Fig. 11.1
Flow diagram for the workup of a woman with delayed puberty (Courtesy of Dr. Lawrence Layman.) PCOS polycystic ovary syndrome, CAIS complete androgen insensitivity syndrome, FSH follicle-stimulating hormone, LH luteinizing hormone, Asherman syndrome adhesions from prior uterine curettage
Cytogenetic Findings in Female Infertility Due to Ovarian Dysfunction
45,X and 45,X Mosaicism
As discussed in Chap. 10, one in about 2,500 baby girls is born with 45,X or a mosaic variant thereof. Ninety percent of women with 45,X or with 45,X mosaicism with 46,XY, 46,XX, 47,XXX, or 46,X,i(Xq) cell lines present with primary amenorrhea and lack of pubertal development. Up to 25% have some breast development [3]. Two to 3% of 45,X women and 10–15% of women with mosaic 45,X experience normal pubertal development and menarche but are highly likely to undergo secondary amenorrhea [3, 4].
Of those who undergo menarche, their only manifestation of a sex chromosome abnormality may be short stature. Thus, blood chromosome analysis is recommended when a woman has short stature and reproductive failure.
More than 100 pregnancies have been reported in women with a 45,X cell line who did not use assisted reproductive technologies (ART) such as in vitro fertilization (IVF). Kaneko et al. reviewed the literature on 138 pregnancies in 62 women with a 45,X cell line, many of whom had a second cell line [5]. Thirteen 45,X patients had 21 pregnancies, 22 45,X/46,XX women had 50 pregnancies, 12 patients with 45,X/47,XXX had 20 pregnancies, 44 pregnancies were from 14 patients with 45,X/46,XX/47,XXX, and one patient with 45,X/46,XX/47,XXX/48,XXXX had three pregnancies. None was reported in women with a 46,XY cell line without IVF.
Among 13 women with 45,X without mosaicism, six of 21 pregnancies ended in spontaneous abortions, and two of 21 ended in stillbirth, including one with hydrocephalus. Another three culminated in a pregnancy and/or offspring with a chromosome abnormality or significant birth defect (one with Down syndrome, one with 45,X, one with a partial cleft of the soft palate).
Among the liveborn for 45,X and mosaic 45,X patients, 23/102 (23%) had chromosome abnormalities or birth defects. For 16 others in the mosaic group, no outcome information was available. Ten percent of liveborn of pregnant women with a 45,X mosaic cell line had a female child with ovarian failure with reported karyotypes in their offspring including 45,X (3%), 45,X/46,XX (4%), 45,X/46,XY (1%), and 45,X/46,XX/47,XXX (2%). From these data, it appears that nonmosaic 45,X live-born offspring are less likely to have abnormalities than live-born offspring of women with mosaic 45,X. In this group, 12 nonmosaic 45,X women’s live born infants had no abnormalities.
Magee et al. described a patient with nonmosaic 45,X, based on several tissue studies, who appeared to have had seven pregnancies. Three of them were confirmed. These three culminated in a missed abortion, a fetus with 45,X that was terminated, and a healthy baby boy [6].
The approximately 30% incidence of fetal loss and other abnormal outcomes among offspring of 45,X mosaic and nonmosaic women should be stressed when providing genetic counseling to these patients, and prenatal diagnosis by chorionic villus sampling or amniocentesis is indicated.
Women with 45,X and 45,X Mosaicism and In Vitro Fertilization
Pregnancy rates using ovum donors in centers specializing in in vitro fertilization report pregnancy rates of 50–60%, with the endometrial response to estrogen treatment not significantly different from that of women with secondary ovarian failure [3]. Cardiovascular and kidney functions are to be assessed prior to instituting a pregnancy in these patients, given the high baseline risks of heart and kidney abnormalities in women with 45,X and mosaic 45,X.
