Fig. 10.1
Idiograms of the X and Y chromosomes showing the pseudoautosomal regions (PAR1 and PAR2), the locations of the X-inactive-specific transcript (XIST) gene, the critical region on Xq, the sex-determining region Y (SRY), the azoospermia factor region (AZF), the heterochromatic region of the Y, and the male-specific region located between PAR1 and PAR2
All genes within PAR1 escape X inactivation in women. One of the genes in this region, SHOX, requires two functional copies, without which there will be short stature with or without features of Leri-Weill dyschondrosteosis. If no functional copy of SHOX is present, a more severe skeletal dysplasia, Langer mesomelic dysplasia, results. Families segregating deletions or mutations of SHOX can have unusual inheritance patterns due to the very high recombination frequency in PAR1. Males may inherit the deleted or mutated gene from their mother but pass it to their sons due to recombination. This has important implications for genetic counseling [4].
X-Chromosome Inactivation
There are thousands of genes on the X chromosome but relatively few on the Y chromosome. The explanation for the fact that males survive quite nicely with only one X chromosome while females have two involves a concept called “dosage compensation,” and is termed the Lyon hypothesis after its proponent, Dr. Mary Lyon [5].
In somatic cells in females, only one X chromosome is active. X inactivation occurs early in embryonic life, beginning about 3 days after fertilization, and is completed by the end of the first week of development. The inactivation is random between the two X chromosomes. Either the maternal or paternal X can be inactivated, and after one X has become inactive, all the daughter cells from that original cell have the same inactive X. In female germ cells, the inactive X chromosome is reactivated as the cells enter meiosis, and in male germ cells, the single X chromosome becomes inactive.
The inactive X has properties characteristic of heterochromatin, with late DNA replication in the S phase of the cell cycle and remaining condensed during interphase. Histone proteins associated with the inactive X are underacetylated, and the cytosines in the CpG islands are methylated [6]. A gene that controls X inactivation is XIST (X-inactive-specific transcript) and is located at the X-inactivation center (XIC) at band Xq13 (Fig. 10.1). Only the inactive X expresses this gene. XIST codes for Xist RNA, which appears to coat or paint the inactive X chromosome and is responsible for inactivation [7]. About 15% of genes on the X-chromosome escape inactivation and remain active to some degree on both X chromosomes in females [8]. An additional 10% of genes show variable patterns of inactivation and are expressed to different extents from some inactive X chromosomes [9]. Many more genes on Xp escape inactivation as compared to Xq [6]. These genes are clustered and primarily map to the distal portion of the short arm [9]. In individuals with extra or missing X chromosomes, a single X remains active. However, in triploids, there are usually two active X chromosomes, suggesting a counting mechanism protecting a single X-chromosome inactivation for every two autosome sets [6]. Genes retaining Y homology tend to be expressed on the inactive X [9]. Although all genes in PAR1 escape X-chromosome inactivation (XCI), some in PAR2 do not escape XCI but achieve dosage compensation through inactivation on both the inactive X and the Y [10].
Early evidence for the existence of the inactive X was the observation of the Barr body, named for the Canadian cyto-logist Murray Barr [11]. This is a dark-staining chromatin body, present in one copy in normal females, which is the condensed, inactive X chromosome. Normal males have no Barr body. Initially, a buccal smear was obtained from patients to look for Barr bodies. Because of improved methods for looking at sex chromosomes and the inaccuracy of the buccal smear technique, it is now considered an obsolete test. The sex chromatin body in polymorphonuclear leukocytes takes the form of the “drumstick,” seen attached to the nucleus in approximately 2% of these cells in XX women but not in XY men [12].
Techniques for detecting the inactive X have been based on the fact that it is late replicating. The most commonly used cytogenetic method involves the use of bromodeoxyuridine (BrdU) [13]. Newer methods for detecting the inactive X involve molecular techniques often using differential methylation analysis [14–18].
Despite the fact that XIST was identified in 1990, there remains an incomplete understanding of the cis- and trans-acting elements that control X-chromosome inactivation and determine how some genes escape it as well as how XIST is only able to associate with the chromosome from which it is transcribed [19].
Skewing of X-chromosome inactivation (defined as greater than 80% of cells with one of the two chromosomes inactivated) may occur through XIST promoter mutations or mutation of other regulatory elements within the XIC, defined as primary skewing. Secondary skewing is post-X-chromosome inactivation selection due to slower proliferation of cells expressing a mutant allele. Evidence supporting this is a study by Amos-Landgraf in which 4.9% of cord blood samples but 14.2% of adult peripheral blood samples showed skewing of XCI [20].
The Y Chromosome
The Y chromosome is made up of several different regions. These include the pseudoautosomal regions at the distal short and long arm, PAR1 and PAR2, the heterochromatic region on the long arm, and the recently sequenced male-specific region of the Y (MSY) located between PAR1 and PAR2, with 78 protein-coding genes that encode 27 distinct proteins (Fig. 10.1). Twelve of the MSY genes are ubiquitously expressed in many organs throughout the body, while 11 MSY genes are expressed predominantly in the testes [21].
The testis-determining factor (TDF) that leads to differentiation of the indifferent gonads into testes is located on the short arm of the Y chromosome. TDF was mapped by molecular analysis of sex-reversed patients (chromosomally female but phenotypically male and vice versa), and the gene SRY (sex-determining region Y) was identified in 1990 [22]. It is located on the short arm of the Y at band p11.3 in the MSY region (Fig. 10.1). Deletions and mutations in this gene have been found in some 46,XY females (see Disorders of Gonadal (Testicular) Development).
The Y chromosome has a highly variable heterochromatic region on its long arm. The length of this region is usually constant from one generation to the next. A gene controlling spermatogenesis, termed the azoospermia factor (AZF), was first proposed by Tiepolo and Zuffardi in 1976 and mapped to the distal part of the euchromatic Yq11 region (Yq11.23) [23] (Fig. 10.1). In studies of men with azoospermia or severe oligospermia, deletions in different intervals of Yq11 have been found, and three nonoverlapping regions or azoospermia factors (AZFa, AZFb, and AZFc, from proximal to distal Yq) have been defined as spermatogenesis loci [24] (see also Chap. 11).
A locus for susceptibility to gonadoblastoma (GBY) has been proposed on the Y chromosome based on the high incidence of gonadoblastoma in females with 45,X/46,XY mosaicism or XY gonadal dysgenesis [25]. Deletion mapping has localized this putative gene to a region near the centromere but has raised the possibility of multiple GBY loci dispersed on the Y chromosome [26, 27]. One of the most likely candidate genes in this region is the testis-specific protein Y-encoded (TSPY) gene. This gene functions normally in male germ cell proliferation and differentiation but is ectopically expressed in early and late stages of gonadoblastoma [28, 29].
Numerical Abnormalities of the Sex Chromosomes
Introduction
Numerical abnormalities of the sex chromosomes are one of the most common types of chromosomal aneuploidy, with a frequency of 1 in 500 live births. This might be the result of the fact that abnormalities of sex chromosomes have less severe clinical abnormalities and are more compatible with life as compared to autosomal disorders. Reasons for this include inactivation of all additional X chromosomes and the small number of genes on the Y chromosome.
Sex chromosome disorders are more commonly diagnosed prenatally than autosomal aneuploidies, and genetic counseling for these conditions is often more complex and challenging than that for an autosomal abnormality. In the past, many individuals with sex chromosome disorders would have gone through life undetected, as they do not have physical or developmental problems that would have warranted a chromosome study [30]. Women undergoing amniocentesis and chorionic villus sampling (see Chap. 12) should be informed about the possibility of detecting a sex chromosome disorder, and when a fetal sex chromosome abnormality is detected prenatally, information should be provided to the patient by a clinical geneticist or pediatric endocrinologist when possible [30]. Cytogenetics labs reporting results to physicians and genetic counselors discussing results with patients should provide up-to-date and accurate information about these conditions. It is important for couples faced with having a child with a sex chromosome disorder to see the potential problems for developmental delay in the context of any chromosomally normal child having a risk of developmental delay (by definition, a 5% chance) [30].
There appears to have been a trend toward a higher rate of pregnancy continuation in more recent years [31]. This might be at least in part from results of long-term studies of individuals with sex chromosome disorders revealing a better prognosis than previously reported. [32]. Average percentages of pregnancy terminations for sex chromosomal aneuploidies reported range from 10 to 88% depending on the population, type of aneuploidy, maternal age, presence of fetal abnormalities on ultrasound, and the medical professional providing information [33–39].
Turner Syndrome
45,X (and its variants) occurs in approximately 1 in 2,500 live-born females but is one of the most common chromosome abnormalities in spontaneous abortions and is estimated to occur in 1–2% of all conceptuses. The syndrome was first described in 1938, and a report that it is caused by a single X chromosome appeared in 1959 [40, 41]. The older medical literature sometimes referred to the Turner syndrome karyotype as 45,XO. This terminology is incorrect and should not be used; there is no O chromosome.
Ninety-nine percent of 45,X conceptuses result in spontaneous loss, usually by 28 weeks (Fig. 10.2). These fetuses usually have ultrasound abnormalities including a cystic hygroma and hydrops. Although 45,X is quite lethal in the fetus, those that survive to term have relatively minor problems. The reasons for this are not known, although it has been speculated that all conceptions that survive have some degree of undetected mosaicism for a normal cell line [42]. Prenatally diagnosed cases with mosaicism for a 45,X cell line and a cell line with a second structurally normal sex chromosome usually result in the birth of a child with a normal phenotype [43, 44].


Fig. 10.2
A 45,X fetus with large cystic hygroma and hydrops
Origin of the X Chromosome in Turner Syndrome
In approximately 75% of patients with 45,X, the X chromosome is maternal in origin [37, 45, 46]. There is no parental age effect [46]. Although phenotypic differences have not been found between Turner patients with a maternal or paternal X chromosome, there may be some cognitive differences particularly in memory function [47]. This has been theorized to be on the basis of an imprinted X-linked locus; however, no human imprinted X-linked genes have yet been identified [19, 48].
