CHAPTER 5 The Cervical Region
The cervical region is possibly the most distinct region of the spine. The fact that so many structures, spinal and otherwise, are packed into such a small cylinder, connecting the head to the thorax, makes the entire neck an outstanding feat of efficient design. The cervical spine is the most complicated articular system in the body, comprising 37 separate joints (Bland, 1989). It allows more movement than any other spinal region and is surrounded by a myriad of nerves, vessels, and many other vital structures. However, such complexity can come at a high cost; more than 50% of individuals suffer from significant neck pain at some time in their lives (SBU Project Group, 2000). All clinicians who have spent significant time working with patients suffering from pain of cervical origin have been challenged and sometimes frustrated with this region of immense clinical importance. Understanding the detailed anatomy of this area helps clinicians make more accurate assessments of their patients, which in turn results in the establishment of more effective treatment protocols.
CHARACTERISTICS OF THE CERVICAL SPINE AS A WHOLE
Cervical Curve (Lordosis)
The cervical curve is the least distinct of the spinal curves. It is convex anteriorly (lordosis) and is a secondary (compensatory) curvature (see Chapter 2). The cervical curve begins to develop before birth at as early as 9 weeks of prenatal life. Onset of fetal movements plays an important role in the early development of the cervical lordosis (Bagnall, Harris, and Jones, 1977; Williams et al., 1995). However, the curve becomes much more marked when the child begins to lift the head at approximately 3 to 4 months after birth, and the curve increases as the child begins to sit upright at approximately 9 months of age (Williams et al., 1995).
The amount of cervical lordosis can be measured reliably from standard x-rays if rigorous, standardized methods of evaluation are used (Côté et al., 1997). The cervical lordosis increases significantly with age in both sexes. In males, the change is rather gradual until the age of 70, when a dramatic increase in lordosis occurs. In females, in whom the cervical lordosis is greater throughout life, the curve increases gradually until a time of several years of marked increase begins near the age of 50; the rate of change then becomes more gradual but continues throughout the remainder of life (Doual, Ferri, and Laude, 1997).
Some authors state that the cervical curve actually is composed of two curves, upper and lower (Kapandji, 1974; Oliver and Middleditch, 1991). The upper cervical curve is described as a distinct primary curve that extends from the occiput to the axis and is concave anteriorly (kyphotic). The lower cervical curve is the classically described lordosis, but in this case begins at C2 rather than C1. This description helps to explain the dramatic differences seen between the upper and lower cervical vertebrae, such as the independent movements that can occur in the two regions (e.g., flexion of the lower cervicals and simultaneous extension of occiput on atlas and atlas on axis).
The lack of a normal cervical lordosis is a frequent finding in children and adolescents under age 17, but a lack of cervical lordosis in the adult may be a sign of ligamentous injury (Fesmire and Luten, 1989) or anterior cervical muscular hypertonicity. However, some controversy exists on this topic. Some authors (G. Schultz, personal communication) consider a hypolordotic or even kyphotic cervical curve to be clinically insignificant, especially if a tendency toward the establishment of a lordosis can be demonstrated during extension of the cervical region. Methods used to evaluate such a tendency include palpation at the spinous processes during extension, lateral projection x-ray films taken with the patient holding the neck in extension, and cineradiography studies in which a series of x-ray films are taken while the patient moves the neck. Another cause of decreased cervical lordosis is the presence of hyperplastic articular pillars (see Articular Processes). Hyperplastic articular pillars are considered to be a common normal variation of the typical cervical vertebrae (C3-6) and are associated with a reduction of the cervical lordosis (Peterson et al., 1999).
Total cerebrospinal (CS) height decreases significantly from early adulthood to senescence in both sexes, but the rate of change is different in males and females. In males, the change is more gradual, whereas in females, in whom the overall decrease in height is greater than in males, there is a marked change near the age of 50, presumably associated with menopause. As described, the cervical lordosis also markedly increases during this time. The rate of decrease in height of the cervical region then slows again after the fifth decade (Doual, Ferri, and Laude, 1997).
Degenerative joint disease (DJD; bone spur, or osteophyte formation, and intervertebral disc narrowing) occurs earlier in males (31 to 40 years of age) than females. However, the incidence of female DJD of the cervical region catches up as a sharp rise in DJD occurs at 51 to 60 years of age. After 60 years of age the incidence between males and females is roughly equal (Wiegand et al., 2003).
Typical Cervical Vertebrae
Vertebral Bodies.
Each cervical vertebra is made up of a vertebral body and a posterior arch (Fig. 5-1). The vertebral bodies of the cervical spine are small and are more or less rectangular in shape when viewed from above, with the transverse (left-to-right) diameter greater than the anteroposterior diameter. The exception to this is C7, which is almost square. The transverse and anterior to posterior diameters both increase from C2 to C7. This allows the lower vertebrae to support the greater weights they are required to carry. In addition, the vertebral bodies of males have been found to be larger than those of females (Liguoro, Vandermeersch, and Guerin, 1994; Lu et al., 1999).
