The Fed State. During a meal, we ingest carbohydrates, lipids, and proteins, which are subsequently digested and absorbed. Some of this food is oxidized to meet the immediate energy needs of the body. The amount consumed in excess of the body’s energy needs is transported to the fuel depots, where it is stored. During the period from the start of absorption until absorption is completed, we are in the fed, or absorptive, state. Whether a fuel is oxidized or stored in the fed state is determined principally by the concentration of two endocrine hormones in the blood, insulin and glucagon.
Fate of Carbohydrates. Dietary carbohydrates are digested into monosaccharides, which are absorbed into the blood. The major monosaccharide in the blood is glucose (Fig. 2.1). After a meal, glucose is oxidized by various tissues for energy, enters biosynthetic pathways, and is stored as glycogen, mainly in the liver and muscle. Glucose is the major biosynthetic precursor in the body, and the carbon skeletons of most of the compounds we synthesize can be synthesized from glucose. Glucose is also converted to triacylglycerols. The liver packages triacylglycerols, made from glucose or from fatty acids obtained from the blood, into very-low-density lipoproteins (VLDL) and releases them into the blood. The fatty acids of the VLDL are stored mainly as triacylglycerols in adipose tissue, but some may be used to meet the energy needs of cells.
FIGURE 2.1 Major fates of fuels in the fed state. TG, triacylglycerol.
Fate of Proteins. Dietary proteins are digested into amino acids, which are absorbed into the blood. In cells, the amino acids are converted to proteins or used to make various nitrogen-containing compounds such as neurotransmitters and heme. The carbon skeleton may also be oxidized for energy directly or can be converted to glucose for storage as glycogen.
Fate of Fats. Triacylglycerols are the major lipids in the diet. They are digested into fatty acids and 2-monoacylglycerols, which are resynthesized into triacylglycerols in intestinal epithelial cells, packaged in chylomicrons, and secreted by way of the lymph into the blood. The fatty acids of the chylomicron triacylglycerols are stored mainly as triacylglycerols in adipose cells. They are subsequently oxidized for energy or used in biosynthetic pathways, such as the synthesis of membrane lipids.
THE WAITING ROOM 
Ivan A. returned to his doctor for a second visit. His initial efforts to lose weight and follow the dietitian’s recommendations had failed dismally. In fact, he now weighs 270 lb, an increase of 6 lb since his first visit 2 months ago (see Chapter 1). He reported that the recent death of his 45-year-old brother of a heart attack had made him realize that he must pay more attention to his health. Because Ivan A.’s brother had a history of hyperlipidemia and because Ivan A.’s serum total cholesterol was significantly elevated (296 mg/dL) at his first visit, his blood lipid profile was determined, he was screened for type 2 diabetes, and a few other blood tests were ordered. (The blood lipid profile is a measure of the content of the various triacylglycerol- and cholesterol-containing particles in the blood.) His systolic blood pressure was 162 mm Hg and diastolic blood pressure 98 mm Hg, or 162/98 mm Hg (normal ≤ 120/80 mm Hg or less, with elevated blood pressure 120 to 129/<80, and hypertension defined as >130/80). His waist circumference was 48 in (healthy values for men, <40 in; for women, <35 in).
I. Digestion and Absorption
A. Carbohydrates
Dietary carbohydrates are converted to monosaccharides. Starch, a polymer of glucose, is the major carbohydrate of the diet. It is digested by the enzyme salivary α-amylase, and then by pancreatic α-amylase, which acts in the small intestine. Enzymes are proteins that catalyze biochemical reactions (usually they increase the speed at which the reactions occur). Di-, tri-, and oligosaccharides (disaccharide refers to two linked sugars; a trisaccharide to three linked sugars, and an oligosaccharide to n linked sugars) produced by these α-amylases are cleaved to glucose by digestive enzymes located on the surface of the brush border of the intestinal epithelial cells. Dietary disaccharides also are cleaved by enzymes at the brush border. Sucrase converts the disaccharide sucrose (table sugar) to glucose and fructose, and lactase converts the disaccharide lactose (milk sugar) to glucose and galactose. Monosaccharides produced by digestion and dietary monosaccharides are absorbed by the intestinal epithelial cells via transporters and are released into the hepatic portal vein, which carries them to the liver.
B. Proteins
Proteins contain amino acids that are linked through peptide bonds (see Chapter 1). Dipeptides contain two amino acids, tripeptides contain three amino acids, and so on. Dietary proteins are cleaved to amino acids by enzymes known as proteases (Fig. 2.2, circle 3), which cleave the peptide bond between amino acids (see Fig. 1.5). Pepsin acts in the stomach, and the proteolytic enzymes produced by the pancreas (trypsin, chymotrypsin, elastase, and the carboxypeptidases) act in the lumen of the small intestine. Aminopeptidases and di- and tripeptidases associated with the intestinal epithelial cells complete the conversion of dietary proteins to amino acids, which are absorbed into the intestinal epithelial cells and released into the hepatic portal vein.