Phenotype
Clinical features of Turner syndrome in newborns may include decreased mean birth weight (average weight 2,800 g), posteriorly rotated ears, neck webbing (Fig. 10.3a), and edema of hands and feet (Fig. 10.3c, d), although more than half are phenotypically normal [49]. Congenital heart defects, especially coarctation of the aorta, and structural renal anomalies are common and should be checked for. Most older children and adults with Turner syndrome have short stature and ovarian failure, and variable dysmorphic features including down-slanting eyes, posteriorly rotated ears, low posterior hairline, webbed neck (Fig. 10.3a), a broad chest, short fourth metacarpals (Fig. 10.3b), and cubitus valgus [49]. Adults with Turner syndrome have a four- to fivefold increased rate of premature mortality, mainly due to complications of congenital heart disease [50]. There is a risk of aortic dilatation leading to dissection. Regular follow-up throughout life with a cardiologist and cardiac magnetic resonance imaging is recommended [49]. Without hormonal supplementation, there is usually lack of secondary sex characteristics. The gonads are generally streaks of fibrous tissue. Although germ cells normally form in the 45,X embryo, there is accelerated oöcyte loss by 15 weeks of gestation [51]. Standard treatment includes use of growth hormone and estrogen. It is recommended that these patients be followed by endocrinologists familiar with Turner syndrome. There are several published guidelines for health supervision for children and adults with Turner syndrome [49, 52–55].


Fig. 10.3
Child with Turner syndrome and low posterior hairline and webbed neck (a) and short fourth metacarpals (b). Infant with Turner syndrome and lymphedema of the hand (c) and feet (d)
The SHOX gene, located in the distal part of the pseudoautosomal region on Xp, escapes X inactivation. Haploinsufficiency for SHOX causes short stature and Turner skeletal features [56–58]. A gene determining lymphedema has been proposed at Xp11.4 [59].
Some degree of spontaneous puberty occurs in 10–30% of girls with Turner syndrome, but fewer reach complete puberty with menarche, and most require estrogen treatment beginning at age 12 for normal secondary sex characteristic development, and throughout adulthood to prevent osteoporosis [49]. Spontaneous pregnancy may occur in 2–5% [49, 54].
Development
Intelligence in individuals with Turner syndrome is average to above average, although there is an increased risk of a nonverbal learning disorder and behavioral problems. Infants can have feeding problems and developmental delay. Problems with visual-spatial skills, working memory, executive functions, and social skills can occur. Verbal IQ scores are typically higher than performance IQ [55]. With appropriate therapeutic and educational intervention, women with Turner syndrome can do well academically and socially.
Turner Syndrome Variants
Approximately half of all individuals with Turner syndrome have a 45,X karyotype. The remainder exhibit mosaicism and/or structural abnormalities of the X chromosome. In a study of cytogenetic and cryptic mosaicism in 211 patients with Turner syndrome, Jacobs et al. reported pure 45,X in 46%, 47% had a structurally abnormal sex chromosome (41% with an abnormal X and 6% with an abnormal Y), and 7% had a 46,XX or 47,XXX cell line [60]. Two patients were found to have cryptic X mosaicism, and none had cryptic Y mosaicism.
Mosaicism
Mosaicism for 45,X and another cell line is found in 15–20% of patients with Turner syndrome with lymphocyte chromosome analysis. A 46,XX cell line may modify the phenotypic features of the syndrome. As mentioned earlier, in order to explain why 99% of 45,X conceptions terminate in miscarriage, it has been proposed that most surviving 45,X fetuses have some degree of mosaicism. In a study of both lymphocytes and fibroblast cultures in 87 patients with Turner syndrome, mosaicism was found in 66.7% [61]. In a patient with several typical features of Turner syndrome but normal lymphocyte chromosome analysis, analysis of another tissue such as skin for fibroblast culture or buccal cell analysis using an X-chromosome probe and fluorescence in situ hybridization (FISH; see also Chap. 17) should be considered [61, 62].
In prenatal diagnosis, when multiple cells from a single culture are identified with a 45,X karyotype, a moderate work-up is warranted with examination of an additional 20 cells from cultures other than the one with the initial finding or 12 colonies from coverslips other than the one with the abnormality [62, 63]. Markers identified prenatally should have their origin determined using X and Y centromere FISH. When a small ring or marker chromosome is determined to be derived from the X chromosome, FISH with a probe for XIST should be performed. Lack of the XIST locus in a ring X chromosome may be associated with a more severe phenotype that includes developmental disability (see section “Ring X”).
Mosaicism with a Y Chromosome
Patients with 45,X/46,XY mosaicism may have external genitalia ranging from normal male to ambiguous to female with features of Turner syndrome. The Y chromosome is often structurally abnormal. One study of 92 prenatally diagnosed cases found that 95% had normal male genitalia. Abnormal genitalia included hypospadias, micropenis, and abnormal scrotum. In those fetuses for which pathologic studies were possible, 27% had abnormal gonadal histology, classified as dysgenetic gonads. The percentage of mosaicism found in amniotic fluid samples was a poor predictor of the phenotype [44]. Another study of 42 cases of 45,X/46,XY mosaicism diagnosed prenatally found phenotypically normal male offspring in 90%, with 10% having questionably abnormal phenotypes, including three cases with mixed gonadal dysgenesis [64]. In another study, three of 27 patients with mosaic 45,X/46,XY diagnosed postnatally had mixed gonadal dysgenesis (a streak gonad on one side and testis on the other) and normal plasma testosterone levels. The streak gonads were removed in these patients but the testes were not, and all three had normal puberty. Mild intellectual disability (mental retardation) and autism were seen in four and two patients, respectively, in this series, although there might have been biased ascertainment [65]. Abnormal gonadal development including gonadal dysgenesis, infertility, low testosterone level, and azoospermia can occur in patients with 45,X/46,XY and an apparently normal external male phenotype [65]. Fertility cannot be evaluated until puberty, but infertility is common.
Using fluorescence in situ hybridization (FISH) analysis, Robinson et al. looked at the structure of the Y chromosomes present in 14 cases of Turner syndrome with at least one cell line with an abnormal Y chromosome [66]. Ten patients had a pseudodicentric Yp chromosome, two had an isodicentric Yq, one a pseudodicentric Yq, and one had a derivative Y chromosome (see Chap. 9). Results suggested that the majority of Turner syndrome patients with structurally abnormal Y chromosomes contain two copies of most of the euchromatic Y material (see the section “Structural Abnormalities if the Y Chromosome,” later). In a study of 211 patients with Turner syndrome, Jacobs et al. found a clinically significant structural abnormality of the Y chromosome in 13 patients (6%) [60]. One hundred cells were examined from each patient. No patients were found to have 45,X/46,XY mosaicism with a structurally normal Y.
The frequency of occult Y mosaicism in Turner syndrome has varied widely depending on the study and type of analysis used. One study using polymerase chain reaction (PCR) and Southern blot analysis found that 40% of 45,X patients had SRY sequences [67]. Most patient samples produced only a faint signal, indicating a low percentage of cells with Y-chromosome material (or contamination). A more recent study using PCR and Southern blot analysis found evidence of Y mosaicism in only 3.4% of patients with Turner syndrome who were cytogenetically nonmosaic [68]. In a population study in Denmark of 114 females with phenotypic Turner syndrome, 12.2% of patients had Y chromosome material by PCR analysis. Fifty percent of these patients did not have evidence of Y detected by karyotype analysis [69]. Nishi et al. studied 122 patients with Turner syndrome and compared results of nested PCR in these patients with those of 100 women with no known chromosome abnormality [70]. First-round PCR identified Y sequences in four patients (3%); all were also found to have a marker chromosome with cytogenetic analysis. Fourteen percent of DNA samples from 100 “normal” women showed positive amplification after nested PCR. The authors hypothesized contamination with PCR amplification products. The possibility of microchimer-ism in these women secondary to having had a male conception or a male twin has also been raised [71]. Jacobs et al. found no occult mosaicism for Y in 178 patients with Turner syndrome using Y-specific PCR primers [60]. Thirteen patients had Y-chromosome material detected by routine cytogenetic analysis of 100 cells. Only two of the thirteen patients had fewer than 10% of cells with a Y chromosome: one had 7% and one had 8%. It is likely that these would have been detected with standard analysis of 30 cells [60].
These studies suggest that the presence of cryptic mosaicism involving the Y chromosome is rare and might involve less than 1% of patients. Nested PCR appears to overestimate the frequency of Y sequences in patients with Turner syndrome and should be avoided to prevent false-positive results, which lead to unnecessary surgical treatment in these patients [70]. At this time, PCR analysis for Y sequences in patients with Turner syndrome does not appear to be warranted unless a marker chromosome is found. In such instances, identification of the marker with FISH or molecular analysis is critical due to the risk of gonadoblastoma. Most marker Y chromosomes in patients with Turner syndrome should be detected with standard G-band analysis of 30 cells. The use of FISH analysis to look for Y-chromosome mosaicism in 45,X patients has been recommended [52, 62]. If a 30-cell analysis reveals an apparently nonmosaic 45,X karyotype in patients without virilizing features, FISH analysis using X and Y probes should be performed to identify low-level sex chromosome mosaicism to prevent a potential life-threatening cancer [62, 72]. Identification of mosaicism for a marker, ring, or isochromosome derived from the X chromosome eliminates the need to pursue additional investigation for Y-chromosome material. All ring and marker chromosomes should be further analyzed with FISH to determine their origin.
If a patient with Turner syndrome has evidence of virilization with clitoromegaly or other masculinizing features, mosaicism for a Y-containing cell line is likely. FISH with X and Y centromere probes should be performed on a minimum of 200 cells to detect low-level mosaicism. If lymphocyte analysis is normal, study of a second tissue should be considered [62].
The risk of gonadoblastoma is increased in phenotypic females with 45,X/46,XY mosaicism [73]. Some but not all patients with Turner syndrome and a Y cell line will have masculinization. In one study, gonadoblastoma was found in one of ten patients with Y-chromosome material who had ovariectomy performed [69]. This patient had a 45,X/46,XY karyotype. None of the patients with only PCR-detected Y-chromosome material developed gonadoblastoma. In another study, 10 of 171 patients with Turner syndrome without clitoromegaly or other evidence of masculinization had Y-chromosome mosaicism with conventional cytogenetic analysis of at least 50 metaphase cells. Four additional patients were identified using PCR for SRY and the DYZ3 region, two of whom subsequently had marker chromosomes identified cytogenetically. Of the 12 who underwent gonadectomy, gonadoblastoma was identified in 4 (33.3%), with the youngest patient aged 2.8 years. The percentage of Y-bearing cells in the blood or gonadal tissue did not correlate with gonadoblastoma incidence. Combining their data with previous studies, there was a 26.9% risk of gonadoblastoma in patients with 45,X and Y-chromosome material. In those with gonadoblastoma, the risk of malignancy was 14% [72]. As the risk of malignant degeneration of gonadoblastoma cannot be predicted, surgical removal of gonadal tissue is generally indicated for patients with Turner syndrome and Y-chromosome material. The appropriate timing for this surgery should be discussed with a pediatric endocrinologist. For those patients with a male phenotype and external testes, the risk of neoplasm is not as high, but frequent physical and ultrasound examinations are recommended [74].
