Chapter Two. The cell—its structures and function
CHAPTER CONTENTS
Physical characteristics of mammalian cells 9
Cell size and shape 10
Cytoskeleton and cell motility 10
Physical characteristics of mammalian cells
Living species are made up of a diversity of cells which are small membrane-bound units filled with a concentrated aqueous solution of carefully balanced chemicals and organelles (Fig. 2.1). Although sharing a common origin, cells show considerable morphological diversity which has evolved to support the functional adaptation and survival of a specific organism. Eukaryotic cells are distinguished from prokaryotic cells by their membrane-bound nucleus and organelles. Cell survival depends on their specific intracellular biochemistry which supports their metabolism and homeostasis. All nucleated mature cells can create copies of themselves by replication and division, which ensures the survival of their genetic lineage. The morphological uniqueness and specialised functions are governed by complex genetic activity. There are more than 200 different cell types in the human body, assembled into tissue types such as epithelia, connective tissue, muscle, conducting neural tissue, non-conducting neuroglia and osteocytes. The function of different cell types is preserved through communication and co-ordination.
![]() |
Figure 2.1 Diagram of the ultrastructure of a cell. (From Hinchliff S M, Montague S E 1990, with kind permission of Elsevier.) |
Mammalian cells average 5–20 micrometres (μm) in diameter (Alberts et al 2008). Their microstructure cannot be determined by light microscopy, but electron microscopes and electronic processing can reveal structural details as small as a few nanometres (nm). This has enabled cell biologists to identify complex cellular ultrastructures that are necessary for microstructural and functional integrity. Cells and their microstructural components are sustained, repaired or replaced when necessary by genetic expression and selective assimilation of matter from the extracellular compartment.
Cell size and shape
Cells differentiate, modify their structure and activities during their stages of development and aggregate correctly to form specific tissues, organs and systems. Genetic and biochemical controls and communication are fundamental to these processes. Knowledge of the size and appearance of different cells allows conclusions about how their morphological and functional features contribute to the whole body. For instance, resting lymphocytes are amongst the smallest of cells, their average diameter being 6 μm, whereas erythrocytes are approximately 7.5–9.0 μm in diameter and columnar epithelial cells are 20 μm tall and 10 μm wide. Some cells are significantly larger than this; for example, bone marrow megakaryocytes average 200 μm in diameter, and mature ova may be over 80 μm in diameter (Bannister 2007). Some neurons and multinucleated skeletal muscle cells are relatively large, reflecting their roles as discussed in later chapters.
The external appearance of a cell depends on its functions, interactions with other cells, external environment and the internal structures which mastermind its activities. Cellular dimensions and metabolic activities are also partly determined by the rate of substrate diffusion across highly selective plasma membranes (Pollard & Earnshaw 2008) which permit rapid diffusion of substrates in both directions over short distances of up to 50 μm. However, as cells increase in size, their mass outstrips their surface area and their shape changes to an irregular or elongated structure as is seen in many neurons. This larger size sustains efficient substrate transport and diffusion, particularly as many physiological processes such as diffusion of gases, ions and nutrient transport depend on cellular surface area.
An increase in cell mass brings problems; for example, the further the cell periphery is from the nucleus, the more difficult is nuclear control of the cytoplasm and plasma membrane. This can be overcome to some extent by increasing surface area by either folding the plasma membrane and forming microvilli, or other surface protrusions, or flattening the entire body of the cell. Alternatively, nuclear control in larger cells can be enhanced by the presence of multiple nuclei which arise due to fusion of mononuclear cells as seen in skeletal or cardiac muscle or, more rarely, by multiplication of the central nucleus without cytoplasmic division.
Cytoskeleton and cell motility
The cytoskeleton is a complex network of specialised proteins organised into filaments and microtubules that extends throughout the cytoplasm. This highly dynamic structure reorganises continuously as the cell changes shape, divides and responds to its environment (Morgan 2007). It is responsible for cellular movement such as cell crawling, the beating of cilia, muscle contraction, migration of phagocytic leucocytes from blood to a site of tissue injury, or in response to the presence of pathogens, and changes in cell shape in the developing embryo. The cytoskeleton also provides the machinery for intracellular movement, such as the transport of organelles within the cytoplasm, as well as the segregation of chromosomes at mitosis.