FIGURE 2.2 The fed state. The circled numbers indicate the approximate order in which the processes occur. AA, amino acid; Acetyl CoA, acetyl coenzyme A; ATP, adenosine triphosphate; CHO, carbohydrate; FA, fatty acid; I, insulin; RBC, red blood cell; TCA, tricarboxylic acid; TG, triacylglycerols; VLDL, very-low-density lipoprotein; ⊕, stimulated by.
C. Fats
The digestion of fats is more complex than that of carbohydrates or proteins because fats are not very soluble in water. The triacylglycerols of the diet are emulsified in the intestine by bile salts, which are synthesized in the liver, stored in the gallbladder, and released. The enzyme pancreatic lipase converts the triacylglycerols in the lumen of the intestine to fatty acids and 2-monoacylglycerols (glycerol with a fatty acid esterified at carbon 2), which interact with bile salts to form tiny microdroplets called micelles. The fatty acids and 2-monoacylglycerols are absorbed from these micelles into intestinal epithelial cells, where they are resynthesized into triacylglycerols. The triacylglycerols are packaged with proteins, phospholipids, cholesterol, and other compounds into lipoprotein complexes known as chylomicrons, which are secreted into the lymph and ultimately enter the bloodstream (see Fig. 2.2, circle 2). Fats must be transported in the blood bound to protein or lipoprotein complexes because they are insoluble in water. Thus, both triacylglycerols and cholesterol are found in lipoprotein complexes.
II. Changes in Hormone Levels after a Meal
After a typical meal, the pancreas is stimulated to release the hormone insulin, and the release of the hormone glucagon is inhibited (see Fig. 2.2, circle 4). Endocrine hormones are released from endocrine glands, such as the pancreas, in response to a specific stimulus. They travel in the blood, carrying messages between tissues concerning the overall physiologic state of the body. At their target tissues, they adjust the rate of various metabolic pathways to meet the changing conditions. The endocrine hormone insulin, which is secreted from the β-cells of the pancreas in response to a meal, carries the message that dietary glucose is available and can be transported into the cell, utilized and stored. The release of another hormone, glucagon, from the α-cells of the pancreas, is suppressed by glucose and insulin. Glucagon carries the message that glucose must be generated from endogenous fuel stores. The subsequent changes in circulating hormone levels cause changes in the body’s metabolic patterns, involving a number of different tissues and metabolic pathways.
III. Fate of Glucose
A. Conversion to Glycogen, Triacylglycerols, and CO2 in the Liver
Because glucose leaves the intestine via the hepatic portal vein (which carries blood from the intestine to the liver), the liver is the first tissue it passes through. The liver extracts a portion of this glucose from the blood. Some of the glucose that enters hepatocytes (liver cells) is oxidized in adenosine triphosphate (ATP)-generating pathways to meet the immediate energy needs of these cells, and the remainder is converted to glycogen and triacylglycerols or used for biosynthetic reactions. In the liver, insulin stimulates the utilization of glucose by increasing its use as a fuel and its storage as glycogen and triacylglycerols (see Fig. 2.2, circles 5 to 7).
To generate energy and metabolic precursors, glucose is first oxidized to pyruvate in the glycolysis pathway, which consists of a series of reactions common to the metabolism of many carbohydrates (discussed in more detail in Chapter 22). Pyruvate is then oxidized to acetyl coenzyme A (acetyl CoA). The acetyl group enters the tricarboxylic acid (TCA) cycle, where it is completely oxidized to CO2. Energy from the oxidative reactions is used to generate ATP. The ATP that is generated is used for anabolic pathways and other energy-requiring processes in the cell. Coenzyme A (CoA), which makes the acetyl group more reactive, is a cofactor derived from the vitamin pantothenic acid.
Liver glycogen stores reach a maximum of approximately 200 to 300 g after a high-carbohydrate meal, whereas the body’s fat stores are relatively limitless. As the glycogen stores begin to fill, the liver also begins converting some of the excess glucose it receives to triacylglycerols. Both the glycerol and the fatty acid moieties of the triacylglycerols can be synthesized from glucose. The fatty acids are also obtained preformed from the blood (dietary fatty acids). The liver does not store triacylglycerols, however, but packages them along with proteins, phospholipids, and cholesterol into the lipoprotein complexes known as very-low-density lipoproteins (VLDL), which are secreted into the bloodstream. Some of the fatty acids from the VLDL are taken up by tissues for their immediate energy needs, but most are stored in adipose tissue as triacylglycerols.