Isochromosome X
An isochromosome X [i(X)(q10)] (see Fig. 10.4), consisting of two copies of the long arm (missing all or most of the short arm), is seen in 18% of patients, either as a single cell line or, more commonly, in mosaicism with a 45,X cell line. Although most i(Xq) chromosomes look monocentric, molecular evidence suggests that many are actually dicentric and result from breakage within the proximal short arm of a single X chromosome with subsequent reunion of the sister chromatids [60, 75]. The i(Xq)s are equally likely to be maternal or paternal [60]. The error occurs during male or female gametogenesis. When the error occurs postzygotically, it is likely to be at the first postzygotic division because 45,X/46,X,i(X)(q10)/46,XX mosaics are extremely rare [60].


Fig. 10.4
Normal X chromosome and isochromosome Xq: 46,X,i(X)(q10) in a patient with Turner syndrome. Brackets indicate region of duplication on Xq
These patients are phenotypically indistinguishable from those with a pure 45,X karyotype although there have been reports of an increased risk of autoimmune problems in patients with an isochromosome X [76]. In a study of 145 women with Turner syndrome, 83% with an i(Xq) had positive thyroid autoantibodies compared with 33% of women with other karyotypes. The women with an i(Xq) chromosome were also more likely to become hypothyroid (37.5%) and require thyroxine compared to the 45,X women (14%) [77].
Ring X
A subset of patients with Turner syndrome, estimated at approximately 15%, have one normal X chromosome and a ring X chromosome, most often as a mosaic cell line [60]. In a study of patients with a 45,X/46,X,r(X) karyotype in whom the parental origin of the ring could be determined, the ring was derived from the opposite parent from the normal X. In one patient, there was uniparental disomy (UPD) for the X chromosome (see Chap. 20). In two-thirds of patients, the normal X was maternal in origin, and in one-third of patients, it was paternal, as it is in 45,X patients [78]. The size of the ring varies from minute to nearly the size of a normal X. An important first step in characterizing a ring is to perform FISH (see Chap. 17) with a painting probe for the X to confirm that it is derived from the X and not Y [79] (see the section “Mosaicism with a Y Chromosome”).
In general, ring X patients lack many of the classic somatic features of Turner syndrome [80]. Those with ring X often do not have lymphedema and, therefore, are less often ascertained at birth compared to 45,X individuals [81]. Although some have typical features of Turner syndrome, others have a severe phenotype with developmental disability, facial dysmorphism, and congenital malformations. Some patients with a ring X and severe phenotype have features similar to those of patients with Kabuki syndrome, a multiple malformation syndrome due to mutations in the MLL2 gene on chromosome 12 [80–83]. El Abd et al. reported a characteristic behavioral profile in five patients with a small active ring X that included autistic-like features, obsessive compulsive behavior, and social and communication problems [84].
Studies have suggested that the more severely affected patients have smaller rings that are deleted for XIST. It has been hypothesized that the lack of XIST causes the ring to fail to inactivate, thus causing functional disomy for genes present on the ring, resulting in phenotypic abnormalities [85–87]. Larger rings have XIST present and are preferentially inactivated. However, Turner et al. reported that in seven females with 45,X/46,r(X) and a XIST-negative ring, only one had a severe phenotype and this was explained by the absence of XIST expression, a large amount of Xp material in the ring, and, possibly, the concomitant maternal uniparental isodisomy [88] (see Chap. 20). The remaining six patients had physical phenotypes consistent with Turner syndrome. The size of the ring X chromosome lacking XIST correlates with the degree of clinical severity [81, 87–89]. Those with extremely small rings have been found to have cognitive functioning similar to those with 45,X. There could be particular gene sequences that, when functionally disomic, result in the severe physical phenotype.
Other factors that could contribute to the phenotype in patients with small ring X chromosomes are the tissue-limited distribution of the ring X cell line or ring formation from an inactive X after the establishment of X inactivation. In patients with an inactivated ring X chromosome, having a larger proportion of cells with the ring was associated with lower verbal and nonverbal IQ scores [88, 89]. Migeon et al. reported two patients with inactive ring X chromosomes, mental retardation, and a severe phenotype [90]. Cultured fibroblasts from these patients showed a second ring in a small percentage of cells. The authors hypothesized that the severe phenotype with an inactive X chromosome is the result of the presence of a second ring X that was active in some tissues during embryogenesis.
The prognosis for patients with small ring X chromosomes might be better than previously proposed [87]. However, a ring X chromosome appears to be associated with a substantially increased risk of significant learning difficulties, requiring special educational provision, compared to 45,X [89]. It might not be possible to accurately predict, prenatally, the phenotype that will be associated with the ring X chromosome after birth. Although a relatively large, active ring X (XIST not expressed) is more likely to be associated with severe phenotypic abnormalities, demonstration of an inactive ring X is not necessarily reassuring [90]. The etiology of the abnormal phenotype in ring X is complex and cannot be based solely on the inactivation status of the ring. Size and gene content, extent of X inactivation, parental origin, and timing of ring formation and of cell selection likely play a role in the broad phenotypic variability [79].
45,X/47,XXX Mosaicism
Approximately 2% of patients with Turner syndrome have a 45,X/47,XXX mosaic karyotype [60, 91]. A study of seven girls with this type of mosaicism aged 6.1–20.4 years found that three of seven did not require growth hormone, five of six girls older than 10 years had spontaneous puberty, and four of five girls older than 12 years had spontaneous menarche with regular menstrual cycles without medication. No renal or cardiac anomalies and no cognitive or behavioral problems were found in this small group of patients. In general, patients with Turner syndrome caused by 45,X/47,XXX mosaicism are more mildly affected clinically with regard to phenotype and ovarian function [91].
Marker Chromosomes in Patients with Turner Syndrome
It is important to identify the origin of a marker chromosome in a patient with Turner syndrome, because of the risk of gonadoblastoma if it is made up of Y material (see the section “Mosaicism with a Y chromosome” earlier) or the increased risk of phenotypic and developmental abnormalities if the marker is of autosomal origin. This can be done either with FISH or molecular techniques.
47,XXX
Trisomy X or triple X is the most frequent sex chromosome disorder present at birth in females, occurring in 1 in approximately 1,000 live female births [92]. It was first described in 1959 by Jacobs et al. [93]. Unfortunately, the term originally used for this cytogenetic abnormality was “superfemale,” which gives a misconception of the syndrome and is no long-er in use. Due to variation in phenotype, with some individuals very mildly affected, only approximately 10% of cases are ascertained clinically [94].
Origin
Most 47,XXX conceptions result from maternal nondisjunction at meiosis I, and so there is an association with increased maternal age. Two of the X chromosomes are inactivated, and abnormalities could result from three active X chromosomes early in embryonic development, prior to X inactivation and/or from genes on the X chromosome that escape inactivation.
Phenotype
In contrast to the result of a 45,X karyotype, there is not a recognizable syndrome in females with an additional X chromosome. The majority are physically normal, although there is a slight increase in the frequency of minor anomalies. The mean birth weight is at the 40th percentile, the mean birth length is at the 70th percentile, and the mean birth head circumference is at the 30th percentile [95]. In general, as adults, these women have moderately tall stature, with an average height of 172 cm (5 ft and 7.7 in.). Pubertal development is normal, and most have normal fertility, although a small number have ovarian dysfunction and premature ovarian insufficiency sometimes associated with autoimmune thyroid disease [96, 101, 97]. Renal and ovarian abnormalities and congenital heart defects have been reported in small numbers of patients. There appears to be an increased risk of seizures [94]. Most have good health, although one study found that 25% had recurrent nonorganic abdominal pain as teenagers [98].
Development
Usually, 47,XXX females have normal intelligence, but most have lower IQs than their siblings. There is a great deal of variability and many women have normal intelligence and are well adjusted. Precise predictions regarding an individual child’s prognosis are not possible, but there does appear to be a risk for mild to moderate developmental problems in the areas of motor, speech and language, and learning [95, 101 102]. Verbal IQ tends to be lower than performance IQ, with many having problems with expressive language.
Many studies of 47,XXX females have ascertainment bias; however, in a group of 11 females with 47,XXX ascertained at birth by unbiased screening of all newborns who were then followed into adulthood, most had serious patterns of dysfunction [102]. Most showed early delays in motor, language, and cognitive development and were described as shy, withdrawn, and immature, with poor coordination [95]. The full-scale IQ was 26 points lower than in normal sibling controls; average IQ was in the 85–90 range, and subjects were at the 24th percentile in academic achievement scores, but mental retardation was rare. Nine of the eleven needed special education in high school either full time or part time, and less than half completed high school, but two achieved “A”s and “B”s, and one excelled in math. Most did not participate in extracurricular activities. They were described as socially immature. All had heterosexual orientation. Compared to individuals with other types of sex chromosome disorders, 47,XXX females seemed to have the most psychological problems, including depression and, occasionally, psychoses. However, one woman attended college, and many were able to overcome psychological problems and become independent, hold jobs, and marry. Stability of the home environment was somewhat related to outcome but not to such an extent as is seen in other sex chromosome disorders [102]. In adulthood most of these women were employed full time (unskilled or semiskilled jobs), had married, and had children [103]. In another long-term study, 47,XXX women were found to have more work, leisure, and relationship problems as compared to a control group [104]. They were found to have poorer psychosocial adaptation and more psychiatric impairment as compared to their female siblings. However, most were self-sufficient and functioned reasonably well as adults. Severe psychopathology and antisocial behavior were rare, and there was a larger variability in behavioral phenotype than originally appreciated [104]. In another longitudinal study of 16 girls with 47,XXX ascertained at birth through a cytogenetic survey of consecutive newborns, 50% had speech delay and IQ scores averaged 85.3 verbal (range 67–109) and 88.3 performance (range 67–110). Higher IQ scores were positively correlated with the level of parental education. All attended regular schools, but most required extra help in math and reading. Behavior problems required psychiatric referral in 25% of the girls [105].