Detection of Y Chromosome Sequences in 45,X and Mosaic 45,X Patients
Among the hypotheses as to why all but one percent of 45,X fetuses die in utero and why some women with apparent nonmosaic 45,X have some fertility is that 45,X individuals may actually be cryptic mosaics for another cell line that supports survival. It is important to consider too that the detection of mosaicism is limited by the numbers of tissues and cells examined. Sometimes, mosaicism is inferred by cytogenetic findings in the offspring. In one such case, described by Magee et al., a woman with 45,X had two pregnancies—one ending in spontaneous abortion at 8 weeks of gestation and the other resulting in a female with 46,X,del(X)(p21) [6]. In another case, a woman with apparent nonmosaic 45,X had a baby girl with 46,X,der(X). Using fluorescence in situ hybridization (FISH), one cell of 450 examined in maternal lymphocytes showed a der(X). Kocova et al. note in their paper describing Y chromosome sequences in Turner syndrome that when others evaluated both peripheral lymphocytes and fibroblasts, only about 21% of karyotypes of 87 live-born Turner syndrome patients were found to be 45,X. Kocova’s group evaluated 18 females with nonmosaic Turner syndrome by performing chromosome analysis on blood and/or skin fibroblasts. In six of these patients, presence of the SRY (testis-determining factor, or gene) was detected [7].
X Chromosome Deletions
X chromosome deletions are usually sporadic, although familial cases have been reported. Deletions affecting the short arm of the X chromosome at band p11 result in ovarian failure in about half of women, and the other half experience menstrual irregularities. Fertility is rare even if menstruation occurs. If the deletion occurs more distally, such as at band p21, patients usually have a milder phenotype with normal menarche, even though secondary amenorrhea or infertility is common. Most women with Xp deletions are short, even if ovarian function is normal.
Deletions of the long arm of the X chromosome generally are associated with ovarian failure if they involve the so-called critical region—the region between Xq13 and Xq26. As with deletions of the short arm, more distal Xq deletions are associated with a milder phenotype. These women may have menarche with or without ovarian failure. Women with deletions in Xq13 have primary amenorrhea, no breast development, and ovarian failure with high levels of FSH and LH. Davison et al. performed cytogenetic analyses on 79 women with primary or secondary amenorrhea, and two of the 79 had an abnormal karyotype. One of them was a woman with primary amenorrhea and a 46,XY karyotype. The other was a woman with secondary amenorrhea and a deletion at Xq26.1. This woman had a family history of premature ovarian failure, and her mother, who had undergone premature ovarian failure at 28 years, also had this deletion [8]. See Fig. 11.2, which shows locations of different deletions of the X chromosome and the associated phenotype. See also Chap. 10.


Fig. 11.2
An idiogram of the X chromosome with locations of various deletions and the corresponding clinical characteristics (Reprinted with permission from Simpson and Rajkovic [9])
X Chromosome; Autosome Translocations
In a balanced X-autosome translocation, the normal X is generally inactivated. If the abnormal X were inactivated, autosomal material would be inactivated along with it. Inactivation of autosome genes would probably be a lethal event.
In an unbalanced X-autosome translocation, the normal X chromosome remains active, while the abnormal X is inactivated in an attempt to compensate for the imbalance.
Translocations involving the X chromosome and an autosome are rare, occurring in one in about 30,000 live births [4]. This relates in part to the fact that all males and half of females with this finding are infertile. For women, the phenotypic effects depend on the breakpoint and the status of inactivation of the X chromosomes. If the derivative X is active in all cells and the breakpoint does not interrupt a functional gene, about half have a normal phenotype and half have ovarian failure. In general, those with ovarian failure have breakpoints within the Xq13–26 region.
For women with an active derivative X, when the breakpoints interrupt important genes on either the X or the autosome, a single-gene disorder, such as Duchenne muscular dystrophy, may result. When the derivative X is active in only a portion of cells, multiple anomalies and mental retardation usually result.
The breakpoints on the X chromosome vary widely in X-autosome translocations. The most common autosomes involved include chromosomes 15, 21, and 22. The pericentromeric regions of these chromosomes are predisposed to pairing with the X chromosome.
For non-cytogenetic-inherited causes of ovarian dysfunction, please see Table 11.1.