Motility is also observed in the tip(s) of developing dendrites and axons as they grow in response to local conditions and migrate to their synaptic targets. One of the best examples of cell migration is observed in fibroblasts during early embryonic development, tissue repair and remodelling when the fibroblasts secrete collagen which is essential for the development of the extracellular matrix. As the fibroblasts interact with collagen by means of adhesion plaques they exert traction on the cell matrix. The diverse activities of the cytoskeleton depend on three types of protein filaments: actin filaments, microtubules and the intermediate filaments.
All cells display varying degrees of motility which augments their shape and position and facilitates the best conditions for cellular homeostasis by moving the cytoplasm, specific organelles or vesicles from one part of the cell to another. Whilst the exact molecular mechanisms involved in cell motility are unknown, it is unlikely that a single organelle or cytoskeletal component can be responsible for such complex processes (Pollard & Earnshaw 2008). The following interrelated processes are thought to be essential:
1. Cell motility is dependent on adenosine triphosphate (ATP).
2. Cells define their own motility.
4. Microtubules, intermediate and actin filaments unify the cytoskeleton forming a mechanical structure that resists external forces on the migrating cells.
All activities involve actin filaments in different ways, although the plasma membrane of the crawling cell’s leading edge appears to organise the actin filaments by providing small aggregates of proteins that promote actin polymerisation (Pollard & Earnshaw 2008; see also below). Biochemical and micro-anatomical environments modulate the speed and direction of cell motility.
Epithelial cells
Epithelial cells are derived from the three embryonic germ layers: the ectoderm, the endoderm and the mesoderm (Alberts et al 2008):
• Ectoderm contributes to the development of epithelia and provides the basis for the epidermis, breast glandular tissue, cornea and the junctional zones of the buccal cavity and anal canal.
• Endoderm forms the epithelial lining of the alimentary canal and its glands, most of the respiratory tract and the distal tract of the urogenital tract.
• Mesoderm gives rise to the epithelium-like cells lining internal cavities such as the pericardium, pleural and peritoneal cavities, the lining of the blood vessels and lymph vessels and the proximal parts of the urogenital tract.
In general, epithelial cells form sheets which line the inner and outer body surfaces and thus provide a covering for the body and its internal organs and serve as selective barriers, facilitating or preventing the transfer of substrates across the surfaces which they cover. Some epithelia, such as the skin, protect underlying tissue from dehydration, chemical or mechanical injury, whilst other epithelia act as sensory surfaces, a function best illustrated by neural tissue, which is a modified form of epithelium.
Classification of the epithelia
The polygonal, diverse shape of epithelial cells is partly determined by their cytoplasmic contents and partly by pressure and the functional demands of the surrounding tissue (Bannister et al 2008). The conventional classification of the epithelia takes into consideration their structural and functional characteristics.
Simple epithelia
Simple epithelia are formed by single layers of cells resting on a basal lamina formed of filamentous proteins and proteoglycans. They are subdivided according to the shape of their cells, which may be columnar, cuboidal, pseudostratified or squamous. The cellular shapes are largely related to cell volume. Where cells are small, the volume of the cytoplasm is relatively low, denoting few organelles and low metabolic activity. Conversely, highly metabolic epithelial cells generally form secretory cells containing abundant mitochondria and endoplasmic reticulum and are tall, cuboidal or columnar. Simple epithelia are capable of special functions and help to form cilia, microvilli, secretory vacuoles or sensory features.
Stratified squamous epithelia
Stratified squamous epithelia consist of superficial cells, which are constantly replaced by their regenerating basal layers. These epithelia consist of flattened, interlocking, polygonal cells. Their cytoplasm may sometimes not exceed 0.1 mm in thickness and their nucleus may bulge into the overlying space. As the stratified squamous epithelium is so thin, it is ideally suited to facilitating diffusion of gases and water. However, it is also engaged in active transport, a role indicated by numerous endocytic vesicles. The most critical positions for stratified squamous epithelia are in the lining of the lung alveoli, the glomeruli and the thin segments of the loop of Henle.