In a study of five girls ranging in age from 7 to 14 years with 47,XXX diagnosed prenatally, only one had motor and language delays and learning problems; the others had normal IQs (range 90–128) and were doing well in school [106]. Another longitudinal study by Linden and Bender of 17 47,XXX females, ages 7–18, initially diagnosed prenatally, found that eleven needed academic assistance and seven required speech therapy. Many were shy in younger years but became more outgoing as teenagers, and three had no developmental problems. Their IQs ranged from 73 to 128, with a mean of 103. The girl with a 73 IQ was from a family with a history of learning problems [107]. Reasons for the difference between the two groups could be the higher socioeconomic status and greater stability of the prenatally diagnosed group.
Experts in this field advocate for anticipatory guidance for these patients, emphasizing the child’s normal development but remaining prepared to identify and provide early intervention when developmental problems occur. Appropriate speech, occupational and physical therapy, educational assistance, and psychiatric treatment should be instituted as soon as a need is identified [104]. There have been several recent reviews of 47,XXX [94, 105, 106].
Variants with Additional X Chromosomes
48,XXXX
As compared to 47,XXX, there is almost always mild to moderate intellectual disability (mental retardation) in individuals with 48,XXXX or tetrasomy X syndrome, with an average IQ of 60, ranging from less than 30 to 75 [110]. One individual was reported to have a normal IQ [111]. Phenotypic features include mild hypertelorism, epicanthal folds, micrognathia, and midface hypoplasia [112]. Tall stature is common, with an average height of 169 cm (approx. 5 ft and 6 in.) [110]. Skeletal anomalies include radioulnar synostosis and fifth finger clinodactyly. Incomplete development of secondary sex characteristics could occur with scant axillary and pubic hair and small breasts, and some patients have gonadal dysgenesis [113]. Speech and behavioral problems are common. Fertility is reduced, and primary ovarian failure has been reported, although some have had chromosomally normal offspring [114].
49,XXXXX
Nondisjunction in successive meiotic divisions is the probable mechanism for pentasomy X syndrome, and molecular studies have shown that, at least in some cases, the extra X chromosomes are all maternally derived [115, 116].
Phenotypic features seen in penta-X females include intrauterine growth restriction, short stature, microcephaly, up-slanting palpebral fissures, low hairline, and coarse, Down syndrome-like facial features. Congenital heart defects, renal anomalies, hip dysplasia, and joint subluxation have been reported [117–120]. One individual with penta-X and hyper-IgE syndrome has been reported [121]. An infant with laryngomalacia and extreme neonatal hypotonia has been reported with mosaicism for tetrasomy X and pentasomy X [122]. Most have moderate developmental disability (IQ range 20–75, average IQ 50) and are described as shy and cooperative [110, 120]. There have been no reports of pregnancy in women with this chromosomal aneuploidy [110].
47,XXY (Klinefelter Syndrome)
Klinefelter syndrome (KS) was the first sex chromosome disorder to be described and its cytogenetic cause identified, and is the most common cause of hypogonadism and infertility in males [120, 123–125]. It is found in approximately 1 in 575–1,000 newborn males, although only 25% are ever diagnosed [30, 92, 126].
Origin
In one study, the extra chromosome arose at paternal meiosis I in 53% of patients, 34% at maternal meiosis I, 9% at maternal meiosis II, and 3% from postzygotic errors. There is an association with increased maternal age in those with maternal meiosis I errors [127]. A recent study found the additional X chromosome to be paternal in approximately 25% of cases, and these were associated with increased paternal age, although other studies have not supported this finding [128, 129].
Maternal nondisjunction during meiosis I leads to uniparental heterodisomy for the X chromosome, while an error during meiosis II results in uniparental isodisomy (see Chap. 20). If the father contributes the X, there are two different X chromosomes with different parental origins. So far, studies have found contradicting results regarding differences in phenotype and development between KS males with a paternal versus a maternal additional X [130]. No phenotypic differences between heterodisomy and isodisomy for the X in KS have yet been found. The repeat length and inactivation status of the androgen receptor at Xq11.2-q12 has also been studied as to its correlation with the KS phenotype, but so far there have been no definitive findings [130].
Phenotype
47,XXY males have taller than average stature, with mean height at the 75th percentile, and might have a eunuchoid build with long limbs and pear-shaped hips, although there is a great deal of phenotypic variability [98]. Head circumference during infancy is usually average but, beginning at age 4 years, tends to be below the mean for age, although generally within normal limits [131]. Testicular and penile size is usually small during childhood, although prepubertal phenotype is often unremarkable. Gynecomastia occurs in up to 50% of 47,XXY males during adolescence. Most enter puberty normally, although there is usually inadequate testosterone production and most require testosterone supplementation. Measurement of serum testosterone level in male infants with Klinefelter syndrome at 6 weeks of age can help predict the amount of natural testosterone production these patients will have.
Testes are small in adulthood, and there is hypergonadotropic hypogonadism. Almost all have infertility with absent spermatogenesis, tubular hyalinization, and Leydig cell hyperplasia. Most are born with spermatogonia, but during early puberty, the spermatogonia are thought to undergo massive apoptosis along with damage to the germinal epithelium [132]. Many men with KS are diagnosed in adulthood, with a chief complaint of infertility, but based on a population study, as many as 74% might never be diagnosed [30]. There have been more than 200 reports of pregnancies resulting from intracytoplasmic sperm injection (ICSI) from nonmosaic 47,XXY patients [130]. In one study of 38 pregnancies, there were 34 karyotypically normal neonates, two karyotypically normal pregnancy losses, one healthy unkaryotyped neonate, and one 47,XXY prenatally diagnosed fetus [133] (see also Chap. 12).
Development
Boys with Klinefelter syndrome have been reported to have decreased muscle tone during infancy, delayed speech and language skills, and an increased incidence of reading disability and dyslexia [134]. IQs are lower than in controls and compared to siblings, with the average between 85 and 90, although there is a wide range [98]. Verbal IQ is usually lower than performance IQ. The majority require special help in school, especially in the areas of reading and spelling. They are often described as awkward with mild neuromotor deficits, shy, immature, restrained, reserved, and lacking confidence [98]. A group of 13 males with Klinefelter syndrome, diagnosed as newborns and followed into adulthood, were said to have struggled through adolescence with limited academic success but were able to function independently in adulthood [102]. Most needed at least some special education help in school; nine completed high school, and four went to college. All were heterosexual. Some had psychological problems, including conduct disorder and depression, and difficulties with psychosocial adjustment. A stable and supportive family environment was found to correlate with better outcome [102]. Another long-term study of this group found that as adults, ten of eleven were employed full time and eight had married [103].
In a group of five boys ranging in age from 7 to14 years who had been prenatally diagnosed with 47,XXY karyotypes, there were fewer language and motor deficits in childhood as compared to the postnatally diagnosed group, and all were doing well in school. IQs ranged from 90 to 131. The reason for the better outcome might be the result of environmental and other genetic factors [108]. In a long-term study of 14 prenatally diagnosed boys with Klinefelter syndrome followed to 7–18 years, ten had average school performance, eight required educational assistance, three had attention deficit disorder, and four had speech problems. In general, they were healthy and had pleasant personalities [109]. A study of 50 boys with KS diagnosed prenatally and through clinical ascertainment found problems with complex language processing, impaired attention, and motor function [134]. An excellent review of the developmental phenotype in KS is by Boada et al. [135].
In summary, individuals with Klinefelter syndrome can have productive and fulfilling lives but often require special assistance in school and could have social and behavioral problems. Early evaluation and intervention are strongly recommended because the prognosis can be improved significantly with appropriate therapeutic intervention [136].
Variants with Additional X or Y Chromosomes
48,XXYY
This is the most common variant of Klinefelter syndrome [110]. There is overlap between this condition and both Klinefelter and XYY syndrome (see the section 47,XYY). The incidence is estimated at 1 in 50,000 male births [137]. Men are tall statured, with adult height above 6 ft, a eunuchoid body habitus, and long thin legs. There is hypergonadotropic hypogonadism, small testes, and sparse body hair. Gynecomastia occurs frequently [110].
Most 48,XXYY patients have mild intellectual disability, although IQs ranging from 60 to 111 have been reported. Speech and motor delays and hypotonia are seen in 75%, with average age at ambulation of 18 months [138]. Developmental outcomes described range from milder language-based learning disability (reading disability, dyslexia) to mild mental retardation in 30% of patients. Overall adaptive functioning is often more impaired than would be expected based on IQ scores [139]. Psychosocial and behavior problems are generally more severe than in 47,XXY individuals, although patients without significant behavior problems have been reported [110, 140]. Four patients with 48,XXYY observed over a 10-year period had psychiatric disorders, including aggressiveness, self-injury, and mental retardation [141].
48,XXYY is not associated with advanced parental age. Nondisjunction in both the first and second male meiotic divisions leading to an XYY sperm has been hypothesized [142].
48,XXXY
This is a rare condition, with more abnormal features and developmental disability as compared to 47,XXY. It was first described by Barr in 1959 [143]. Stature is normal to tall, and dysmorphic features include epicanthal folds, hypertelorism, protruding lips, prominent mandible, radioulnar synostosis, and fifth finger clinodactyly. There is hypergonadotropic hypogonadism and hypoplastic penis in 25% of patients, and gynecomastia is common. Testosterone therapy has been shown to be beneficial. Affected individuals are infertile.
Males with this condition have mild to moderate intellectual disability, with most in the 40–60 IQ range, although an individual with an IQ of 79 has been reported [110]. Most have speech delay, slow motor development, and poor coordination. Behavior is immature, with personality traits described as passive, pleasant, placid, and cooperative [110]. A 10–15 point decrease in IQ for each additional X chromosome has been reported, although there is still wide variability in outcomes [144].
49,XXXXY
49,XXXXY has an approximate incidence of 1 in 85,000 male births, and more than 100 cases have been described in the literature since this karyotype was first reported by Fraccaro et al. in 1960 [145]. It results from nondisjunction of the X in maternal meiosis I and II [146].