Gene | Locus | Phenotype | Inheritance |
FMR1 | Xq27.3 | Fragile X syndrome; ovarian failure | X-linked dominant |
SRY | Yp11.3 | Swyer syndrome; sexual infantilism, normal vagina and uterus, streak gonads with risk for gonadoblastoma +/− germ cell tumor | Sporadic; Y-linked |
FSHR | 2p21-p16 | Primary amenorrhea, half with breast development; men have oligospermia | Autosomal recessive |
LHCGR | 2p21 | Anovulation in women; undermasculinization in men | Autosomal recessive |
CYP17A1 | 10q24.3 | 17-hydroxylase deficiency; delayed puberty in women, absent breast development, primary amenorrhea, and elevated gonadotropins | Autosomal recessive |
CYP19A1 | 15q21.1 | Aromatase deficiency; cannot convert androgens to estrogens; females with sexual ambiguity, clitoromegaly; no breast development or menses | Autosomal recessive |
AIRE | 21q22.3 | Autoimmune polyendocrinopathy-candidiasis-ectodermal dystrophy (APECED); multisystem autoimmune disease; adrenal, ovarian, and testicular failure may occur | Autosomal recessive |
NR5A1 | 9q33 | Steroidogenic factor 1; adrenal failure, sex reversal in men; presents as female phenotype; rare | Autosomal recessive |
GALT | 9p13 | Galactose-1-phosplate uridytransferase; causes galactosemia; normal puberty; half with primary and half with secondary amenorrhea; 67% of women have ovarian failure and testicular function apparently not affected. Heterozygotes (carriers) not affected | Autosomal recessive |
Endometriosis
Endometriosis is a common disorder that accounts for infertility in 6–10% of women of reproductive age. It is characterized by the formation of collections of endometrial tissue outside of the uterus in so-called chocolate cysts. These cysts occur in the ovary and elsewhere in the pelvis and body. The name of the cysts is due to the appearance of chocolate syrup within the cysts. Endometriosis causes painful menses as well as infertility and has been reported in sisters fairly often [10, 11].
Endometriosis is characterized by monoclonal growth and can exhibit features of malignant behavior, including local invasion and metastasis. Comparative genomic hybridization (CGH), a molecular cytogenetic method that facilitates screening of the entire genome for chromosome gains and/or losses (see Chap. 17), showed recurrent copy number losses on several chromosomes in 15 of 18 cases of endometrial tissue. Losses of 1p and 22q were each detected in half of the cases. Chromosome 7p was lost in one-fifth of the cases. These results were validated by selective dual-color FISH and were interpreted as indicating that genes localized to certain chromosome regions play a role in the development and progression of endometriosis [12]. A subsequent case–control study showed that gene variants on chromosome 7 and chromosome 1 were found to be associated with endometriosis [11].
Hypothalamic and Pituitary Causes of Female Infertility
Several genes have been identified as the cause of infertility involving malfunction of the hypothalamus or pituitary gland. Because no cytogenetic testing is helpful in these cases, a summary of gene-level conditions associated with hypothalamic malfunction is provided in Table 11.2. Table 11.3 indicates gene-level conditions associated with pituitary malfunction, and Table 11.4 lists gene-level conditions associated with uterine development abnormalities.
Gene | Locus | Phenotype | Inheritance |
---|---|---|---|
KAL | Xp22.32 | Kallmann syndrome; hypogonadotropic hypogonadism, anosmia; affects only males | X-linked recessive |
NR0B1 | Xp21.3 | Adrenal hypoplasia; congenital and hypogonadotropic hypogonadism | X-linked recessive |
LEP | 7q31.3 | Obesity, hypogonadotropic hypogonadism; delayed puberty | Autosomal recessive |
LEPR | 1p31 | Obesity, hypogonadotropic hypogonadism, and elevated serum leptin | Autosomal recessive |
Gene | Locus | Phenotype | Inheritance |
---|---|---|---|
GNRHR | 4q21.2 | Hypothalamic hypogonadism | Autosomal recessive |
HESX1 | 3p14.3 | Septo-optic dysplasia | Autosomal recessive |
LHB | 19q13.3 | Isolated luteinizing hormone deficiency | Autosomal recessive |
FSHB | 11p13 | Isolated follicle-stimulating hormone deficiency | Autosomal recessive |
PROP1 | 5q35.3 | Short stature, hypothyroidism, and hypogonadotropic hypogonadism | Autosomal recessive |
Gene | Locus | Phenotype | Inheritance |
---|---|---|---|
AR | Xq12 | Androgen insensitivity syndrome (male, 46,XY, phenotypic female) | X-linked recessive |
HOXA13 | 7p15.2 | Hand-foot-uterus syndrome | Autosomal dominant |
Causes of Male Infertility
A standardized approach to the evaluation of an infertile man was published by the World Health Organization (WHO) in 2004 [13]. This manual delineates several diagnostic categories for male infertility, including acquired and idiopathic causes. Among genetic causes are chromosome disorders, genetic disorders that affect reproduction only, and genetic disorders with other effects which also are associated with infertility. Although many advances have been made in the field of male factor infertility, it is estimated that the cause of about 30% of male infertility is still not known [14]. This chapter will provide an overview of causes but provide detail only on cytogenetic and molecular cytogenetic causes.