B. Glucose Metabolism in Other Tissues
Dietary glucose that is not taken up and metabolized by the liver travels in the blood to peripheral tissues (most other tissues), where it can be oxidized for energy. Glucose is the one fuel that can be utilized by cells of all tissues. Many tissues store small amounts of glucose as glycogen. Collectively, muscle has the largest body glycogen stores.
Insulin greatly stimulates the transport of glucose into the two tissues that have the largest mass in the body, muscle and adipose tissue. It has much smaller effects on the transport of glucose into other tissues.
Fuel metabolism is often discussed as though the body consisted only of brain, skeletal and cardiac muscle, liver, adipose tissue, red blood cells, kidney, and intestinal epithelial cells (“the gut”). These are the dominant tissues in terms of overall fuel economy, and they are the tissues we describe most often. However, all tissues require fuels for energy, and many have very specific fuel requirements.
1. Brain and Other Neural Tissues
The brain and other neural tissues are very dependent on glucose for their energy needs. Neurons generally oxidize glucose, via glycolysis and the TCA cycle, completely to CO2 and H2O, generating ATP (see Fig. 2.2, circle 8). Except under conditions of starvation, glucose is their only major fuel. Glucose is also a major precursor of neurotransmitters, the chemicals that convey electrical impulses (as ion gradients) between neurons. If our blood glucose drops much below normal levels, we become dizzy and light-headed. If blood glucose continues to drop, we become comatose and ultimately die. Under normal, nonstarving conditions, the brain and the rest of the nervous system require roughly 150 g of glucose each day.
2. Red Blood Cells
Glucose is the only fuel used by red blood cells because they lack mitochondria. Fatty acid oxidation, amino acid oxidation, the TCA cycle, the electron-transport chain, and oxidative phosphorylation (ATP generation that is dependent on oxygen and the electron-transport chain) occur principally in mitochondria. Glucose, in contrast, generates ATP from anaerobic glycolysis in the cytoplasm, and thus red blood cells obtain all their energy by this process. In anaerobic glycolysis, the pyruvate formed from glucose is reduced to lactate and then released into the blood (see Fig. 2.2, circle 9).
Without glucose, red blood cells could not survive. Red blood cells carry O2 from the lungs to the tissues. Without red blood cells, most of the tissues of the body would suffer from a lack of energy because they require O2 to completely convert their fuels to CO2 and H2O.
3. Muscle
Exercising skeletal muscles can use glucose from the blood or from their own glycogen stores, converting glucose to lactate through glycolysis or oxidizing it completely to CO2 and H2O. Muscle also uses other fuels from the blood, such as fatty acids (Fig. 2.3). Resting or lightly exercising muscle cells mostly use fatty acids as fuel. After a meal, glucose is used by muscles to replenish the glycogen stores that were depleted during exercise. Glucose is transported into muscle cells and converted to glycogen by processes that are stimulated by insulin.
FIGURE 2.3 Oxidation of fuels in exercising skeletal muscle. Exercising muscle uses more energy than resting muscle; therefore, fuel utilization is increased to supply more ATP. Acetyl CoA, acetyl coenzyme A; TCA, tricarboxylic acid.
4. Adipose Tissue
Insulin stimulates the transport of glucose into adipose cells as well as into muscle cells. Adipocytes oxidize glucose for energy, and they also use glucose as the source of the glycerol moiety of the triacylglycerols they store (see Fig. 2.2, circle 10).
IV. Lipoproteins
Two types of lipoproteins, chylomicrons and VLDL, are produced in the fed state. The major function of these lipoproteins is to provide a blood transport system for triacylglycerols, which are very insoluble in water. However, these lipoproteins also contain the lipid cholesterol, which is also somewhat insoluble in water. The triacylglycerols of chylomicrons are formed in intestinal epithelial cells from the products of digestion of dietary triacylglycerols. The triacylglycerols of VLDL are synthesized in the liver.
When these lipoproteins pass through blood vessels in adipose tissue, their triacylglycerols are degraded to fatty acids and glycerol (see Fig. 2.2, circle 12). The fatty acids enter the adipose cells and combine with a glycerol moiety that is produced from blood glucose. The resulting triacylglycerols are stored as large fat droplets in the adipose cells. The remnants of the chylomicrons are cleared from the blood by the liver. The remnants of the VLDL can be cleared by the liver, or they can form low-density lipoprotein (LDL), which is cleared by the liver or by peripheral cells.