Common features include low birth weight, short stature in some patients, craniofacial features consisting of round face in infancy, and coarsening of features in older age, with hypertelorism, epicanthal folds and prognathism, and a short, broad neck [110, 142]. Congenital heart defects are found in 15–20%, with patent ductus arteriosus the most common defect described, but atrial ventricular septal defects and tetralogy of Fallot are also reported [110, 147, 148]. Skeletal anomalies include radioulnar synostosis, genu valgus, pes cavus, and fifth finger clinodactyly. Muscular hypotonia and hyperextensible joints are often present. There is hypergonadotropic hypogonadism [110]. Genitalia are hypoplastic with short penile length, small testicular volume, and cryptorchidism [110, 139]. Because of the distinctive phenotype, some authors have suggested classifying this condition separately from Klinefelter syndrome [139, 148].
Mild to moderate intellectual disability (mental retardation) is seen in most patients, although reported IQs range from 20 to 78. Language development is most severely impaired with some patients having speech aphasia [148]. Behavior has been described as timid, shy, pleasant, anxious, and irritable [110]. Testosterone replacement therapy has been found to be beneficial in some patients, with improvement in attention and behavior [149]. Recent studies have suggested that there may be less significant cognitive impairment in these individuals than previously thought [150]. In 13 children aged 2–7 years, the mean nonverbal IQ was 87.1 [139].
49,XXXYY
Only five cases of live-born males with this sex chromosome disorder have been described. Physical features include tall stature, dysmorphic facial features, gynecomastia, and hypogonadism. All have had moderate to severe mental retardation, with passive but occasionally aggressive behavior and temper tantrums. One patient had autistic-like behavior [110].
47,XY,i(X)(q10)
There have been reports in the literature of 19 individuals with Klinefelter syndrome with an isochromosome Xq or i(Xq) [151]. The phenotype is very similar to typical Klinefelter syndrome with azoospermia, hypogonadism, and gynecomastia, but height is in the normal range, presumably due to a normal copy number of the pseudoautosomal region on Xp/Yp, including the SHOX gene. Using microdissection, Hockner et al. demonstrated the i(Xq) chromosome observed in their patient was an isodicentric, maternally derived, true isochromosome and not the result of an Xq;Xq translocation [151]. These findings are consistent with the proposed mechanism of origin for most of the i(Xq) chromosomes observed in Turner syndrome females (see section “Isochromosome X” above).
Origin of Extra Chromosomes
The extra chromosomes in polysomy X syndromes most likely arise from sequential nondisjunction events during either maternal or paternal gametogenesis. Studies using polymorphic microsatellite DNA markers have shown a maternal origin of extra X chromosomes in 30 cases of 49,XXXXY and 49,XXXXX [115, 116, 157, 159–162, 165–168]. There does not appear to be a maternal age effect as is seen for 47,XXX and 47,XXY. Two cases of 48,XXYY have been shown to arise from paternal nondisjunction [116, 162].
For cases of 48,XXXY studied with Xg blood groups or other polymorphic markers, two are maternal and five are paternal in origin [45, 116, 146, 159–164]. Nondisjunction at first and second meiotic divisions is proposed versus fertilization of an ovum by an XY sperm followed by postzygotic nondisjunction, because mosaicism has not been detected in these patients [116].
47,XYY
Origin
One in 800–1,000 males has an extra Y chromosome [92]. This arises through nondisjunction at paternal meiosis II.
Phenotype
Males with 47,XYY tend to have normal birth length and weight, but when older, most are above the 75th percentile in height. Minor anomalies are found in 20% of patients, but the rate of major malformations is not increased. Most infants are normal in appearance [152]. Pubertal development is usually normal, although onset of puberty in one group of patients studied was approximately 6 months later than average for males with no sex chromosome disorder. Patients often have severe facial acne, are described as tall and thin, and have good general health. Sexual orientation is typically heterosexual. Individuals are described as somewhat awkward and have minor neuromotor deficits [98]. Most have normal fertility and are able to father children. It has been estimated that only 12% of men with XYY are ever diagnosed. Half of those identified were karyotyped because of developmental delay and/or behavior problems [30].
Development
Intelligence is normal, although there is an increased incidence of learning disabilities. There have been two groups of patients with 47,XYY studied long term: one diagnosed in a newborn screening program and the second diagnosed prenatally. The latter group of patients comes from families with an above-average socioeconomic status. The first group had an IQ range of 93–109, and all required part-time special education. The second group had an IQ range of 109–147, and all were reported to be getting “A”s and “B”s in school. IQ is usually somewhat lower than in siblings [98, 108]. Most older boys attend college or have jobs after high school. Longer follow-up of the boys in the second group found that five of 14 required extra assistance in school for academic difficulties and two were in special education programs for the learning disabled [109]. Overall, school performance in this group was above average. IQ scores were available for six of the boys and ranged from 100 to 147. Most were involved with sports, although five were described as poorly coordinated. Two had serious emotional problems. Five had no academic or behavior problems. In general, there was wide variability of development and adaptation [109].
Delays in speech and language development occur in some boys. An increased rate of autism spectrum disorder in boys with XYY has been reported [153]. In a systematic literature review, Leggett et al. found that although males with XYY had lower IQ scores than expected for their social background, they were not impaired in relation to general population norms. Although some have problems with reading, others report reading as a relative strength, and some are noted to have proficiency in science and mathematics [105].
Hyperactive behavior, distractibility, temper tantrums, and a low frustration tolerance are reported in some boys in late childhood and early adolescence. Aggressiveness is not common in older boys. Although early studies raised the possibility of an increase in criminal behavior in these individuals, recent studies have shown that although there is a higher percentage of males with 47,XYY in prisons than in the general population, there was not an increase in violent behavior in these individuals [154]. Differences in rates of incarceration may be due to the lower intelligence of some XYY males. A study of a newborn cohort of XYY males followed into adulthood reported a higher proportion with antisocial behavior than their peers, but there was no statistical difference when diagnostic criteria for antisocial behavior disorder was used and compared with a group of unaffected males [105, 155].
The condition is clearly variable. Most blend into the population as normal individuals. Better outcomes seem to be associated with a supportive, stable environment.
Variants with Additional Y Chromosomes
(See also the section “48,XXYY” presented earlier).
48,XYYY
49,XYYYY
Five nonmosaic cases of 49,XYYYY have been reported. Facial features include hypertelorism, low-set ears, and micrognathia. Skeletal abnormalities include clinodactyly, radioulnar synostosis, scoliosis, and spina bifida occulta. Mental retardation and speech delay, along with impulsive and aggressive behavior, were reported [110, 158–162]. In a patient followed from age 4 months to 26 years, adult height was at the 80th percentile, walking began at 2 years, and IQ at age 7 years was 75, but he was described as having mild to moderate mental retardation as an adult with impulsivity, behavioral disturbance, and difficulties with social interaction [162].
Sex Chromosome Aneuploidy and Age
Increased aneuploidy with advancing age was first reported by Jacobs et al. in 1961 [169]. This was subsequently found to be the result of the loss of the X chromosome in females and of the Y in males [170]. Premature centromere division in the X chromosome and loss through anaphase lag and formation of a micronucleus are one proposed mechanism in females [171, 172]. This is supported by the finding that hyperdiploidy for the X is much less common than monosomy, which would not be expected if nondisjunction was the mechanism [173]. It is usually the inactive X chromosome that is missing in monosomy X cells [174]. Another proposed mechanism is telomere shortening, as telomeres play a key role in chromosome segregation [175]. X-chromosome aneuploidy is not observed in bone marrow preparations from older women but is seen in phytohemagglutinin (PHA)-stimulated peripheral blood lymphocytes. Although some early studies suggested an increase in sex chromosome aneuploidy in women with a history of reproductive loss, recent studies have shown that this is probably not true and that it is purely a phenomenon of aging [173, 176].
In a prospective study of 11 women from 83 to 100 years of age, Jarvik et al. found a fourfold increase in X-chromosome loss after 5 years, compared with the initial level [177]. Galloway and Buckton found a tenfold increase in X-chromosome aneuploidy in women aged 25–35 years compared with those between 65 and 75 years [170]. Between 30 and 55 years, the rate of hypodiploid cells was 3–5% in females, increasing to 8% at age 70 years. This holds true for the loss of any chromosome, but the most common was loss of an X chromosome. It should be noted that there is variability of sex chromosome loss between individuals of the same age, so that what is “normal” aneuploidy at a specific age is impossible to predict. This makes it difficult to interpret the clinical implications of X-chromosome loss seen in an older woman who can also have features of Turner syndrome such as ovarian failure and/or infertility. Because the age-related loss is limited to peripheral blood lymphocytes, analysis of other tissues such as skin fibroblasts or buccal cells can be helpful in clarifying these situations. Russell et al. studied metaphase cells from cultured peripheral blood lymphocytes in 655 females ranging in age from birth to 80 years and found that below age 16 years there is 0.07% X-chromosome loss and above age 65 years there is 7.3% X-chromosome loss. The authors provide their data as a reference graph to illustrate normal percent X-chromosome loss based on number of cells counted (ranging from 30 to 60 cells) [178].
Age-associated loss of the Y chromosome in men is found more often in bone marrow than in peripheral blood, and it approaches the rate generally seen in peripheral blood in women [173]. Studies of bone marrow preparations have shown that Y-chromosome loss was restricted to males over age 40–50 years, with a frequency of 8–10% of cells [179, 180]. For men of varying ages with no hematological malignancies, 7.7% had Y-chromosome loss; it was rare below age 50 years but was found in 20% of men above 80 years of age. The percentage of Y-negative cells ranged from 10 to 100% [180].
Most studies comparing age-related sex chromosome aneuploidy were done on metaphase preparations and are, therefore, at risk for preparation aneuploidy. Guttenbach et al. performed an in situ study of lymphocyte interphase nuclei to look at sex chromosome loss and aging [181]. In males, the rate was 0.05% up to age 15 years, 0.24% in 16- to 20-year-olds, and then steadily increased to 1.34% at age 76–80 years. The mean value of monosomy X cells in females was 1.58% in 0- to 5-year-olds and increased to 4–5% in women over 65 years. Only women over 51 years of age showed a distinct age correlation. This study also found no difference in aneuploidy rates between cultured and noncultured cells [181]. Bukvic et al. performed analysis of sex chromosome aneuploidy in interphase cells of 16 centenarians and found loss of Y signal in 10% of cells in males compared to 1.6% of cells in younger control men and loss of X signal in 22% of females compared to 1.7% of cells in young women [182].
These findings should be considered when analyzing peripheral blood chromosomes in older females and bone marrow from older men, in order to avoid misinterpretation of normal age-related aneuploidy as clinically significant mosaicism or acquired changes.