The thickness of the outer cortex of the superior and inferior aspects of the vertebral bodies increases from C3 to C7. In addition, the superior aspect of each C3-7 vertebral body (superior bony end plate) is thickest posteriorly and thinnest anteriorly; the opposite is true for the inferior end plate (i.e., thickest anteriorly). The center of the inferior bony end plate also is thicker than the center of the superior bony end plate in the C3-7 vertebrae. Finally, the heights (superior to inferior dimensions) of both the anterior and posterior aspects of the cervical vertebral bodies generally increase from C3 to C7 (Panjabi et al., 2001a).
The anterior surface of the cervical vertebral body is convex from side to side. However, these same surfaces are concave from superior to inferior (Panjabi et al., 2001a) because of prominent ridges at the superior and inferior borders (discal margins) formed by the attachment sites of the anterior longitudinal ligaments. Indentations seen on the left and right of the anterior midline of the vertebral bodies are for attachment of the vertical fibers of the longus colli muscle.
The anterior aspects of the vertebral bodies can develop bony spurs (osteophytes). Asymptomatic osteophytes may occur in 20% to 30% of the population. Pressure on the more anteriorly located esophagus or trachea from osteophytes may lead to difficulty with swallowing (dysphagia) and speech (dysphonia), although such consequences are rare (Kissel and Youmans, 1992).
The posterior surface of a typical cervical vertebral body is slightly indented (Panjabi et al., 2001a) and possesses two or more foramina for exit of the basivertebral veins (Williams et al., 1995). The posterior longitudinal ligament attaches to the superior and inferior margins of the posterior aspect of the cervical vertebral bodies.
The superior and inferior surfaces of the vertebral bodies typically are described as being sellar or saddle shaped. More specifically, the superior surface is concave from left to right as a result of the raised lateral lips (uncinate processes). The superior surface is also convex from front to back because of the beveling of its anterior aspect. The inferior surface is convex from left to right and concave from anterior to posterior. Much of the concavity is created by the anterior lip of the inferior surface. This concavity is greatest at C3 and C4 and then becomes shallower as one moves inferiorly from C5 to C7 (Panjabi et al., 2001a). The inferior aspects of the vertebral bodies of C5 and C6 have the greatest anteroposterior dimension (Lu et al., 1999). Osteophyte (bone spur) formation is also most common at the C5-6 levels (Wiegand et al., 2003).
Raised Lips at the Superior Aspect of the Vertebral Bodies and the Uncovertebral Joints.
When viewed from the lateral or anterior aspect, several unique characteristics of the vertebral bodies become apparent (see Fig. 5-1, B). Lateral lips (uncinate processes) project from the superior surface of each typical cervical vertebra. These structures arise as elevations of the lateral and posterior rims on the top surface of the vertebral bodies (Dupuis et al., 1985). The posterior components of the uncinate processes tend to become more prominent in the lower cervical vertebrae. Normally the uncinate processes allow for flexion and extension of the cervical spine and help to limit lateral flexion. In addition, the uncinate processes serve as barriers to posterior and lateral IVD protrusion. The relationship of the left and right uncinate processes to the IVD has led at least one investigator to state that IVD herniation in the cervical region may occur less frequently than previously thought (Bland, 1989).
The uncinate processes of one vertebra may articulate with the small indentations found on the inferior surface of the vertebra above by means of small synovial joints. These joints are sometimes called the uncovertebral joints (of von Luschka). They consist of oblique clefts that develop at approximately 9 to 10 years of age. The clefts are limited medially by the IVD and laterally by capsular ligaments (Williams et al., 1995); the latter are derived from the anulus fibrosus of the IVD. Some investigators do not believe that the uncovertebral joints can be classified as synovial joints (Tondury, 1943; Orofino, Sherman, and Schecter, 1960; Bland, 1989), whereas others believe they do possess a synovial lining (Cave, Griffiths, and Whiteley, 1955). Regardless of their true classification, the distance between the apposing bony structures in the uncovertebral region is only approximately one third of that of the remainder of the interbody space (Hadley, 1957). In addition, the uncovertebral joints frequently undergo degeneration with resulting bony outgrowth (osteophyte formation) of the uncinate processes. These osteophytes may encroach on neighboring structures such as the vertebral artery and exiting cervical spinal nerves within the intervertebral foramen (Hadley, 1957; Bland, 1989). The uncinate processes are significantly higher at C4 to C6 (5.8 ± 1.1 mm to 6.1 ± 1.3 mm) than at C3 or C7 levels, and the length of the exiting dorsal and ventral roots and spinal nerve from the dura mater to the lateral border of the intervertebral foramina increases from C3 to C7. The higher uncinate processes at C4-6 and the longer nerves in this region may help to explain the higher incidence of neural compression syndromes associated with the C4-6 vertebral levels (Ebraheim et al., 1997c). Furthermore, dense fibrous tissue attaches to the uncinate process and this tissue then encases the spinal nerve and vertebral artery in the intertransverse space at the same level. The vertebral artery may become vulnerable to irritation, or in extreme cases perforation, from osteophytes projecting from the uncinate processes, because in essence the vertebral artery is held in a relatively close relationship to the uncinate process by this uncinate–spinal nerve–vertebral artery fibrous complex (Ebraheim et al., 1998; Yilmazlar et al., 2003a,b). Because the vertebral artery courses closer to the uncinate processes in the midcervical region than in the lower cervical region, the vertebral artery in the midcervical region is potentially more vulnerable than the vertebral artery in the lower cervical region to damage from osteophytes arising from the uncinate processes (Ebraheim et al., 1997c).