The SRY Gene and Genetic Sex
The presence of the SRY (sex-determining region Y) gene on the short arm of the Y chromosome induces differentiation of precursor cells into Sertoli cells, which express anti-Müllerian hormone. Anti-Müllerian hormone, which is also known as Müllerian-inhibiting substance, causes regression of Müllerian structures—the fallopian tubes, uterus, and upper vagina—and the production of testosterone in the Leydig cells. The Leydig cells are thought to differentiate because of messages from the Sertoli cells. Testosterone leads to the formation of internal male genitalia, such as epididymis, vas deferens, seminal vesicles, and ejaculatory duct. The production of dihydrotestosterone results in the formation of the penis, testes, prostate gland, and urethra. Secretion of insulin-like hormone 3 by the Leydig cells causes the descent of the testes [15].
About 10% of infertile men have severe defects in sperm production, and it is in this group of men that many of the cytogenetic and genetic disorders are concentrated [16]. Hackstein et al. note that in the fruit fly Drosophila, there is evidence that up to 1,500 genes contribute to male fertility [17]. Much more work remains to be done in humans, in whom several genes have been found to be involved in early sexual development, but many remain to be discovered. In this chapter, cytogenetic and molecular cytogenetic causes of male infertility will be discussed. See Table 11.5 for information on other genetic causes of male infertility.
Table 11.5
Percentage of certain cytogenetic or microdeletion findings in various studies of men with infertility
Cytogenetic finding | % of men with chromosome abnormality | Sperm count | Motility | Morphology |
---|---|---|---|---|
Robertsonian translocations | 6a; 0.7b; 1.6c | ≤5.6 × 106/mLa; 0.8–12 × 106/mLb; 1 of 15 with 0 and 14 of 15 with <106 per mLc | Normal to very lowa; NSb; 13 of 15 with OA or OATc | Poora; NSb; 11 of 15 with OATc |
Reciprocal translocation | 0.97a; 0.3b; 0.94c | 0a; 0–1 × 106/mLb; 4 of 9 with 0 spermc | 5 of 9 with OA or OATc | 2 of 9 with OATc |
46,X,del(Y)(q11q22); del(Yq)b; 46,X,del(Y)(q12)c | 1.9a; 2.1b; 0.21c | 0a; 0 in 7 of 17b; “oligo” in 10 of 17b; <106 per mLc | 2 of 2 with OATc | 2 of 2 with OATc |
47,XXY | 2.5b; 5.4c | 0a; 0 in 49 of 52c; <106 per mL in 3 of 52c | 3 of 52 with oligo onlyc | 3 of 52 with oligo onlyc |
47,XYY;46,XY/47,XXY; 47,XXY/48,XXXY;46,XX; 45,X/46,XY | 0.8b; 1.0c | 0 | – | – |
46,XY,inv(1)—pericentric | 0.97a; 0.1c | 0a | OAc | – |
46,XY,inv(9)(p23q33) | 0.1b | 6 × 106 per mLb | ||
Other pericentric inversions: Two with inv(2)(p11q13), one inv(3)(p13q25), one inv(5)(p13q13), and one 46,X,inv(Y)(p11q11) | Together, 0.4c | <106 per mL for all but inv(Y), which had 0 sperm countc | inv(1) and inv(3), OAc | inv(2) and inv(5), OATc |
46,XY,inv(9)(p11q12) | 2.7b | 0 in 9 of 22b; “oligo” in 13 of 22b | – | – |
Variants: Yqh+, 21 ps+, 22 ps+, 1qh+, 13 ps+, 14 ps+, 15 ps+, 16qh+ | 7.2b | 0 in 35 of 78b; “oligo” in 43 of 78b | – | – |
46,XY | – | 0a | 0a | – |
In general, men with infertility and a normal semen analysis are less likely to have a cytogenetic or molecular cytogenetic basis for their infertility. However, men with normal spermatozoa concentrations but whose spermatozoa do not fertilize also have an increased risk of a constitutional chromosome abnormality. In a study of 400 men who were to undergo intracytoplasmic sperm injection (ICSI), 6.1% of the azoospermic men and 2.7% of the oligospermic men were found to have constitutional chromosome abnormalities, and 7.4% of the men with normospermic analysis also had constitutional cytogenetic abnormalities [20].