Cuboidal and columnar epithelia
Cuboidal and columnar epithelia consist of regular rows of cylindrical cells. Commonly, the free surfaces of columnar cells have microvilli suited to their absorptive role in the small intestine, where they enhance the surface area for absorption of water and nutrients. By contrast, the columnar epithelium of the gall bladder displays a brush border, essential to the concentration and storage of bile. Ciliated columnar epithelium is found in most of the respiratory tract, the lining of fallopian tubes and uterine cervix. Large cuboidal cells are found in the proximal and distal convoluted segments of nephrons, where they form a brush border which selectively reabsorbs substances from the filtrate into the renal medullary interstitium.
Transitional epithelium
The characteristic feature of transitional epithelium is its thickness, formed by an extended arrangement of 4–6 cells held together in a specific arrangement by numerous desmosomes (filamentous structures). In stretching, these cells flatten without altering their position relative to each other. Most of these epithelial cells are attached to their basal lamina by slender processes forming a basal structure where they appear cuboidal and uninucleate when relaxed. At the surface of this multilayered epithelium, cells progressively fuse to form larger, and sometimes binucleate, polyploid cells with a plasma membrane covered by glycoprotein particles. This cellular arrangement has two roles:
• It facilitates expansion and contraction, stretching considerably without losing structural integrity.
• It provides an impermeable lining for organs that hold liquid containing toxic metabolic end-products such as urea and uric acid and high concentrations of salts such as potassium and sodium.
Transitional epithelium is invaluable in forming an impermeable lining in the genitourinary tract.
Complex structures derived from epithelium
Complex organ structures derived from epithelia retain familiar cellular complex characteristics; for instance, the capacity of the liver or the placenta to absorb, secrete and transport a diversity of substrates. Similarly, the diverse forms of neural tissue are functional modifications of epithelia. Most neural tissue is differentiated into conducting and non-conducting cells which provide a complex network for processing and managing information by signal transduction.
Cellular organisation
A typical cell includes a single nucleus, cytoplasm and a cellular boundary known as the plasma membrane. The different substances making up the cell are collectively known as cytoplasm or protoplasm and are composed predominantly of water, electrolytes, proteins, lipids and carbohydrates, all of which play crucial roles in shaping the cell and its organelles. These include the cell membrane, the nuclear membrane, the endoplasmic reticulum, Golgi apparatus, mitochondria, lysosomes and centrioles.
The plasma membrane
Plasma membrane is the most common feature of all cells (Alberts et al 2008). An appropriate plasma membrane is crucial to the survival and function of each cell. It encloses the cellular contents, defines cellular boundaries and maintains the essential biochemical differences between the cytosol and the extracellular environment. Plasma membranes are dynamic structures capable of considerable adaptation due to the ability of most of their molecules to move about within the plane of the membranes.
The lipid bilayer
The plasma membrane (Fig. 2.2) consists of a complex lipid bilayer interspersed by a range of protein molecules (Pollard & Earnshaw 2008). This complex arrangement of lipid and specialised proteins is held together predominantly by non-covalent interactions (Ch. 1) which occur in an aqueous environment.
![]() |
Figure 2.2 Diagram of the fluid mosaic model of cell membrane structure. (From Hinchliff S M, Montague S E 1990, with kind permission of Elsevier.) |
The lipid bilayer is composed almost entirely of fatty acids, made up of phospholipids and cholesterol. Phospholipids are small molecules constructed mainly from fatty acids and glycerol. The glycerol is joined by two rather than the three fatty acid chains that are characteristic of triacylglycerols. The third position (site) on the glycerol molecule is linked to a hydrophilic phosphate group, which is in turn attached to a small hydrophilic compound such as choline. Each phospholipid molecule has a polar head group and two hydrophobic hydrocarbon tails consisting of fatty acids that differ marginally in length. The differences in the length and saturation of the fatty acid tails allows the phospholipid molecules to stack against one another and construct a functional ‘fluid’ framework which offers different physical and chemical properties to those of the hydrophobic triacylglycerols (Alberts et al 2008).
The lipid molecules are arranged in a continuous double layer about 5–7 nm thick. The hydrophobic portions of the phospholipid face each other, whereas the hydrophilic components make up the plasma membrane surface, which is in perpetual contact with the surrounding interstitial and intercellular fluid. This lipid bilayer is highly permeable to lipid-soluble substances, such as hormones, corticosteroids, alcohol, oxygen and carbon dioxide but is relatively impermeable to most water-soluble molecules such as inorganic ions and glucose.