Injury to the Vertebral Bodies.
Injury to the vertebral bodies frequently, but not always, results in swelling of the prevertebral soft tissues (Miles and Finlay, 1988). Therefore swelling of the prevertebral tissues seen on standard x-ray films after trauma is an indication for further diagnostic studies such as computed tomography (CT).
Pedicles.
The left and right pedicles of a typical cervical vertebra (see Fig. 5-1) are small and project posterolaterally from the vertebral bodies at approximately a 60-degree angle to the frontal (coronal) plane. They form the medial boundary of the left and right foramina of the transverse processes, respectively. The length of the pedicles is similar in the C3 to C7 vertebrae, and the pedicles of C2 are shorter than those of the rest of the cervical region (Karaikovic et al., 1997). The pedicles of typical cervical vertebrae are placed more or less midway between the superior and inferior margins of the vertebral body. Therefore the superior and inferior vertebral notches are of roughly equal size (Williams et al., 1995). The spinal nerve, surrounded by a sleeve of dura, courses just above (superior to) the pedicle of each typical cervical vertebra.
The cervical pedicles have a relatively thick layer of cortical bone (also known as compact bone) that surrounds a central core of cancellous bone (also known as trabecular, or spongy, bone). Generally, the cortex of the lateral aspect of the pedicle (the region closest to the vertebral artery) is thinner than that of the medial side. However, approximately 4% of pedicles are composed entirely of cortical bone with no medullary cavity. In the remainder of pedicles, the central trabecular bone is continuous with that of the articular processes. This allows transfer of loads from the vertebral body to the articular pillar (discussed later in this chapter) during flexion and from the articular pillar to the vertebral body during extension (Pal et al., 1988).
Transverse Processes.
The left and right transverse processes (TPs) of a typical cervical vertebra are each composed of two roots, or bars, one anterior and one posterior (see Fig. 5-1). The two roots end laterally as tubercles (anterior and posterior). Occasionally an anterior tubercle of one vertebra is significantly longer than those of its neighbors. This usually occurs at C5 or C6 and has been associated with a lack of segmentation (congenital fusion) of the vertebral body of the segment with the long anterior tubercle to the vertebral body directly above or below (Ehara, 1996). The two tubercles are joined to one another by an intertubercular lamella, which is less correctly known as a costotransverse lamella (bar) (Williams et al., 1995). The distance between the lateral tips of the left and right TPs is greatest at C1. This same distance, although smaller, remains relatively constant from C2 through C6 and then increases greatly at C7.
In the typical cervical vertebrae a gutter, or groove, for the spinal nerve is formed between the anterior and posterior roots of each TP (Fig. 5-2). This groove serves as a passage for exit of the spinal nerve and its largest branch, the anterior primary division (ventral ramus). The neural grooves form approximately a 50-degree anterior angle with the midsagittal plane, except for C7 where the angle is larger, usually 56 to 57 degrees. Generally, there is no significant difference between the length of the neural grooves in males and females. The depth of the neural grooves gradually increases from C3 (≈3 mm ± 1.0 mm deep) to C7 (≈5 mm ± 0.7 mm deep) (Ebraheim, Biyani, and Salpietro, 1996). The intertubercular lamellae of C3 and C4 have an oblique course, descending from the anterior root and passing laterally as they reach the posterior root of the TP. Therefore the anterior tubercles (roots) of these vertebrae are shorter than the posterior ones, and the grooves for the spinal nerves are deeper posteriorly than anteriorly. The left and right intertubercular lamellae of C6 are wide (from left to right) and shallow (from superior to inferior) (Williams et al., 1995).
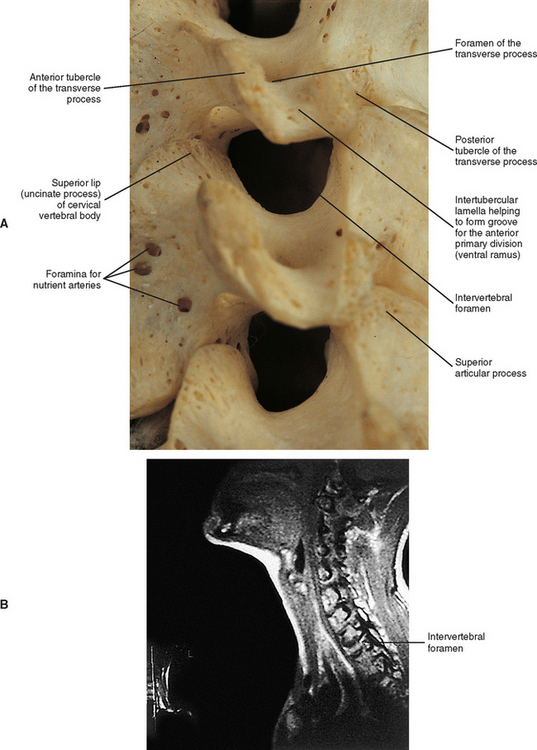
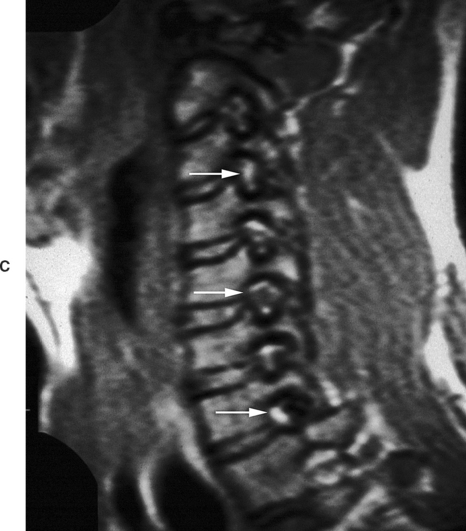
(From Cramer G et al. [2002]. Oblique MRI of the cervical intervertebral foramina: A comparison of three techniques. J Neuromusculoskel Syst 10, 41-51.) (B, Courtesy Dr. Dennis Skogsbergh.)
