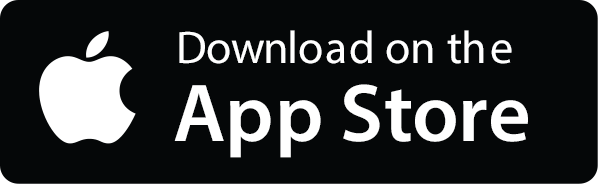
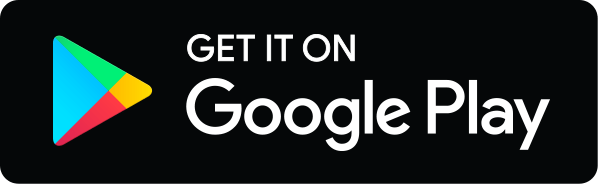