Structural Abnormalities of the X Chromosome
In addition to the isochromosome Xq commonly found in patients with Turner syndrome, the X chromosome can be involved in translocations, both balanced and unbalanced, and can also have deletions and duplications (see Chap. 9).
Structural abnormalities of the X in males are generally associated with more severe phenotypic manifestations than in females. This is partly explained by preferential inactivation of the structurally abnormal X in cases of duplications or deletions or in unbalanced X;autosome translocations in females. In cases of balanced X;autosome translocations, there is usually preferential inactivation of the normal X chromosome. Theories explaining this are discussed in the following paragraphs. “High-resolution” chromosome analysis should be performed on females manifesting X-linked disorders to look for a structural X abnormality. Chromosome microarray can also be helpful to look for X-chromosome imbalance (see Chap. 18).
The molecular X-inactivation pattern seems to correlate with phenotype in women with structural abnormalities of the X chromosome. Completely nonrandom X inactivation of the abnormal X is generally associated with a normal phenotype, whereas those with skewed or random inactivation patterns usually have nonspecific intellectual disability (mental retardation) and/or congenital abnormalities. The X-inactivation status of women with structurally abnormal X chromosomes and an abnormal phenotype should be assayed as part of a routine clinical workup. The phenotype could be correlated with differences in X-inactivation ratios [183]. There have been very few reports on the use of prenatal X-inactivation studies in amniotic fluid or CVS [184] (see also Chap. 12). Studies comparing prenatal and postnatal analysis of X inactivation and their correlation with phenotypic and developmental outcomes are needed before these could be used to give prognostic information in female fetuses with X-chromosome abnormalities.
X;Autosome Translocations
Balanced Translocations
In females, balanced X;autosome translocations can be divided into four phenottypic categories: normal phenotype with or without history of recurrent miscarriage, gonadal dysfunction with primary amenorrhea or premature ovarian failure (POF), a known X-linked disorder, or congenital abnormalities and/or developmental delay [185]. The reasons for the variable phenotypes are complex and not fully understood, making genetic counseling in cases of prenatal detection of these translocations very difficult.
Translocations involving the X chromosome and an autosome often lead to primary or secondary ovarian failure and sometimes Turner syndrome-like features if the translocation occurs within the critical region of Xq13-q26 [186–188]. There are several different hypotheses concerning the cause of gonadal dysfunction in these cases, including disruption of POF-related genes, a position effect resulting from local alteration of chromatin caused by the translocation, and incomplete pairing of X chromosomes at pachytene [186–190]. In cases in which the derivative X chromosome is inactive, inactivation will spread from the translocated X segment to the attached autosomal material, where it will inactivate genes. The other X-chromosome segment will remain active. There is incorrect dosage of both autosomal and X-linked genes in these cells, with functional autosomal monosomy for the derived (X)t(X;autosome) chromosome that contained the X-inactivation center and functional X-chromosome disomy for the portion of the X chromosome translocated onto the active (autosomal) reciprocal translocation product [191]. There is strong selection against such cells. In general, the normal X is preferentially inactivated in approximately 75% of such patients [191, 192]. When the translocation disrupts a gene located on the X chromosome, a female with such a translocation could manifest a disease condition [193, 194]. Any mutated genes on the derivative X chromosome will be fully expressed, as they would be in a male [5]. Several X-linked genes have been mapped in this way.
A “critical region” determining normal ovarian function has been hypothesized at Xq13-Xq26 [188, 195]. The majority of females with balanced translocations with breakpoints in this region usually have premature ovarian failure (secondary amenorrhea associated with elevated gonadotropin levels before the age of 40 years). Although there have been several candidate genes for POF identified in this region, molecular characterization of the translocation breakpoints of women with balanced translocations involving the critical region has often shown no gene disruption [196, 197]. This supports the hypothesis that a position effect secondary to chromatin alteration or pairing abnormalities at meiosis causes ovarian dysfunction.
It has been thought that the majority of females with balanced X;autosome translocations with breakpoints above the X-inactivation center at Xq13 are phenotypically normal [198, 199]. Schmidt and DuSart found that most X;autosome translocation patients with phenotypic abnormalities or developmental delay had breakpoints clustered in the subtelomeric bands Xp22 and Xq28 [191]. This was thought to be the result of persistence of cells with inactivation of the translocated X in these patients. However, in a study by Waters et al. that reported 104 cases ascertained from cytogenetics laboratories in the United Kingdom, female X;autosome translocation carriers had a significantly higher number of abnormalities, including developmental delay and learning problems, than would be expected from literature review [185]. Those with congenital anomalies and/or developmental delay showed random X-chromosome breakpoint distribution. De novo translocations were significantly more likely to be associated with an abnormal outcome (18 of 19 cases), suggesting that de novo status versus breakpoint location is the most important risk factor in predicting phenotypic outcome [185].
Some studies have indicated that in those patients with phenotypic and/or developmental abnormalities, the translocated X was late replicating (inactive) [200]. However, Waters et al. did not find an association with aberrant late replication and abnormal phenotype. Eight of their patients showed a deviation from the expected pattern of consistent early replication of the derived X chromosome and late replication of the normal X chromosome. Five of these patients were phenotypically normal [185].
Because of variability in X inactivation from one person to another with the same X;autosome translocation, it is possible for a phenotypically normal mother to have a daughter with phenotypic abnormalities and intellectual disability (mental retardation) even though both carry the same such rearrangement. This can be because of skewed (nonrandom) X inactivation in the former and random X inactivation in the latter, leading to functional X disomy and functional autosomal monosomy in some cells. This is estimated to occur in approximately 25% of females with X;autosome translocations [193].
A fertile woman with a balanced X;autosome translocation is at risk for having offspring with an unbalanced rearrangement (Fig. 10.5). There is also the risk that even balanced offspring could be abnormal as a result of random or skewed inactivation of the abnormal X in a female child or by disruption of a functional gene on the X in a male. The risk for a female with a balanced X;autosome translocation to have a live-born child with a structural and/or functional aneuploidy has been estimated at 20–40% [192]. Phenotypic abnormalities can range from mild effects to severe intellectual disability (mental retardation) and birth defects.


Fig. 10.5
Balanced reciprocal translocation between the short arms of chromosomes X and 3: 46,X,t(X;3)(p11.3;p21.2). Brackets indicate regions involved in translocation on the derivative chromosomes. The patient was a 30-year-old clinically normal, fertile female who had a daughter with an unbalanced translocation consisting of a normal X, the derivative X, and two normal chromosomes 3 (partial monosomy Xp and partial trisomy 3p)
Males with balanced X;autosome translocations are usually phenotypically normal but almost all are infertile [201] (see also Chap. 11). There have been reports of severe genital abnormalities in males with such translocations and of multiple congenital anomalies in a man with an apparently balanced (X;6) translocation inherited from his mother [202, 203]. As noted earlier, there is also a risk of an X-linked recessive disorder because of disruption of a gene by the translocation.
Further characterization of breakpoint regions in X-chromosome rearrangements has revealed that although some interrupt specific genes, others are in gene-poor regions. X-linked gene interruption is not a common finding in patients with POF and X;autosome translocations. Two critical regions on Xq associated with POF have been proposed. Critical region I at Xq21 has a low meiotic recombination frequency, is gene poor, is high in repetitive sequences, and is close to the X-inactivation center [204]. Translocations in this region could cause POF as a result of a position effect of autosomal genes important in ovarian function or as a result of meiotic pairing abnormalities [205, 206]. Deletions in this region rarely cause POF. Critical region II, at Xq23-q28, has different properties. Translocations involving this region could cause POF because of a position effect of X-linked genes. Since deletions in this region are frequently associated with POF, haploinsufficiency for missing or interrupted genes in this region could be the cause.
Unbalanced Translocations
In females with unbalanced X;autosome translocations, the abnormal X is generally inactive if the X-inactivation center is present, probably secondary to selection against cells with an autosomal imbalance and functional X disomy. If the X-inactivation center is not present in the translocated segment, phenotypic abnormalities usually result from such imbalances and can include intellectual disability (mental retardation) and multiple congenital anomalies [207]. There have also been patients described who have unbalanced X;autosome translocations but no phenotypic abnormalities and only mild behavioral problems [208].
Earlier studies relied on replication timing to investigate inactivation in X;autosome translocations. The translocated autosomal material can become delayed in its replication timing, and this has been used to examine the extent of the spread of X inactivation in such cases. It has recently been demonstrated that late replication is a poor correlate of the spread of gene silencing [209]. The spreading of late replication is often incomplete and might skip some autosomal bands and affect others [210]. This suggests that autosomal chromatin does not transmit or maintain the inactivation signal as efficiently as the X chromosome [209].
Sharp et al. reported a family with both a balanced and unbalanced (X;10) translocation segregation [209]. A female with the unbalanced form was phenotypically normal except for secondary amenorrhea. Although the derivative X chromosome was late replicating, the late-replicating region extended only to the X;autosome boundary and did not appear to spread into the translocated segment of 10q. However, transcriptional analysis showed that the translocated segment of 10q was mostly inactive, consistent with the phenotype of the patient. There have been several other reports of patients with X;autosome translocations with mild phenotypes in which no spreading of late replication into the attached autosome was observed [211, 212]. This suggests that silencing of autosomal genes by X inactivation can occur without apparent delay in the replication timing of the surrounding chromatin. The use of replication-timing studies to evaluate the extent of spread of inactivation in X;autosome translocations can be misleading and should not be used to make predictions of phenotype [209]. In a study of five cases with X;autosome translocations, there appears to be some correlation between the pattern of gene silencing and clinical phenotype [15]. However, use of such techniques for prognostic purposes on a clinical basis awaits further studies. Cytogenetic features such as depletion of histone acetylation and H3 lysine 4 dimethylation provide more reliable indicators of the extent of spread of X inactivation than replication-timing studies [15].
Prenatal detection of an unbalanced X;autosome translocation presents a difficult genetic counseling problem (see Chap. 21). Although there have been reports of affected females having only secondary amenorrhea or mild developmental delay, many have had a more severe phenotype with mental retardation and birth defects [15, 200, 209, 213].
In males with unbalanced X;autosome translocations, there is in utero lethality or, if they survive, multiple congenital anomalies and intellectual disability (mental retardation) [214].
Functional disomy for the distal long arm of the X caused by unbalanced X;autosome translocations involving Xq28 appears to cause a distinctive phenotype [215] (see also the section on “Xq Duplications” later).