A dural root sleeve, which surrounds each spinal nerve, as well as its continuation as the epineurium of the ventral ramus (anterior primary division) are held to the gutter of the TP by fibrous tissue. This strong attachment to the TP is unique to the cervical region. The C4, C5, and C6 spinal nerves and anterior primary divisions (ventral rami) are held in place to the groove for the spinal nerve by means of tough connective tissue attachments. The nerves at the other levels are not as tightly bound to the bone of the groove for the spinal nerve. Lateral traction of the dura (more specifically the “dural funnel”) pulls it laterally into an intervertebral foramen (IVF) until it plugs the foramen. This prevents further traction on the nerve roots and rootlets and their attachment to the spinal cord. In addition, traction of nerves also tractions the denticulate ligament, which in turn causes the spinal cord to move laterally toward the traction force, thus removing tension on the spinal cord from the nerve roots undergoing traction (Sunderland, 1974).
The dural root sleeves in the cervical region receive more sensory innervation than any other region of cervical dura, and the fibers innervating the dural root sleeves originate directly from the dorsal root ganglia, not the recurrent meningeal nerves (Yamada et al., 1998). This means significant traction or compression of the cervical dural root sleeves could result in the production of pain.
A dorsal ramus leaves each spinal nerve shortly after the spinal nerve is formed by the union of the dorsal and ventral roots. The dorsal ramus (posterior primary division) courses posteriorly and laterally along the zygapophysial joint (see next section), supplying the joint with sensory innervation. The ramus then passes posteriorly to supply the cervical parts of the deep back muscles with motor, nociceptive, and proprioceptive innervation; and then continues posteriorly to reach the dermal and epidermal layers of the back to supply them with sensory innervation. The nerves of the cervical region are discussed in more detail later in this chapter.
As the name implies, the foramen of the TP is an opening within the TP. This foramen is present in the left and right TPs of all cervical vertebrae. It was previously called the foramen transversarium, but the currently preferred term is simply foramen of the transverse process. The boundaries of this foramen are formed by four structures: the pedicle, anterior root of the TP, posterior root of the TP, and intertubercular lamella. The sizes of left and right foramina of the TPs of a single vertebra frequently are asymmetric, and occasionally the foramen of a single TP is double (Taitz, Nathan, and Arensburg, 1978). The vertebral artery normally enters the foramen of the TP of C6 and continues superiorly through the corresponding foramina of C5 through C1. The vertebral artery of each side loops posteriorly and then medially around the superior articular process of the atlas on the corresponding side. The artery then continues superiorly to pass through the foramen magnum. The ventral rami of the C3 to C7 spinal nerves pass posterior to the vertebral artery as they exit the gutter (groove) for the spinal nerve of the TP (see Fig. 5-2).
Several vertebral veins on each side also pass through the foramina of the TPs. These veins begin in the atlanto-occipital region and continue inferiorly through the foramina of the TPs of C1 through C7 and then enter the subclavian vein. The vertebral veins receive branches from both the epidural venous plexus and the external vertebral venous plexus. In addition to the veins, a plexus of sympathetic nerves also accompanies the vertebral artery as it passes through the foramina of the TPs of C1 through C6 (see Fig. 5-22). The vertebral artery and sympathetic plexus associated with it are discussed in more detail later in this chapter.
As mentioned in Chapter 2, the vertebrae of each region of the spine possess specific sites that are capable of developing ribs. Such regions are known as costal elements, costal processes, or pleurapophyses. The cervical region is no exception. The costal process of a typical cervical vertebra makes up the majority of its TP. In fact, all but the most medial aspect of the posterior root of the TP participates in the formation of the costal process. The costal processes may develop into cervical ribs in some individuals (Williams et al., 1995). This occurs most frequently at the level of C7. A cervical rib at C7 may be present and may compress underlying portions of the brachial plexus and the subclavian artery. The symptom complex that results from compression of these structures is commonly known as the thoracic outlet syndrome, and a cervical rib is one cause of this syndrome (Bland, 1987). (Thoracic outlet syndrome is discussed in detail in Chapter 6). A cervical rib may develop as a small projection of the TP or may be a complete rib that attaches to the manubrium of the sternum or the first thoracic rib. However, the cervical rib usually is incomplete, and a bridge of fibrous tissue usually connects the tip of the cervical rib to either the manubrium or first thoracic rib. The osseous extension of the cervical TP frequently can be detected on standard x-ray films, but the fibrous band is much more difficult to evaluate radiographically.