Semen Analysis
Semen analysis is usually performed on a sample that has been ejaculated into a specimen cup. The volume and pH of the semen are measured, and the concentration, morphology, and motility of the spermatozoa are analyzed under a microscope. Cellular debris is examined to determine whether an infection is present, and fructose is measured as an indicator of obstruction. Spermatozoa counts are designated as the number present per mL. A normal number as defined by WHO is 20 × 106 sperm per mL of semen [13]. However, in a study of 430 couples in Denmark having unprotected sex, the probability of conception increased with increasing spermatozoa concentration to 40 × 106 per mL. Above that level, there was no additional likelihood of pregnancy. The authors suggested that the WHO guidelines should be used with caution, as some men above the normal range may be subfertile [21].
Oligospermia, Non-obstructive Azoospermia, and Teratozoospermia
Oligospermia, also called oligozoospermia, is defined as having a low spermatozoa count in an ejaculate. Azoospermia is the absence of spermatozoa, and teratozoospermia indicates abnormally formed spermatozoa. The concentration, morphology, and motility of spermatozoa are important factors in achieving conception.
Gunduz et al. performed chromosome analysis on 41 men with azoospermia and 61 men with oligospermia. Fourteen of the azoospermic men (34.1%), and two of the oligospermic men (3.3%), had a constitutional chromosome abnormality. The most common abnormality was 47,XXY [22].
In a review of the chromosomal contribution to male infertility, Van Assche et al. reported on the chromosome constitution of about 8,000 infertile men and compared the findings to the chromosome constitution of a group of newborn children. In the infertile group, the incidence of sex chromosome abnormalities was 27 times higher (3.8 vs. 0.13%), and the incidence of autosome abnormalities was five times higher (1.3 vs. 0.25%) [23]. When considering men with oligospermia only, pooled data show a frequency of chromosome abnormalities of 4.6%. For men with azoospermia, the pooled data show a frequency of 13.7% [23].
In a separate cytogenetic study of 1,007 infertile men, major chromosome abnormalities were seen in 62 (6.2%). Of those, 38 (3.8%) had sex chromosome abnormalities, and 24 (2.4%) had autosomal chromosome abnormalities. Of those with sex chromosome abnormalities, 28 were 47,XXY, three were 47,XYY, and seven had a Y chromosome with a structural abnormality. Of the autosomal abnormalities, 10 were reciprocal translocations, eight had Robertsonian translocations, five had an inversion, and one had a ring chromosome. The likelihood of a chromosome abnormality was higher in men with a sperm density of <5 × 106/mL, an FSH ≥ 30.1 mLU/mL, an LH ≥ 8.9 mLU/mL, and a testosterone value ≤ 2.69 ng/mL [24].
In a review by Martin, the author noted that men with any type of infertility, whether low count, abnormal motility, or abnormal form, had an increased frequency of sperm chromosomal abnormalities, from 2 to 10 times higher than that of controls. She concluded that any abnormality of spermatogenesis confers an increased risk of aneuploid sperm. The highest risk was seen in macrocephalic, multinucleated, multiflagellate sperm, in which cases the risk of aneuploidy and polyploidy is 50–100% [25].
Sex Chromosome Abnormalities
(See also Chap. 10.)
