Chapter 3
The cells, tissues and organisation of the body
Animations
3.1 Cellular functions compared to organ systems 33
3.4 Passive transport 36
3.5 Active transport 37
3.6 Mucous membrane 45
3.7 Serous membrane 45
3.8 Synovial membrane 45
3.9 Vertebral column 48
Cells are the body’s smallest functional units. They are grouped together to form tissues, each of which has a specialised function, e.g. blood, muscle, bone. Different tissues are grouped together to form organs, e.g. the heart, stomach and brain. Organs are grouped together to form systems, each of which performs a particular function that maintains homeostasis and contributes to the health of the individual (see Fig. 1.2, p. 5). For example, the digestive system is responsible for taking in, digesting and absorbing food, which involves a number of organs, including the stomach and intestines. The structure and functions of cells and types of tissue are explored in this chapter.
The terminology used to describe the anatomical relationships between body parts, the skeleton and the cavities within the body are then considered.
The final section considers features of benign and malignant tumours, their causes and how they grow and may spread.
The cell: structure and functions
The human body develops from a single cell called the zygote, which results from the fusion of the ovum (female egg cell) and the spermatozoon (male sex cell). Cell division follows and, as the fetus grows, cells with different structural and functional specialisations develop, all with the same genetic make-up as the zygote. Individual cells are too small to be seen with the naked eye. However, they can be seen when thin slices of tissue are stained in the laboratory and magnified using a microscope.
A cell consists of a plasma membrane enclosing a number of organelles suspended in a watery fluid called cytosol (Fig. 3.1). Organelles, literally ‘small organs’, have individual and highly specialised functions, and are often enclosed in their own membrane within the cytosol. They include: the nucleus, mitochondria, ribosomes, endoplasmic reticulum, Golgi apparatus, lysosomes and the cytoskeleton. The cell contents, excluding the nucleus, is the cytoplasm, i.e. the cytosol and other organelles.
Plasma membrane
The plasma membrane (Fig. 3.2) consists of two layers of phospholipids (see p. 27) with proteins and sugars embedded in them. In addition to phospholipids, the lipid cholesterol is also present. The phospholipid molecules have a head, which is electrically charged and hydrophilic (meaning ‘water loving’), and a tail which has no charge and is hydrophobic (meaning ‘water hating’, Fig. 3.2A). The phospholipid bilayer is arranged like a sandwich with the hydrophilic heads aligned on the outer surfaces of the membrane and the hydrophobic tails forming a central water-repelling layer. These differences influence the transfer of substances across the membrane.
Figure 3.2 The plasma membrane. A. Diagram showing structure. B. Coloured atomic force micrograph of the surface showing plasma proteins.
Membrane proteins
Those proteins that extend all the way through the membrane provide channels that allow the passage of, for example, electrolytes and non-lipid soluble substances. Protein molecules on the surface of the plasma membrane are shown in Figure 3.2B. The membrane proteins perform several functions:
• they can act as receptors (specific recognition sites) for hormones and other chemical messengers
• some are involved in pumps that transport substances across the membrane.
Organelles
3.1
Nucleus
All body cells have a nucleus, with the exception of mature erythrocytes (red blood cells). Skeletal muscle fibres and some other cells contain several nuclei. The nucleus is the largest organelle and is contained within the nuclear envelope, a membrane similar to the plasma membrane but with tiny pores through which some substances can pass between it and the cytoplasm.
The nucleus contains the body’s genetic material, in the form of deoxyribonucleic acid (DNA, p. 438); this directs all its metabolic activities. In a non-dividing cell DNA is present as a fine network of threads called chromatin, but when the cell prepares to divide the chromatin forms distinct structures called chromosomes (Fig. 17.1, p. 439). A related substance, ribonucleic acid (RNA) is also found in the nucleus. There are different types of RNA, not all found in the nucleus, but which are in general involved in protein synthesis.
Within the nucleus is a roughly spherical structure called the nucleolus, which is involved in synthesis (manufacture) and assembly of the components of ribosomes.