Articular Processes and Zygapophysial Joints.
The general characteristics of the articular processes and the zygapophysial joints (Z joints) are discussed in Chapter 2. The unique characteristics of the cervical Z joints are discussed here. The superior articular processes and their hyaline cartilage-lined facets face posteriorly, superiorly, and slightly medially (see Fig. 5-1), and the cervical Z joints lie approximately 45 degrees to the horizontal plane (White and Panjabi, 1990; Panjabi et al., 1991). More specifically, the facet joints of the upper cervical spine lie at approximately a 35-degree angle to the horizontal plane, and the lower cervical Z joints form a 65-degree angle to the horizontal plane (Oliver and Middleditch, 1991). The articular processes and their hyaline cartilage–lined facets of the typical cervical vertebrae are more horizontally oriented at birth (averaging approximately 34 degrees to the horizontal plane) than in the adult. The articular processes then gradually become more vertically oriented until approximately 10 years of age, reaching an angle averaging approximately 45 to 53 degrees. They maintain this angle throughout the remainder of life. The seventh cervical vertebra follows the same pattern, but throughout life is more vertically oriented than the typical vertebrae. The more horizontally oriented articular processes of children (when compared with adults) lead to an increased anterior-to-posterior translation among the typical cervical vertebrae (Kasai et al., 1996).
Right-to-left asymmetry of the plane of articulation of the cervical articular processes is common. This is particularly true at the junction of the cervical and thoracic regions. An asymmetry of greater than 10 degrees in the coronal (frontal) plane occurs 24% of the time at C6, 10% of the time at C7, and 16% of the time at T1. There is no significant difference in asymmetry of the spatial orientation of the articular processes between men and women (Boyle, Singer, and Milne, 1996).
The heights of the superior articular facets of C3-7 range from 10.0 to 8.7 mm, decreasing in height from C3 to C7. The widths are approximately 11.0 mm from C3 to C6 and increase to 12.3 mm at C7. The height and width of the superior articular processes of males are again greater than that of females (Ebraheim et al., 1997b).
The heights (superior-to-inferior dimension) of the inferior articular facets range from 9.0 to 9.6 mm from C3 to C7; C3 has the greatest height and C5 the smallest. There is a steady decrease in height from C3 to C5, then a slight increase at C6, and again at C7. Therefore unlike the superior articular processes, there is no steady trend in height of the inferior articular processes. The width of the inferior articular processes ranges from 11.2 to 12.1 mm, and there is a small, steady increase from C3 to C7. The height and width of the inferior articular processes of males are greater than that of females (Ebraheim et al., 1997b).
The appearance of the cervical Z joints changes significantly with age. Before age 20 the articular cartilage is smooth, approximately 1.0 to 1.3 mm thick, and the subarticular bone is regular in thickness. The articular cartilage thins with age, and most adult cervical Z joints possess an extremely thin layer of cartilage with irregularly thickened subarticular cortical bone. These changes of articular cartilage and the subchondral bone usually go undetected on CT and magnetic resonance imaging (MRI) scans. Osteophytes (bony spurs) projecting from the articular processes and sclerosis (thickening) of the bone within the articular processes occur often in adult cervical Z joints (Fletcher et al., 1990). Osteophytes of the superior articular processes can extend into the intervertebral foramen and compress the neural elements coursing through this region (Hackney, 1992).
The Z joint capsules probably do little to limit motion in the cervical region (Onan, Heggeness, and Hipp, 1998). They are thicker along the lateral aspect of the joint compared with the posterior aspect, and become even thicker from posterior to anterior along the lateral aspect of the capsule, making the anterolateral aspect the thickest region of the Z joint capsule (Tonetti et al., 1999). However, overall the Z joint articular capsules of the cervical region are thin (Panjabi et al., 1991) and are longer and looser than those of the thoracic and lumbar regions. The collagen fibers, which make up the capsules, course from the region immediately surrounding the articular facet of the inferior articular process of the vertebra above to the corresponding region of the superior articular process of the vertebra below (Fig. 5-3; see also Figs. 5-11 and Figs. 5-16). The bands of collagen fibers are approximately 9 mm long and course perpendicular to the plane created by the Z joint (Panjabi et al., 1991).
Z joint synovial folds (menisci) project into the Z joints at all levels of the cervical spine. There are four distinct types of cervical Z joint menisci, which are also known as synovial folds (Yu, Sether, and Haughton, 1987) (Fig. 5-4). Type I menisci are thin and protrude far into the Z joints, covering approximately 50% of the joint surface. They are found only in children. Type II menisci are relatively large wedges that protrude a significant distance into the joint space and are found almost exclusively at the lateral C1-2 Z joints. Type III folds are small nubs found throughout the C2-3 to C6-7 cervical Z joints of most healthy adults. Type IV menisci are large and thick and usually are only found in degenerative Z joints. Types II and IV have been seen on MRI scans (Yu, Sether, and Haughton, 1987).