Deletions of Xp
Males with deletions of the short arm of the X show contiguous gene syndromes characterized by different combinations of phenotypes, depending upon the location and length of the deletion [216]. X-linked ichthyosis, Kallmann syndrome (anosmia and hypogonadism), intellectual disability (mental retardation), and chondrodysplasia punctata (skeletal dysplasia) are seen in males with deletions involving distal Xp. Deletions in Xp21 cause a contiguous gene syndrome of Duchenne muscular dystrophy, retinitis pigmentosa, adrenal hypoplasia, mental retardation, and glycerol kinase deficiency [217]. Larger Xp deletions in males are lethal.
In females, there is usually preferential inactivation of the structurally abnormal X when the deletion is in or proximal to Xp22.1. In those with breakpoints in Xp22.3, the normal and abnormal X can be active in various proportions of cells [218]. Females with Xp deletions do not usually manifest any of the recessive disorders due to presence of a normal X chromosome, although almost all have short stature and some have phenotypic features of Turner syndrome. Short stature in these patients is likely the result of haploinsufficiency for the SHOX gene, within the pseudoautosomal region on Xp [56, 219]. Turner syndrome features could include variable skeletal anomalies associated with SHOX deletion and soft tissue anomalies such as nuchal webbing and low posterior hairline reported in some patients with Xp11.1 terminal deletions possibly related to a proposed lymphedema critical region in Xp11.4 [43, 59].
Females with terminal deletions at Xp11.1 usually have complete ovarian failure, although in a series reported by Ogata et al., almost 50% of those with deletions in this region had spontaneous puberty and one had fertility [220]. Females with terminal deletions originating at Xp21 are more likely to show premature rather than complete ovarian failure, although they may have normal fertility [220, 221]. The phenotypes associated with Xp deletions can vary, even within the same family [222]. This is most likely due to variable X inactivation and modifying genes.
Studies have shown that most de novo Xp deletions originate on the paternal chromosome [218]. Uniparental disomy (UPD; see also Chap. 20) for the deleted and nondeleted X chromosomes was not found in a study of 25 females with Xp deletions [218].
Interstitial deletions of Xp in females can have intrafamilial phenotypic variability, even when the involved genes escape X inactivation [223]. The cause of this variability is not known.
Deletions of Xq
Large Xq deletions in males are not compatible with survival. Smaller deletions are associated with severe phenotypes [224].
Deletions of the long arm of the X lead to variable phenotypic outcomes in females. Forty-three percent of women with Xq deletions have short stature [225]. In a study by Geerkens et al., it was found that women with breakpoints in Xq13 to Xq25 had both average and short stature, suggesting variable inactivation of growth genes in Xp or proximal Xq [226]. Deletions in various regions of the long arm are sometimes associated with gonadal dysgenesis or POF. Females with terminal deletions originating at Xq13 are more likely to have complete ovarian failure, whereas those with deletions at Xq24 might have POF [221]. In a series of women with Xq deletions ranging from Xq13.3 to Xq27 reported by Maraschio et al., seven of eight patients had secondary amenorrhea. One woman with deletion at Xq27 had fertility and menopause at 43 years [227]. Clinical features of Turner syndrome are less common in Xq as compared to Xp deletions but more common in patients with deletions proximal to Xq25 [227, 228]. The likelihood of an abnormal phenotype in a female with an Xq deletion is low, although primary or secondary ovarian failure is likely. Recent studies using molecular techniques to characterize breakpoints in Xq deletion cases have reported that normal fertility is more likely if the deletion is in critical region I (Xq21) but most women with deletions in critical region II (Xq23-Xq28) have ovarian failure [205]. Women with Xq deletions and fertility should be advised about their 50% risk of passing the abnormal X to male offspring, with likely miscarriage or severe phenotype depending on the size and location of the deletion.
Xp Duplications
Duplications of Xp involving bands p21.2–21.3, plus a Y chromosome, have been reported in patients who were phenotypic females, suggesting a sex-determining gene locus on Xp [229, 230]. These patients also had mental retardation and multiple anomalies. This area of the X has been termed the dosage-sensitive sex reversal (DSS) region (see the section “46,XY Disorders of Sex Development” later). Dosage-sensitive sex reversal is the result of duplication of the DAX1 gene, which, when deleted or mutated, leads to congenital adrenal hypoplasia [231]. Males with duplications involving more distal Xp have been reported with mental retardation and autism but without sex reversal [232].
Both normal and abnormal phenotypes, and normal fertility as well as amenorrhea, have been reported in females with Xp duplications and one normal X chromosome [229, 230, 233]. The abnormal phenotype—including Turner syndrome features, short stature, seizures, and amenorrhea, but normal intelligence—was seen in a female with complete inactivation of the duplicated X, suggesting that random inactivation was not the cause [233]. An interstitial duplication at Xp11.1-p21.2 was reported in a female with macrocephaly, cleft lip, hypertelorism, and other dysmorphic features who died at age 2 months. There was random X-chromosome replication pattern in this patient [234]. In a review of 52 females with partial X duplications involving Xp or Xq, Matsuo et al. found that random or skewed but not completely selective X inactivation occurred in nine of 45 patients examined for X-inactivation pattern, independent of the size or location of the duplicated segments [235]. For Xp duplications, four of six patients with random or skewed X inactivation had an apparently normal phenotype, and three of 12 patients with selective inactivation of the duplicated chromosome had clinical abnormalities [235].
A dicentric inverted duplication of most of the short arm of the X [dic dup(X)(qter→p22.3::p22.3→cen)] has been reported in a mother and daughter with short stature, mental retardation, and dysmorphic features. The mother had the duplicated X as the inactive X in all cells, but the daughter had the duplicated X active in 11% of lymphocytes [236].
Females with duplications of Xp including the SHOX gene region have been reported with tall and normal stature [237, 238].
With the increasing use of chromosomal microarray technology (see Chap. 18), recurrent microduplications associated with variable phenotypes are being delineated and characterized. A recurrent microduplication of Xp11.22-p11.23 has been reported in association with intellectual disability, speech delay, and electroencephalogram (EEG) abnormalities in both males and females [239]. The size of the duplication ranges from 0.8 to 9.2 Mb. Other clinical features include a hoarse or nasal voice, early puberty, and obesity. X-inactivation analysis of affected females showed selective inactivation of the normal X chromosome in six of nine subjects and random inactivation in the other three. The degree of X inactivation did not correlate with clinical phenotypic severity within families. A female with a de novo duplication of Xp11.22-p11.4 has been reported with intellectual disability and structural brain anomalies. X-inactivation studies in peripheral blood lymphocytes showed that the duplicated X was active in the majority of cells [240]. This is a gene-rich region, with numerous genes associated with X-linked intellectual disability, and all genes in this region typically undergo X inactivation, which may account for the abnormal phenotypes of both sexes even with relatively small duplications [9].
Duplication of the region from Xp21-p22 may also be of clinical significance and related to developmental delay and intellectual disability in both males and females along with variable dysmorphic features, including genital anomalies and seizures in a male [241].
An interstitial microduplication of Xp22.31, ranging in size from 149 kb to 1.74 Mb, and including the steroid sulfatase gene has been found with a frequency of 0.15% in a healthy control population and 0.37% in a cohort of individuals with abnormal phenotypes that included developmental delay, intellectual disability, autism, dysmorphic features, and/or multiple congenital anomalies. The most frequent clinical features observed in the abnormal group were intellectual disability, hypotonia, failure to thrive/feeding difficulties, and seizures. Eight phenotypically normal females tested had random or preferential inactivation of the duplicated X as compared to those with an abnormal phenotype in whom the duplicated X was preferentially active in two of seven, preferentially inactive in one, and there was random X inactivation in four [242]. Whether microduplication of Xp22.31 is clinically significant and related to intellectual disability or is a benign copy number variant remains unclear.
Xq Duplications
Males with duplications of the long arm of the X usually have significant intellectual disability (mental retardation) and birth defects due to functional disomy of the duplicated regions. Most females with Xq duplications have normal phenotypes and are ascertained after the birth of an abnormal male child. However, there have been females with phenotypic abnormalities including short stature, microcephaly, developmental delay/mental retardation, and gonadal dysgenesis reported. Reasons for this variability may be the size or location of the duplicated segment, random or nonskewed X inactivation, duplication of dosage-sensitive genes and genes that normally escape inactivation, incomplete inactivation of a portion of the duplicated segment, or an imprinting effect [243, 244] (see also Chap. 20).
In a review of Xq duplications, phenotypically normal females had smaller and more proximal duplicated Xq segments compared to the Xq duplications in females with clinical abnormalities [243]. In a review of Xq duplications, Zhang et al. also reported that the duplicated segments in individuals with abnormal phenotypes were more frequently located in proximal Xq [244]. A review by Matsuo et al. showed that normal phenotypes are more commonly associated with smaller and more proximal duplications of Xq, and abnormal phenotypes tend to have larger and more distal duplications, but that there is a great deal of overlap [235].
Goodman et al. reported three families with duplication of Xq27-qter on the short arm of the X [245]. Affected males had mental retardation and minor anomalies. The abnormal chromosomes were inherited from the mothers, who were phenotypically normal. Replication studies in two of the mothers showed the abnormal X to be late replicating. However, most phenotypically abnormal females have also been reported to preferentially inactivate the abnormal X chromosome [246]. Therefore, both normal and abnormal phenotypes can be seen even when there is preferential inactivation of the abnormal X. One of three patients with random or skewed X inactivation had an abnormal phenotype, and 9 of 22 cases with selective inactivation of the duplicated X had an abnormal phenotype [235]. The reason for the variable phenotypes but similar inactivation patterns could be the result of differential patterns of inactivation along the chromosome. The activation status of the material present in excess copy number might be what differentiates females with normal phenotypes from those with abnormal phenotypes. The functional disomy of genes might affect the phenotype [246].
Replication studies cannot distinguish phenotypically normal and abnormal females with Xq duplications [233, 243]. Correlations of X-inactivation pattern and phenotype in patients with small duplications should be interpreted carefully [183].
Recurrent Xq duplications identified through chromosome microarray have been reported for Xq25 and associated with hemihyperplasia and digital anomalies and also for Xq27 involving the SOX3 gene causing pituitary failure in males [247, 248]. Xq28 duplications involving MECP2 cause the Lubs X-linked mental retardation syndrome (MRXSL) in males [249, 250]. A different condition involving copy number gains at Xq28 has also been reported that involves not MECP2 but FLNA and GDI1, with severity correlating with number of additional copy numbers and causing nonsyndromic intellectual disability [251]. Sanlaville et al. have reviewed distal Xq duplications [252].