Mitochondria
Mitochondria are membranous, sausage-shaped structures in the cytoplasm, sometimes described as the ‘power house’ of the cell (Fig. 3.3). They are central to aerobic respiration, the processes by which chemical energy is made available in the cell. This is in the form of ATP, which releases energy when the cell breaks it down (see Fig. 2.10, p. 28). Synthesis of ATP is most efficient in the final stages of aerobic respiration, a process which requires oxygen (p. 315). The most active cell types have the greatest number of mitochondria, e.g. liver, muscle and spermatozoa.
Ribosomes
These are tiny granules composed of RNA and protein. They synthesise proteins from amino acids, using RNA as the template (see Fig. 17.5, p. 441). When present in free units or in small clusters in the cytoplasm, the ribosomes make proteins for use within the cell. These include the enzymes required for metabolism. Metabolic pathways consist of a series of steps, each driven by a specific enzyme. Ribosomes are also found on the outer surface of the nuclear envelope and rough endoplasmic reticulum (see Fig. 3.3 and below) where they manufacture proteins for export from the cell.
Endoplasmic reticulum (ER)
Endoplasmic reticulum is an extensive series of interconnecting membranous canals in the cytoplasm (Fig. 3.3). There are two types: smooth and rough. Smooth ER synthesises lipids and steroid hormones, and is also associated with the detoxification of some drugs. Some of the lipids are used to replace and repair the plasma membrane and membranes of organelles. Rough ER is studded with ribosomes. These are the site of synthesis of proteins, some of which are ‘exported’ from cells, i.e. enzymes and hormones that leave the parent cell by exocytosis (p. 37) to be used by cells elsewhere.
Golgi apparatus
The Golgi apparatus consists of stacks of closely folded flattened membranous sacs (Fig. 3.4). It is present in all cells but is larger in those that synthesise and export proteins. The proteins move from the endoplasmic reticulum to the Golgi apparatus where they are ‘packaged’ into membrane-bound vesicles. The vesicles are stored and, when needed, they move to the plasma membrane and fuse with it. The contents are expelled (secreted) from the cell. This process is called exocytosis (p. 37).
Lysosomes
Lysosomes are small membranous vesicles pinched off from the Golgi apparatus. They contain a variety of enzymes involved in breaking down fragments of organelles and large molecules (e.g. RNA, DNA, carbohydrates, proteins) inside the cell into smaller particles that are either recycled, or extruded from the cell as waste material.
Lysosomes in white blood cells contain enzymes that digest foreign material such as microbes.
Cytoskeleton
This consists of an extensive network of tiny protein fibres (Fig. 3.5).
Figure 3.5 Fibroblasts. Fluorescent light micrograph showing their nuclei (purple) and cytoskeletons (yellow and blue).
Microfilaments.
These are the smallest fibres. They provide structural support, maintain the characteristic shape of the cell and permit contraction, e.g. actin in muscle cells (p. 421).
Centrosome.
This directs organisation of microtubules within the cell. It consists of a pair of centrioles (small clusters of microtubules) and plays an important role in cell division.
Cell extensions.
These project from the plasma membrane in some types of cell and their main components are microtubules, which allow movement. They include:
• microvilli – tiny projections that contain microfilaments. They cover the exposed surface of certain types of cell, e.g. absorptive cells that line the small intestine (see Fig. 3.6). By greatly increasing the surface area, microvilli make the structure of these cells ideal for their function – maximising absorption of nutrients from the small intestine.
• cilia – microscopic hair-like projections containing microtubules that lie along the free borders of some cells (see Fig. 10.12, p. 249). They beat in unison, moving substances along the surface, e.g. mucus upwards in the respiratory tract.
• flagella – single, long whip-like projections, containing microtubules, which form the ‘tails’ of spermatozoa (see Fig. 1.19, p. 15) that propel them through the female reproductive tract.
The cell cycle
Many damaged, dead, and worn out cells can be replaced by growth and division of other similar cells. The frequency with which cell division occurs varies with different types of tissue (p. 44). This is normally carefully regulated to allow effective maintenance and repair of body tissues. At the end of their natural lifespan, ageing cells are programmed to ‘self destruct’ and their components are removed by phagocytosis; a process known as apoptosis (p. 54).
Cells with nuclei have 46 chromosomes and divide by mitosis, a process that results in two new genetically identical daughter cells. The only exception to this is the formation of gametes (sex cells), i.e. ova and spermatozoa, which takes place by meiosis (p. 442).