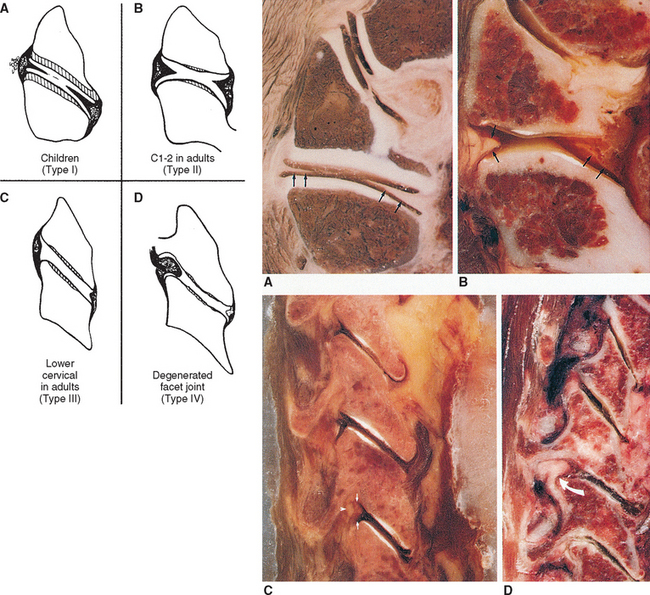
(From Yu S et al. [1987]. Facet joint menisci of the cervical spine: Correlative MR imaging and cryomicrotomy study. Radiology, 164, 79-82.)
When the individual vertebrae are united, the articular processes of each side of the cervical spine form an articular pillar that bulges laterally at the pediculolaminar junction (Williams et al., 1995). This pillar is conspicuous on lateral x-ray films. The cervical articular pillars (left and right) help to support the weight of the head and neck (Pal et al., 1988). Therefore weight bearing in the cervical region is carried out by a series of three longitudinal columns: one anterior column, which runs through the vertebral bodies, and two posterior columns, which run through the right and left articular pillars (Louis, 1985; Pal et al., 1988).
Articular pillar fracture is fairly common in the cervical spine and frequently goes undetected (Renaudin and Snyder, 1978). This type of fracture is usually a chip fracture of a superior articular facet and process. The patient often experiences transient radicular pain (see Chapter 11), which usually is followed by mild to intense neck pain. Persistent radiculopathy in such patients indicates displacement of the fractured facet and articular process onto the dorsal root within the intervertebral foramen (Czervionke et al., 1988) (see Fig. 5-2).
The posterior primary division (dorsal ramus) of the spinal nerve provides sensory innervation by means of both mechanoreceptors and free nerve endings to the Z joint capsule. This indicates that the Z joint capsules are important for providing information on both joint position and tissue damage to the central nervous system (McLain, 1994). The Z joints are a source of pain in a substantial number of patients with neck pain (Aprill and Bogduk, 1992). Pain arising from pathologic conditions or dysfunction of the cervical Z joints can refer to regions distant from the affected joint (Bogduk, 1989b; Aprill, Dwyer, and Bogduk, 1990; Dwyer, Aprill, and Bogduk, 1990). The two most common types of pain referral are neck pain and head pain (headache) arising from the C2-3 Z joints, and neck pain and shoulder pain arising from the C5-6 Z joints (Bogduk and Marsland, 1988).
Laminae.
The laminae of the cervical region are fairly narrow from superior to inferior. Therefore a gap can be seen between the laminae of adjacent vertebrae in a dried specimen (see Fig. 5-3, C). However, this gap is filled by the ligamentum flavum in the living (see Fig. 7-20). The upper border of each cervical lamina is thin, and the anterior surface of the inferior border is roughened by the attachment of the ligamentum flavum. The ligamentum flavum is discussed in detail later in this chapter.
Vertebral Canal.
A vertebral foramen of a typical cervical vertebra is triangular (trefoil) in shape (see Fig. 5-1). It is also large, allowing it to accommodate the cervical enlargement of the spinal cord.
Recall that the collection of all the vertebral foramina is known as the vertebral (spinal) canal. Therefore the IVDs and ligamenta flava also participate in the formation of the vertebral canal. The internal vertebral venous plexus lies within the epidural adipose tissue of the vertebral canal. The left and right longitudinal channels of the cervical anterior internal vertebral venous plexus are kept in the most anterior and lateral regions of the epidural space by a thin fibrous membrane on each side of the vertebral canal. These venous channels anastomose with one another behind each vertebral body via retrocorporeal veins that course between the posterior longitudinal ligament and vertebral bodies (Chaynes et al., 1998).
The vertebral canal is fairly large in the upper cervical region but narrows from C3 to C6. More specifically, the transverse (left-to-right) dimension remains relatively constant at approximately 24.6 mm. However, the sagittal dimension becomes smaller; therefore the shape of the trefoil becomes relatively wider as one descends the vertebral canal in the cervical region (Hackney, 1992). In fact, the spinal cord takes up 75% of the space available within the vertebral canal at the C6 level. The volume of cerebrospinal fluid in the subarachnoid space in the cervical vertebral canal is least in extension and greatest in flexion, and the total change in volume of the cervical subarachnoid space (C2 to C7 levels) during full extension to full flexion is approximately 1.9 ml (Holmes et al., 1996). Table 5-1 summarizes the general characteristics of the cervical vertebral canal.