Among men with infertility, the most frequent cytogenetic findings are 47,XXY and 47,XXY/46,XY. Men with this chromosome constitution commonly have the clinical features of Klinefelter syndrome, which include essentially normal appearance at birth but for a slightly small head; delayed puberty; higher incidence of gynecomastia than other males have; and small, firm testes with hyalinization of seminiferous tubules. Intelligence is usually normal, with performance IQ normal and verbal IQ below normal on average. Reading skills may be a problem [24]. These men have hypergonadotropic hypogonadism and azoospermia or very severe oligospermia. Although many of these men are diagnosed as boys, others are not diagnosed until such time as they are seeking the cause for their infertility. Given the incidence at newborn screening, it appears most males with 47,XXY or 47,XYY do not come to diagnosis. Studies of sperm chromosomes have shown that the frequency of aneuploidy for sex chromosomes varies from 1.5 to 7% in sperm from 47,XXY mosaics and from 2 to 45% in the sperm of non-mosaic 47,XXY men [25]. The majority of the babies born are chromosomally normal, but there is an increased risk for aneuploidy in the offspring of these men.
Men with 47,XYY or 47,XYY/46,XY karyotypes are usually fertile and typically have normal semen analyses [24]. They are slightly taller than their chromosomally normal brothers on average and on average have a normal IQ. About half have learning disabilities requiring special education. [24]. The incidence of men with 47,XYY is about the same as that of 47,XXY in the general population; each is present in about one in 1,000 newborns [24]. However, in infertility surveys—for example, the study by Gunduz et al., noted previously—the finding of 47,XXY is about nine times as frequent as that of 47,XYY [22]. Men with a 47,XYY karyotype are represented more frequently among infertile men (0.26%) than in newborn males (0.07%). Their semen analyses are usually normal, as previously noted, but in a minority of cases, they have severe abnormalities of spermatozoa number, motility, and/or morphology [26]. This was observed in a study of 47,XYY males by Martin, in which no sperm was disomic for sex chromosomes by chromosome analysis; 10,000 sperm studied by FISH showed an increase for XY disomy to 0.6%. Martin noted other labs had reported increased frequencies of sperm aneuploidy for sex chromosomes ranging from 0.3 to 15% [25].
Autosomal Abnormalities
The most common autosomal abnormalities seen in infertile men are Robertsonian translocations (see Chap. 9). In the aforementioned review by Van Assche, the incidence of infertile men with this finding was 0.7% [23]. This was 8.5 times the incidence in the newborn survey used for comparison. It appears that the increased frequency of the X-Y bivalent and the trivalents formed by the chromosomes involved in Robertsonian translocations is correlated with the extent of germ cell impairment [27] (see also Chaps. 2, 9, and 10). Martin noted in seven men with Robertsonian translocations that the actual frequency of unbalanced sperm by chromosome analysis is lower than theoretically expected, with 3–27% of sperm being unbalanced because of the translocation. FISH studies showed 7–40% of Robertsonian translocation heterozygotes were unbalanced, with a mean of 15% [25]. When Huang et al. looked at characteristics of embryo development in Robertsonian translocation carriers, they noted that by day 3, whether or not the embryos have a chromosome abnormality could not be determined based upon morphology, but by days 5–6, this was evident. On day 3 after fertilization, there were 161 high-grade embryos, including 59 normal/balanced embryos and 102 abnormal ones, whereas by days 5–6, the blastocyst percentage in the normal/balanced embryo group was significantly higher than that in the abnormal embryo group (44 vs. 20%, p = 0.0000) [28]. Huang also noted that several studies had shown a strong prevalence of alternate segregation in the gametes of Robertsonian carriers.