Lipid molecules are insoluble in water which is crucial to their grouping spontaneously to form the bilayers in aqueous solutions whilst dissolving readily in organic solvents. Furthermore, in an aqueous environment these lipid molecules aggregate allowing their hydrophobic tails to be buried in the dry, water-free interior of the plasma membrane. Thus lipid bilayers form sealed compartments ensuring that the hydrophobic tails are not in contact with water. If there is damage to the plasma membranes the lipid molecules rapidly reseal the cavity and avoid exposure of the fatty acid tails to water.
Plasma membrane fluidity is important to the survival of the cellular infrastructure and the capacity of the membrane to sustain selective transport processes and enzyme activities. However, these complex membrane functions are also dependent on the presence within the lipid bilayer of cholesterol, glycolipids and glycoproteins. Cholesterol molecules stabilise the lipid bilayer rendering it less deformable, reducing its permeability to small water-soluble molecules and preventing the hydrocarbon chains from coming together and crystallising and damaging plasma membrane functional integrity. Glycolipids and glycoproteins act as receptors for extracellular biochemical products.
The membrane proteins
The proteins suspended within, or found on the surface of, the lipid bilayer are mostly glycoproteins, which mediate many of the selective plasma membrane functions. Although these proteins are functionally highly specific they can be classified as integral or transmembrane proteins, which protrude through the plasma membrane, and peripheral proteins, which attach to the inner surface of the membrane.
The transmembrane proteins or integral proteins have a unique orientation in the plasma membrane, reflecting the asymmetrical manner in which the protein is synthesised in the endoplasmic reticulum and inserted into the lipid bilayer. Membrane proteins do not flop across the plasma membrane but rotate about an axis perpendicular to the plane of the lipid bilayer. They cross the bilayer more than once folding into distinctive α or β strands (Pollard & Earnshaw 2008).
The asymmetrical construction of lipids and proteins found on the outer and inner surfaces of the membrane facilitates the variety of functions including the control of lipid and protein diffusion. For example, the epithelial cells lining the intestinal tract confine some of the plasma membrane transport proteins to the apical surface of the cells, whereas others are confined to the basal and lateral surfaces. This suggests that cell membranes confine specific proteins to functionally distinctive domains. Whilst many peripheral proteins attach to one of the integral proteins, other proteins form structural links connecting the plasma membrane to the cytoskeleton or the extracellular matrix of adjacent cells. A few peripheral proteins serve as specialised ligand-sensitive receptors used for detection and transduction of local chemical signals.
The quantities and types of plasma membrane proteins vary in keeping with cellular functions. For instance, the neural myelin membrane serves mainly as an electrical insulation for nerve cell axons; consequently less than 25% of the membrane mass consists of protein. Conversely, mitochodrial membranes involved in energy transduction consists of approximately 75% protein. Generally, plasma membrane protein content averages 50% of its total membrane mass (Alberts et al 2008). As protein molecules are much larger than lipid molecules there are fewer of them than lipid molecules in most plasma membranes. There may be 50 lipid molecules for each protein molecule in a membrane that consists of 50% protein by mass.
Concept of selective permeability
Selective permeability of the plasma membrane facilitates free passage of some gases such as oxygen, and water, but restricts the movement of larger ions such as sodium, potassium, calcium, chloride and bicarbonate to their specific protein channels. These open or close in order to regulate transmembrane ion traffic and most integral proteins form pores through which the water-soluble substances such as ions diffuse passively. The selective passage of many other substances of larger molecular weight, such as glucose and amino acids, is also limited to protein channels, most of which are ion- or substrate-specific. This contrasts with lipid-soluble substances, such as steroid hormones, which diffuse unhindered through the lipid portion of the plasma membranes.