Table 5-1 General Characteristics of the Cervical Vertebral Canal
Region | Dimensions |
---|---|
Upper cervical vertebral canal | Upper canal is infundibular in shape, wider superiorly than inferiorly;less than half the available space is occupied by the spinal cord at C1 |
C4 | Narrowing of the vertebral canal begins |
C6 | Cord occupies 75% of the vertebral canal |
C1-7 | Critical anteroposterior dimension is 12–13 mm |
Connective tissue attachments to the posterior spinal dura mater.
Connective tissue attachments to the posterior aspect of the spinal dura arising from the foramen magnum, posterior arch of C1, spinous process of C2 (Von Lanz, 1929), rectus capitis posterior minor muscle (Hack et al., 1995a,b; Zumpano, Jagos, and Hartwell-Ford, 2002), ligamentum nuchae (Mitchell, Humphreys, and O’sullivan, 1998; Humphreys et al., 2003), and ligamenta flava between C1-2 and C6-7 (Shinomiya et al., 1995, 1996) have been described. These attachments may hold the dura mater posteriorly during cervical extension (to prevent buckling of the dura mater into the spinal cord) and flexion (to prevent the dura from moving forward and compressing the cord). Some authors have speculated that increased tension of the cervical paraspinal muscles may traction the connection between the rectus capitis posterior minor muscle and the dura, leading to headaches secondary to dural tension (Alix and Bates, 1999). Others have proposed that tearing of these connective tissue attachments during the flexion component of flexion-extension (whiplash) type of injuries or other trauma to the cervical region could lead to buckling of the dura mater into the cervical segments of the spinal cord. Such dural buckling could potentially result in chronic neck pain, headaches, disorders of balance, and signs and symptoms of cervical myelopathy experienced by some patients who have had trauma to the cervical region (Mitchell, Humphreys, and O’sullivan, 1998). However, these theories remain conjecture until further basic science and clinical studies are performed to investigate the relationships between connective tissue attachments of the spinal dura mater and clinical conditions. In this regard, Shinomiya et al. (1995, 1996) have identified connective tissue attachments between the ligamenta flava at C1-2 and C6-7, which they call epidural ligaments. Using MRI scans of humans and spinal cord blood flow measurements and electrophysiologic recordings of the nerves innervating upper extremity muscles and from the spinal cord in an animal model (cat), they have identified a relationship between tearing or absence of the epidural ligaments at C6-7 and Hirayama-type amyotrophy. This condition is characterized by isolated muscle paralysis and atrophy of hand muscles resulting from segmental degenerative changes of the spinal cord gray matter. The authors found that absence or tearing of the epidural ligaments caused the spinal dura to press against the posterior aspect of the spinal cord during flexion of the spine. This compressed the spinal cord and its arterial supply, and led to a reduced blood flow to the anterior aspect of the spinal cord. The decreased blood flow to the spinal cord subsequently decreased motor activity of peripheral nerves to the upper extremity, and also decreased neuronal activity in the spinal cord at the C7 and C8 spinal cord segments. Their work provides strong evidence in support of an important physiologic role of connective tissue attachments to the posterior aspect of the spinal dura mater.
Cervical myelopathy.
Pathology of the spinal cord is termed cervical myelopathy. The development of bone spurs (osteophytes) from the posterior aspect of the vertebral bodies, and articular processes close to the Z joints and uncinate processes have long been accepted as potential causes of compression of the spinal cord and spinal nerve roots, respectively (Bailey and Casamajor, 1911; Boulos and Lovely, 1996). Other potential causes of spinal cord and nerve root compression include IVD protrusion, spinal cord tumor, Z joint hypertrophy, ossification of the posterior longitudinal ligament, buckling of a ligamentum flavum in a congenitally narrow vertebral canal, and a displaced fracture of a lamina, pedicle, or vertebral body. The critical anteroposterior dimension of the cervical vertebral canal is approximately 12 to 13 mm before symptoms occur. A vertebral canal this narrow usually is the result of one of the mentioned pathologic conditions combined with a congenitally narrow canal. However, the minimum dimension before symptoms occur may be slightly lower in blacks than whites. The transverse and midsagittal diameter of the cervical vertebral canal is significantly smaller in black than white South Africans, although determining if the difference in dimensions results from heredity, environment, or prenatal and childhood nutrition is difficult. However, white South Africans have more bone spurs (spondylosis or osteophyte formation) affecting the size of the vertebral canal than black South Africans (Taitz, 1996).
When increased bone formation (spondylosis) of the vertebral bodies, articular processes, or the uncinate processes contributes to cervical myelopathy, the term cervical spondylotic myelopathy (CSM) is appropriate. CSM develops slowly, is progressive, and also may be related to a congenitally narrow vertebral canal (Crawford, Cassidy, and Burns, 1995; Hukuda et al., 1996). Less common causes of the condition are ossification of the posterior longitudinal ligament, ossification of the ligamentum flavum, and calcium pyrophosphate dehydrate deposition disease (Omura et al., 1996). In addition, Hukuda et al. (1996) found that large vertebral bodies (an increase of both the transverse and sagittal diameters) were significantly related to CSM. They assumed this was because the large vertebral bodies resulted in larger osteophytes and larger disc bulges; therefore increasing the chances of clinically significant narrowing of the vertebral canal that would result in compression of the spinal cord. Cervical myelopathy in elderly persons frequently results from anterior or posterior translation of adjacent vertebrae (anterolisthesis or retrolisthesis), secondary to narrowing of the IVDs or injury to the cervical region (Kawaguchi et al., 2003). Inflammation, initiated by arachnoid cells, may occur in the arachnoid mater in certain cases of CSM (Frank, 1995).