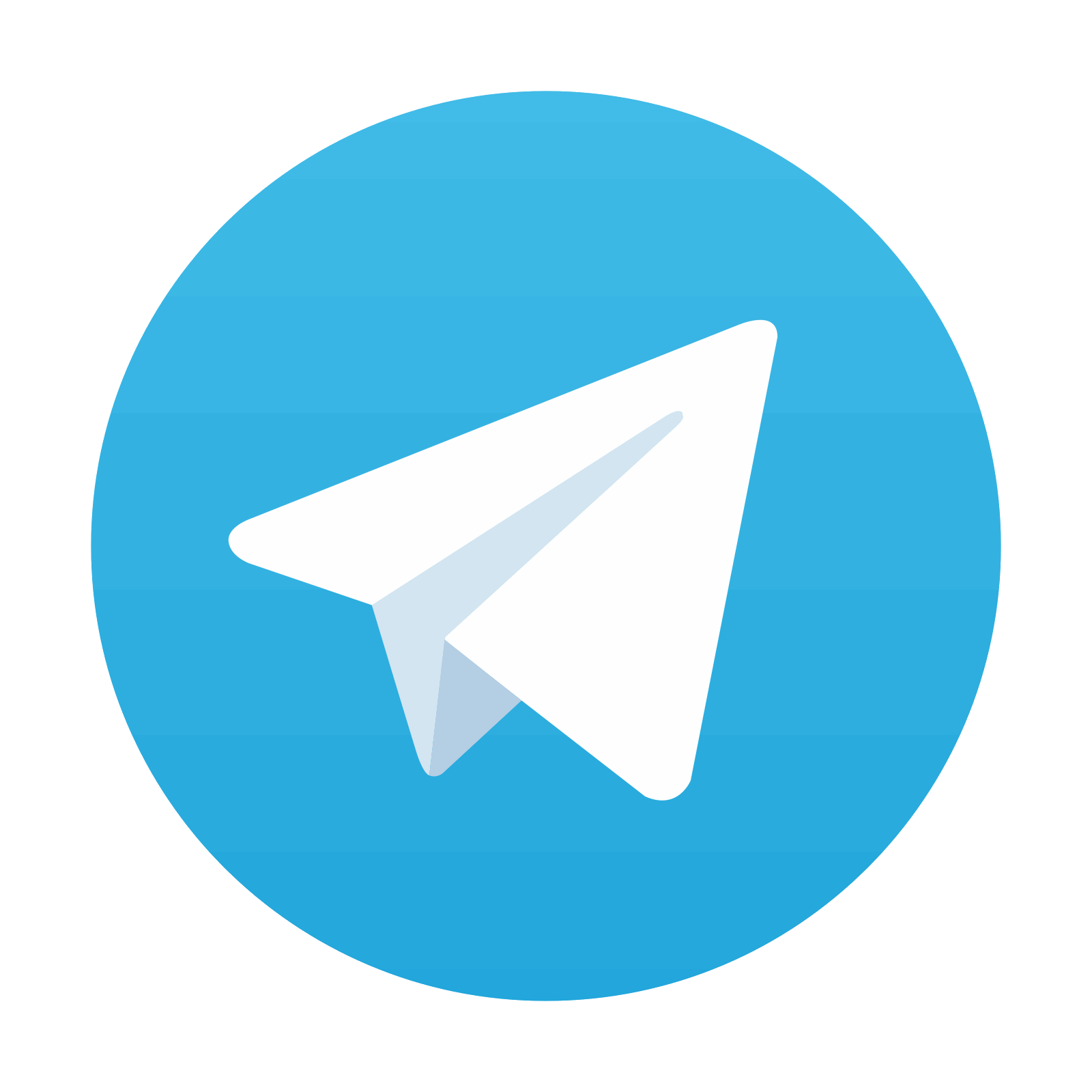
Stay updated, free articles. Join our Telegram channel

Full access? Get Clinical Tree
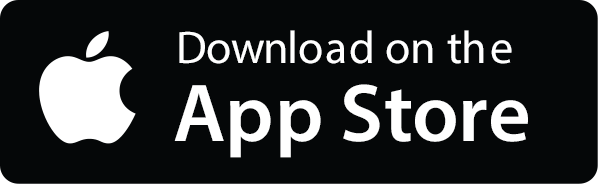
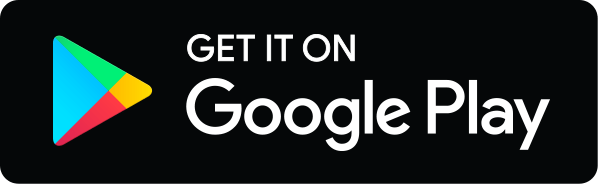