The period between two cell divisions is known as the cell cycle, which has two phases that can be seen on light microscopy: mitosis (M phase) and interphase (Fig. 3.7).
Interphase
This is the longer phase and three separate stages are recognised:
• synthesis of DNA (S phase) – the chromosomes replicate forming two identical copies of DNA (see p. 442). Therefore, following the S phase, the cell now has 92 chromosomes, i.e. enough DNA for two cells and is nearly ready to divide by mitosis.
• second gap phase – (G2) there is further growth and preparation for cell division.
Mitosis (Figs 3.8 and 3.9)
3.2
This is a continuous process involving four distinct stages visible by light microscopy.
Figure 3.9 Mitosis. Light micrograph showing cells at different stages of reproduction with chromatin/chromatids shown in pink.
Prophase.
During this stage the replicated chromatin becomes tightly coiled and easier to see under the microscope. Each of the original 46 chromosomes (called a chromatid at this stage) is paired with its copy in a double chromosome unit. The two chromatids are joined to each other at the centromere (Fig. 3.8). The mitotic apparatus appears; this consists of two centrioles separated by the mitotic spindle, which is formed from microtubules. The centrioles migrate, one to each end of the cell, and the nuclear envelope disappears.
Metaphase.
The chromatids align on the centre of the spindle, attached by their centromeres.
Anaphase.
The centromeres separate, and one of each pair of sister chromatids (now called chromosomes again) migrates to each end of the spindle as the microtubules that form the mitotic spindle contract.
The mitotic spindle disappears, the chromosomes uncoil and the nuclear envelope reforms.
Following telophase, cytokinesis occurs: the cytosol, intracellular organelles and plasma membrane split forming two identical daughter cells.
Transport of substances across cell membranes
The structure of the plasma membrane provides it with the property of selective permeability, meaning that not all substances can cross it. Those that can, do so in different ways depending on their size and characteristics (see Fig. 1.3, p. 6). 3.3
Passive transport
This occurs when substances can cross the semipermeable plasma and organelle membranes and move down the concentration gradient (downhill) without using energy. 3.4
Facilitated diffusion
This passive process is used by some substances that are unable to diffuse through the semipermeable membrane unaided, e.g. glucose, amino acids. Specialised protein carrier molecules in the membrane have specific sites that attract and bind substances to be transferred, like a lock and key mechanism. The carrier then changes its shape and deposits the substance on the other side of the membrane (Fig. 3.10). The carrier sites are specific and can be used by only one substance. As there are a finite number of carriers, there is a limit to the amount of a substance which can be transported at any time. This is known as the transport maximum.
Osmosis
Osmosis is passive movement of water down its concentration gradient towards equilibrium across a semipermeable membrane and is explained on page 29.
Active transport
3.5
This is the transport of substances up their concentration gradient (uphill), i.e. from a lower to a higher concentration. Chemical energy in the form of ATP (p. 27) drives specialised protein carrier molecules that transport substances across the membrane in either direction (see Fig. 3.10). The carrier sites are specific and can be used by only one substance; therefore the rate at which a substance is transferred depends on the number of sites available.
The sodium–potassium pump
All cells possess this pump, which indirectly supports other transport mechanisms such as glucose uptake, and is essential in maintaining the electrical gradient needed to generate action potentials in nerve and muscle cells.
This active transport mechanism maintains the unequal concentrations of sodium (Na+) and potassium (K+) ions on either side of the plasma membrane. It may use up to 30% of cellular ATP (energy) requirements.
Potassium levels are much higher inside the cell than outside – it is the principal intracellular cation. Sodium levels are much higher outside the cell than inside – it is the principal extracellular cation. These ions tend to diffuse down their concentration gradients, K+ outwards and Na+ into the cell. In order to maintain their concentration gradients, excess Na+ is constantly pumped out across the cell membrane in exchange for K+.