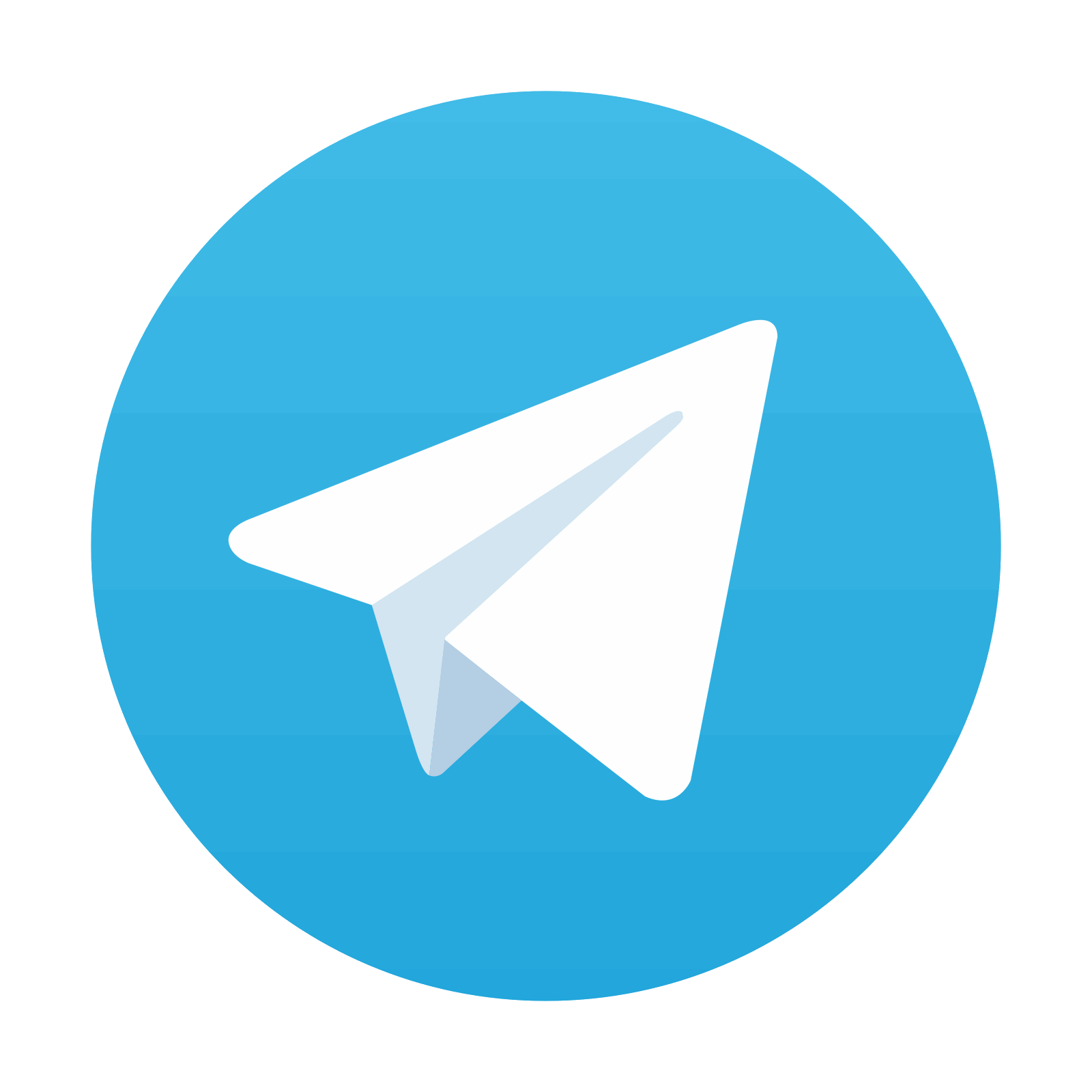
Stay updated, free articles. Join our Telegram channel

Full access? Get Clinical Tree
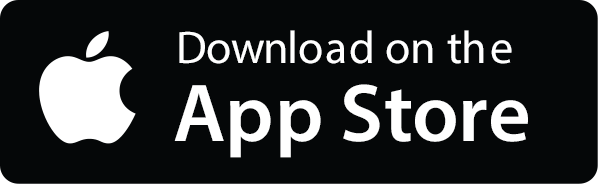
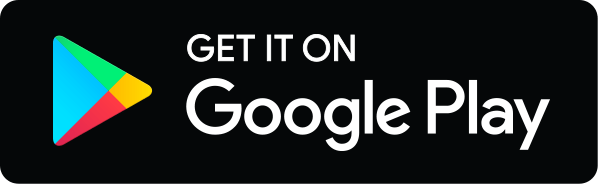