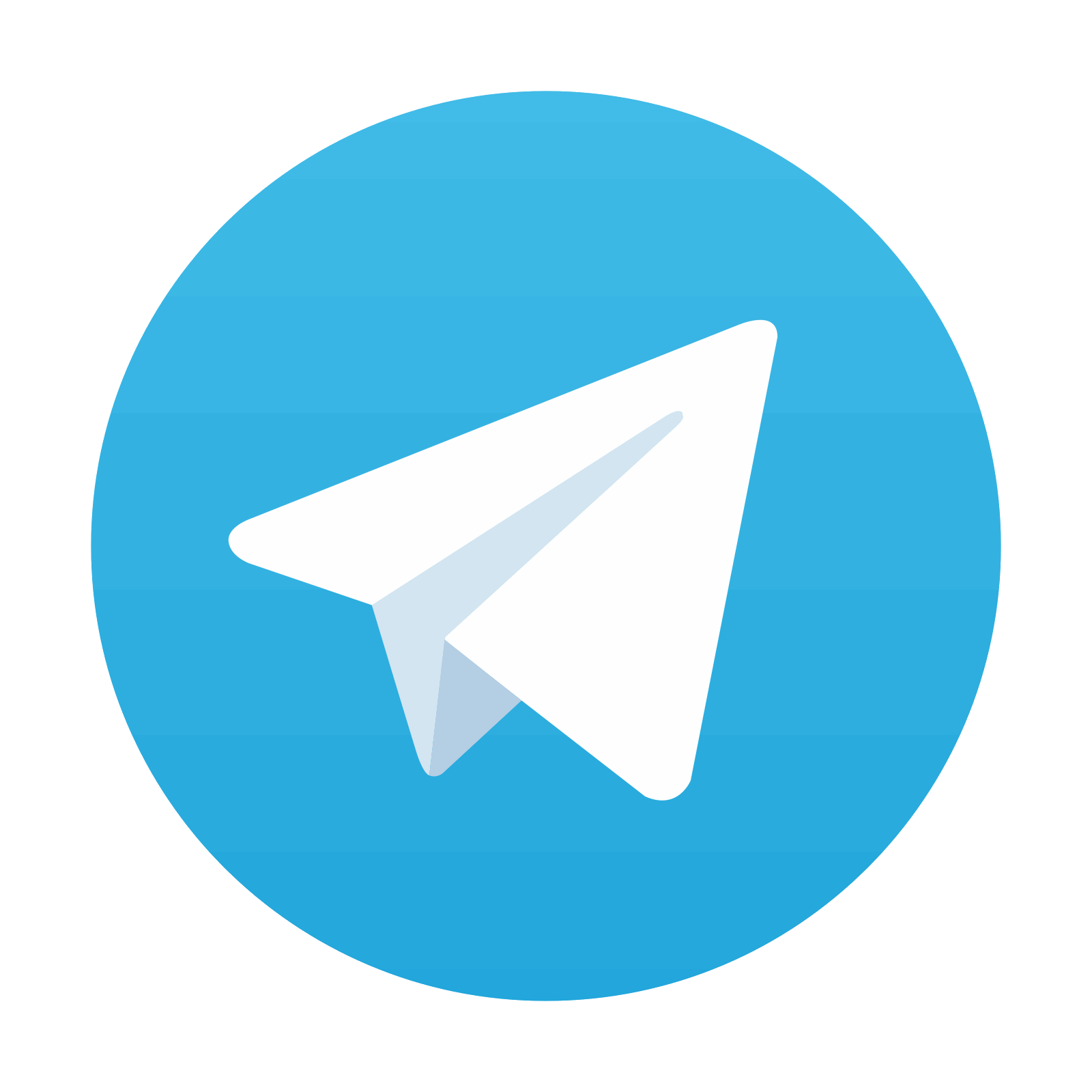
Stay updated, free articles. Join our Telegram channel

Full access? Get Clinical Tree
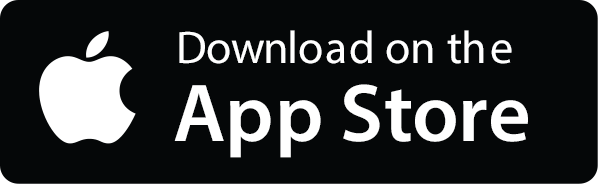
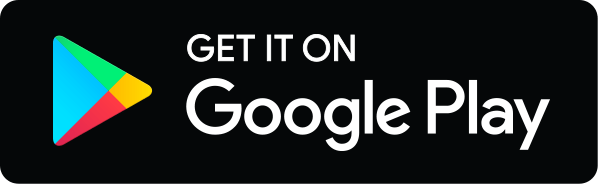