Bulk transport (Fig. 3.11)
Transfer of particles too large to cross cell membranes occurs by pinocytosis (‘cell-drinking’) or phagocytosis (‘cell-eating’). These particles are engulfed by extensions of the cytoplasm (see Fig. 15.1, p. 376) which enclose them, forming a membrane-bound vacuole. Pinocytosis allows the cell to bring in fluid. In phagocytosis larger particles (e.g. cell fragments, foreign materials, microbes) are taken into the cell. Lysosomes then adhere to the vacuole membrane, releasing enzymes which digest the contents.
Extrusion of waste material by the reverse process through the plasma membrane is called exocytosis. Vesicles formed by the Golgi apparatus usually leave the cell in this way, as do any indigestible residues of phagocytosis.
Tissues
Tissues consist of large numbers of the same type of cells and are classified according to the size, shape and functions of their constituent cells. There are four main types of tissue each with subtypes. They are:
Epithelial tissue (Fig. 3.12)
This tissue type covers the body and lines cavities, hollow organs and tubes. It is also found in glands. The structure of epithelium is closely related to its functions, which include:
• protection of underlying structures from, for example, dehydration, chemical and mechanical damage
The cells are very closely packed and the intercellular substance, the matrix, is minimal. The cells usually lie on a basement membrane, which is an inert connective tissue made by the epithelial cells themselves. Epithelial tissue may be:
Simple epithelium
Simple epithelium consists of a single layer of identical cells and is divided into three main types. It is usually found on absorptive or secretory surfaces, where the single layer enhances these processes, and seldom on surfaces subject to stress. The types are named according to the shape of the cells, which differs according to their functions. The more active the tissue, the taller the cells.
Squamous (pavement) epithelium
This is composed of a single layer of flattened cells (Fig. 3.12A). The cells fit closely together like flat stones, forming a thin and very smooth membrane across which diffusion occurs easily. It forms the lining of the following structures:
Cuboidal epithelium
This consists of cube-shaped cells fitting closely together lying on a basement membrane (Fig. 3.12B). It forms the kidney tubules and is found in some glands such as the thyroid (see Fig. 9.9, p. 223). Cuboidal epithelium is actively involved in secretion, absorption and/or excretion.
Columnar epithelium
This is formed by a single layer of cells, rectangular in shape, on a basement membrane (Fig. 3.12C). It lines many organs and often has adaptations that make it well suited to a specific function. The lining of the stomach is formed from simple columnar epithelium without surface structures. The free surface of the columnar epithelium lining the small intestine is covered with microvilli (Fig. 3.6). Microvilli provide a very large surface area for absorption of nutrients from the small intestine. In the trachea, columnar epithelium is ciliated (see Fig. 10.12, p. 249) and also contains goblet cells that secrete mucus (see Fig. 12.5, p. 290). This means that inhaled particles that stick to the mucus layer are moved towards the throat by cilia in the respiratory tract. In the uterine tubes, ova are propelled along by ciliary action towards the uterus.
Stratified epithelia
Stratified epithelia consist of several layers of cells of various shapes. Continual cell division in the lower (basal) layers pushes cells above nearer and nearer to the surface, where they are shed. Basement membranes are usually absent. The main function of stratified epithelium is to protect underlying structures from mechanical wear and tear. There are two main types: stratified squamous and transitional.
Stratified squamous epithelium (Fig. 3.13)
This is composed of several layers of cells. In the deepest layers the cells are mainly columnar and, as they grow towards the surface, they become flattened and are then shed.
Keratinised stratified epithelium.
This is found on dry surfaces subjected to wear and tear, i.e. skin, hair and nails. The surface layer consists of dead epithelial cells that have lost their nuclei and contain the protein keratin. This forms a tough, relatively waterproof protective layer that prevents drying of the live cells underneath. The surface layer of skin is rubbed off and is replaced from below (see Figs 1.16 and 14.4).
Non-keratinised stratified epithelium.