Inversions of the X Chromosome
Paracentric Inversions
Paracentric inversions of the X chromosome (Fig. 10.6) are relatively rare. There has been a wide range of phenotypes described. In general, when long-arm paracentric inversions involve the critical region at Xq13-26, females have some degree of ovarian dysfunction [253]. When the inversion is outside the critical region, normal phenotype and fertility have been reported, although there are exceptions to this [254, 255]. There has also been variability in mental function in females, with some having mental retardation and others with normal intelligence, even in the same family [256]. Males can be phenotypically normal or have mental retardation [255, 257]. Fertility in males is also variable [258, 259].


Fig. 10.6
Distal paracentric inversion of Xq: inv(X)(q26q28) in a woman with normal phenotype and fertility. Brackets indicate region involved in inversion
Pericentric Inversions
Most females with pericentric inversions of the X have normal phenotypes and fertility [260–264]. However, pericentric inversions of the X have been reported in females with gonadal dysgenesis and with mental retardation [261]. Keitges et al. reported dizygotic twins with the same pericentric X inversion [inv(X)(p11;q22)] [261]. One twin was phenotypically normal with normal intelligence and menses and had random X inactivation. The other was mildly mentally retarded and had psychiatric problems, irregular menses, minor anomalies, and selective inactivation of the inverted X. Proposed explanations for these findings include different normal Xs, a nondetectable deletion or duplication in the abnormal twin, or chance. This also raises the likelihood that the replication pattern of the inverted X is a better predictor of fertility than the breakpoints. Interestingly, females with random X inactivation are more likely to have normal fertility than those with skewed inactivation of the inverted X [261]. Offspring of females with pericentric inversions are at risk for inheriting a recombinant chromosome with associated phenotypic abnormalities [260, 263–265].
Most males with inherited pericentric inversions of the X have a normal phenotype and fertility [260, 265, 266]. However, X-linked disorders have been found to segregate with pericentric inversions of the X, presumably by disruption or deletion of a gene by the inversion [267–270].
Analysis of X-chromosome inactivation in women with apparently balanced pericentric inversions might determine whether an imbalance is present at the molecular level. Random inactivation is usually associated with a balanced inversion, whereas skewed inactivation is more likely associated with an unbalanced inversion [266, 268]. Inactivation status of the mother might provide helpful information in cases of prenatal detection of a male fetus with a maternally inherited inversion [271]. Chromosome microarray is also helpful (see Chap. 18).
Isodicentric X Chromosomes
Isodicentric Xp Chromosomes
Isodicentric X chromosomes are formed by the fusion of two X chromosomes [272]. The phenotypic effects are variable and dependent on whether the chromosomes are fused at long or short arms, as well as on the location and extent of the deletion. The isochromosomes that are composed of two copies of the entire short arm [i(Xp)] are rare and have only been reported in females; they are believed to be nonviable in male conceptions [273]. These i(Xp) chromosomes are typically pseudodicentric with one active and one inactive centromere, and the XIST locus is retained in at least one of the deleted long arms [273, 274]. Most of these females have gonadal dysgenesis that could be explained by the mosaicism for a 45,X cell line that is often present, loss of critical regions on Xq, and/or meiotic pairing abnormalities. Emotional and behavioral problems and intellectual disability have been reported in these patients [273]. Stature ranges from tall to short. Tall stature could be related to the presence of three copies of SHOX in those patients with long arms joined.
Isodicentric Xq Chromosomes
Isodicentric X chromosomes consisting of two copies of the long arm joined at the proximal short arms with two centromeres are the most common structural abnormality of the X chromosome seen in Turner syndrome. Although most look monocentric in a G-banded karyotype, they are actually dicentric with one active centromere. The correct nomenclature (in the absence of direct evidence of a dicentric chromosome) is 46,X,i(X)(q10), with the understanding that in many instances it would be more accurate to use 46,X,idic(X)(p11.2).
Females with isodicentric X chromosomes joined at their short arms exhibit short or normal stature, gonadal dysgenesis, and, occasionally, Turner syndrome features [275]. Explanations for the phenotype of short stature when the short arms are joined include deletion of the distal short arm at the region of SHOX and/or as a result of a 45,X cell line. There are some males with Klinefelter syndrome who have one normal X and one isochromosome X [276] (see also the previous section on “47,XXY (Klinefelter Syndrome)”).
Mechanisms to explain formation of terminal rearrangements between homologous chromosomes include the following:
1.
Breakage and deletion of a single chromosome followed by rejoining of sister chromatids
2.
Breakage and deletion of two homologous chromosomes at the same breakpoints followed by interchromosomal reunion
3.
Terminal fusion without chromatin loss between sister chromatids and homologous chromosomes [277]
The isodicentric X is almost always late replicating, suggesting nonrandom inactivation of the derivative X. The second centromere is usually nonfunctional, making it a pseudodicentric chromosome [278] (see also the subsections “Turner Syndrome,” “Isochromosome X” earlier). Studies have shown that those that are functionally dicentric tend to have anaphase lag and, therefore, are more likely to be found in association with a 45,X cell line. Those that are functionally monocentric tend to segregate normally in mitosis [278, 279]. The occurrence of a 45,X cell line also correlates with the distance between the centromeres, presumably due to less mitotic stability of the isodicentric chromosome [75]. Most are thought to be derived from exchanges between sister chromatids. Analysis of breakpoints in cell lines with idic(X)(p11.2) has found that they lie within large inverted repeat sequences associated with low copy repeats or highly repetitive elements on Xp11.2, suggesting that the principal mechanism in their formation involves nonallelic homologous recombination. The Xp11.2 region appears to be susceptible to rearrangements leading to isochromosome formation and other rearrangements [280].
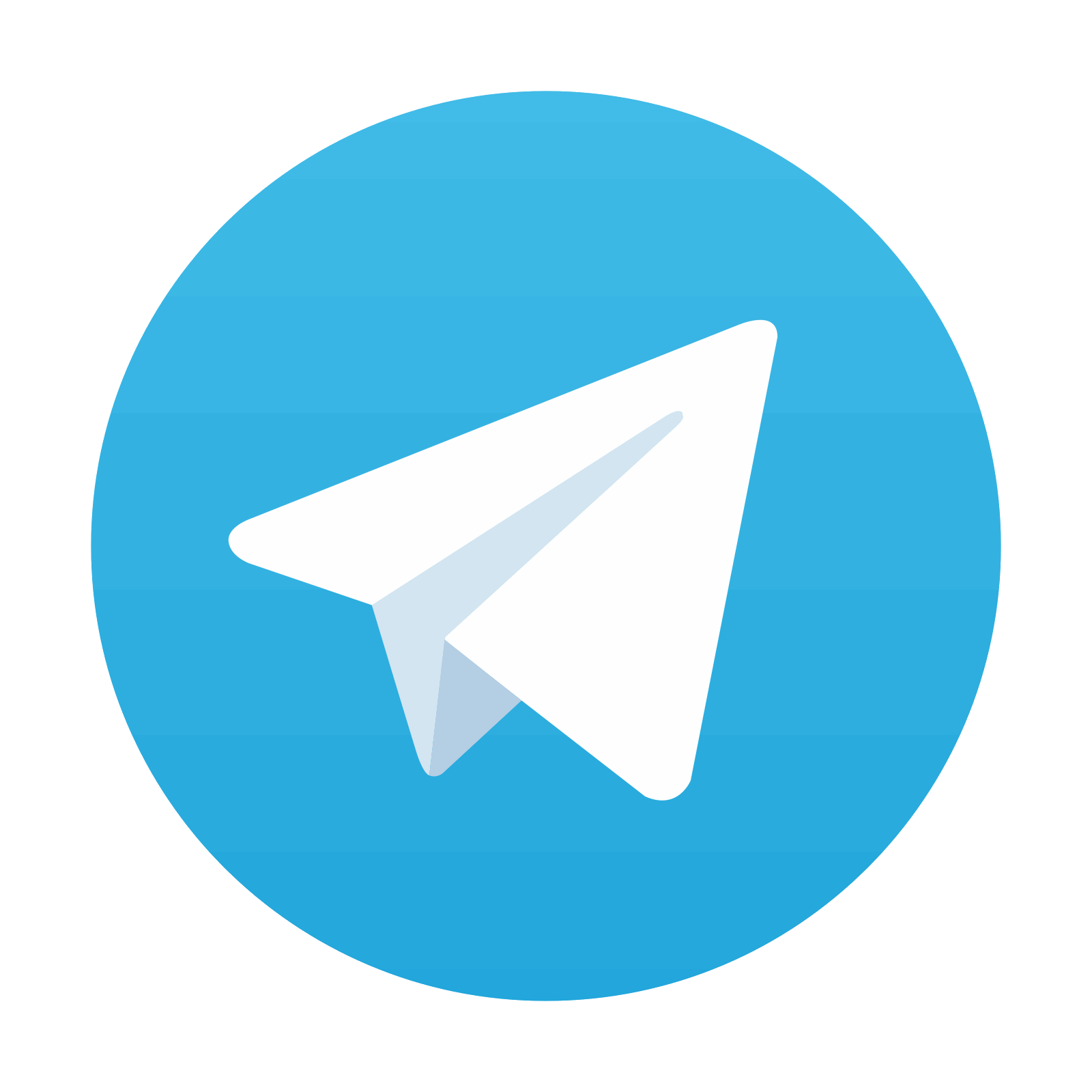
Stay updated, free articles. Join our Telegram channel

Full access? Get Clinical Tree
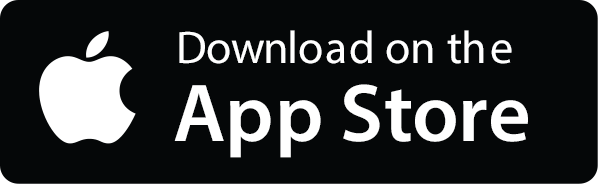
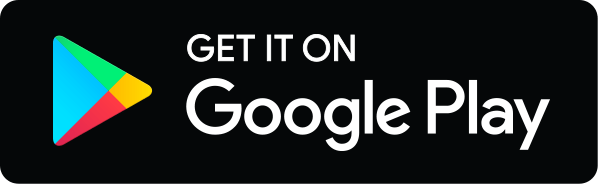