Cells also take up larger molecules and transport them into other cellular regions by endocytosis. This involves the invagination of small segments of plasma membrane to create vacuoles or endocytic vesicles
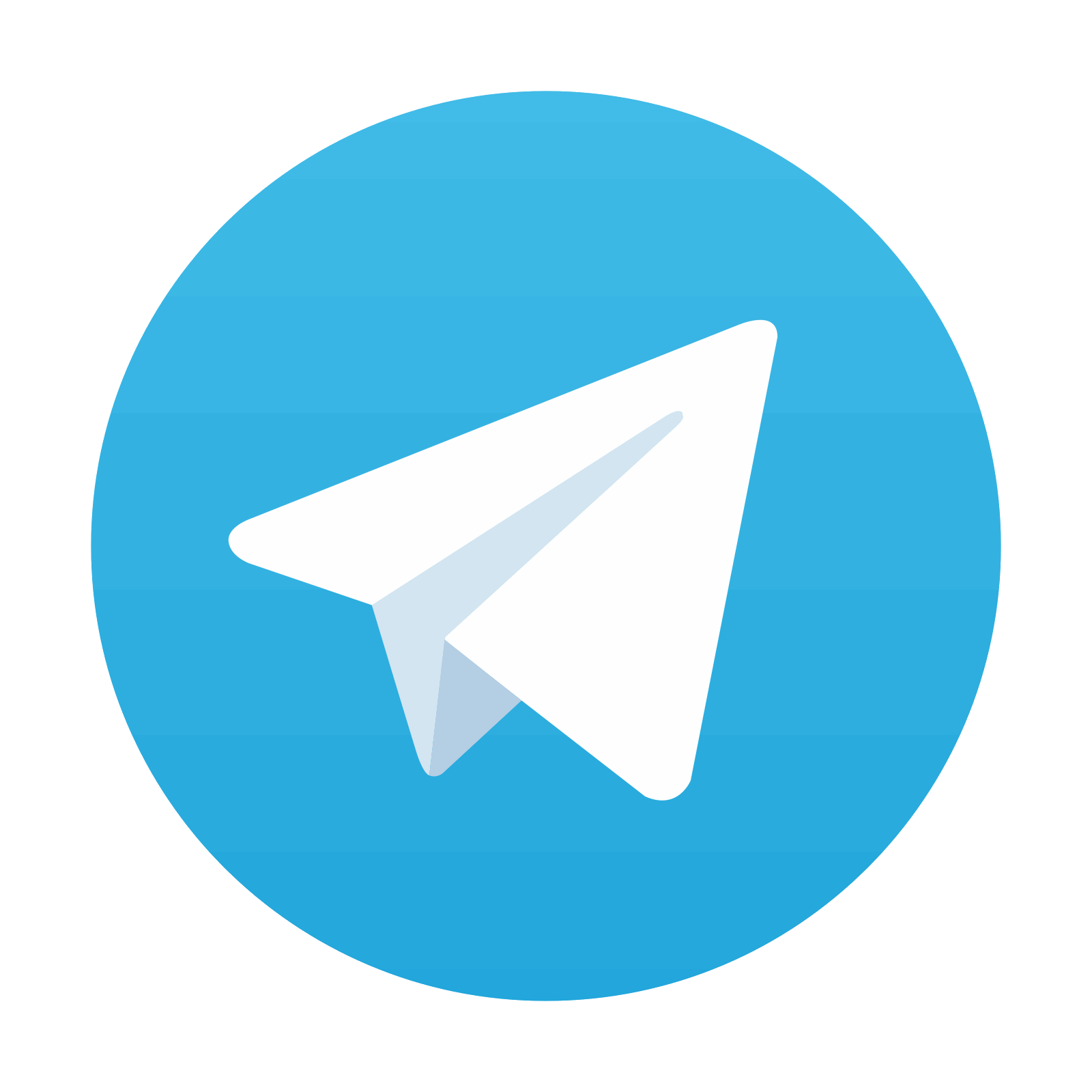