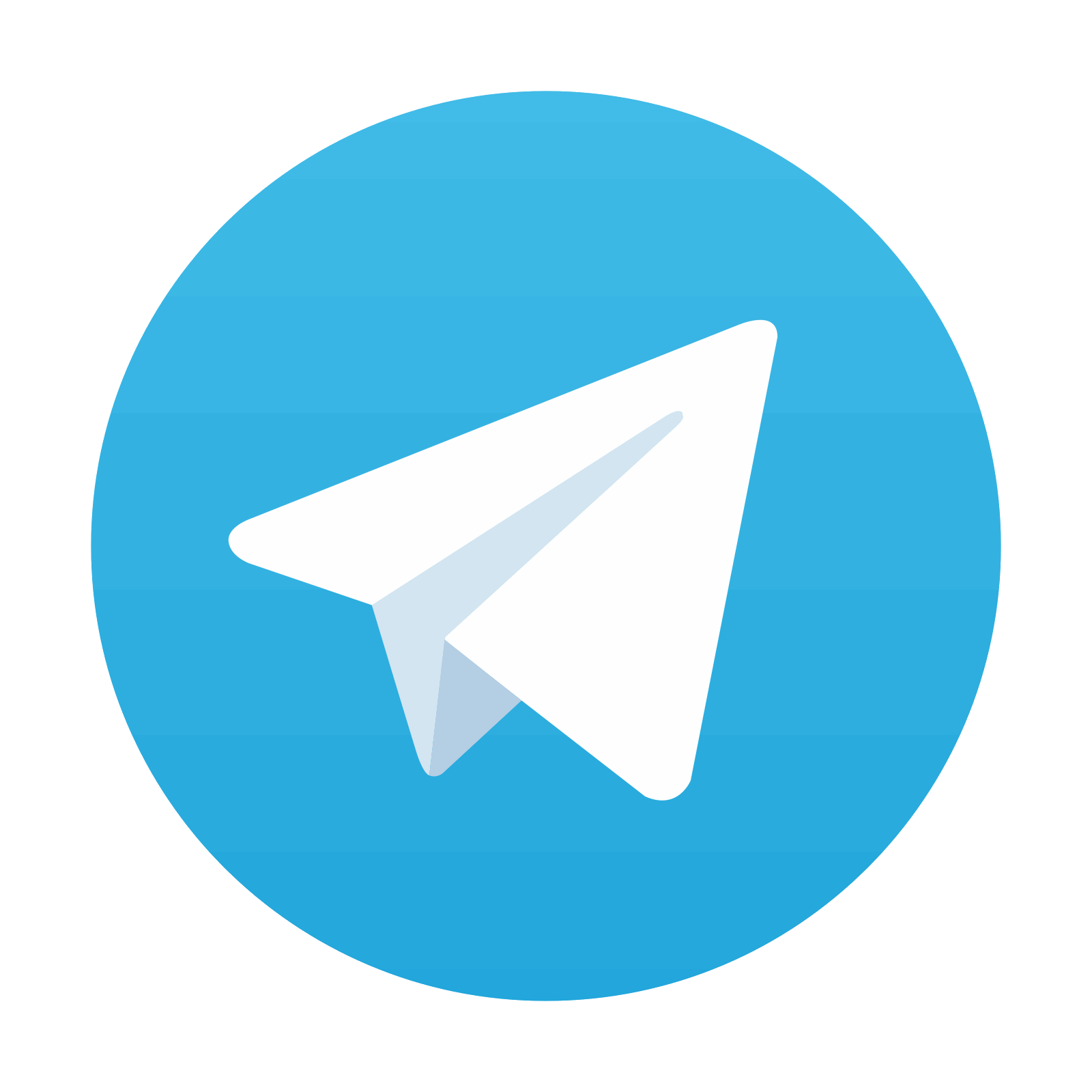
Stay updated, free articles. Join our Telegram channel

Full access? Get Clinical Tree
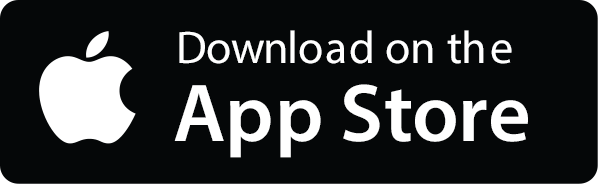
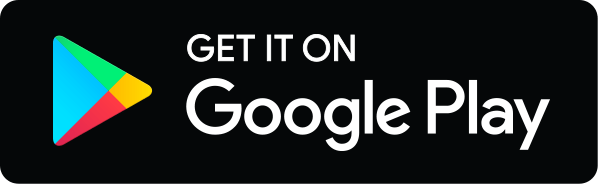