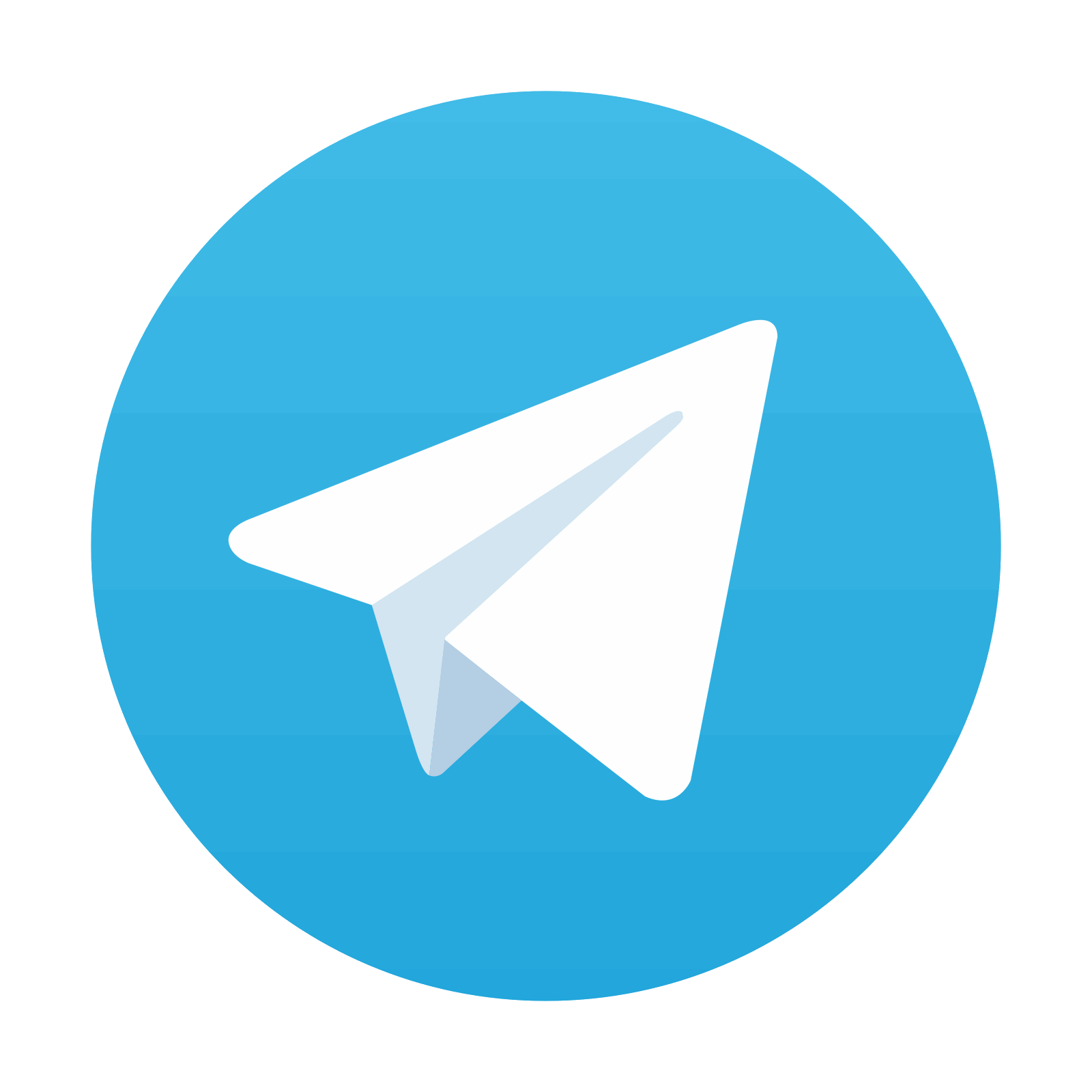
Stay updated, free articles. Join our Telegram channel

Full access? Get Clinical Tree
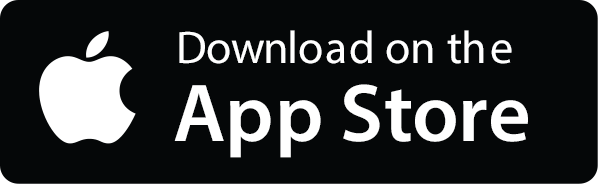
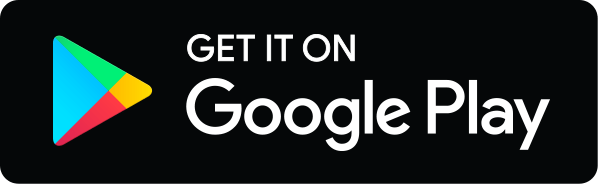
