!DOCTYPE html PUBLIC “-//W3C//DTD XHTML 1.1//EN” “http://www.w3.org/TR/xhtml11/DTD/xhtml11.dtd”>
|
Matthew A. Wikler, Franklin R. Cockerill III, and Paul G. Ambrose |
DEFINITION AND CLINICAL UTILITY OF ANTIMICROBIAL BREAKPOINTS
A breakpoint, in its simplest terms, represents the concentration of an antimicrobial agent that separates populations of microorganisms. Breakpoints are used in many ways, and so there may be more than one breakpoint for a specific antimicrobial agent–microorganism combination. It is also of interest that a breakpoint may change from time to time for a variety of reasons, as discussed later. In addition, breakpoints can vary from one country to another and from one official body to another in the same country. For example, in the United States, both the Clinical and Laboratory Standards Institute (CLSI), formerly the National Committee for Clinical Laboratory Standards (NCCLS), and the U.S. Food and Drug Administration (FDA) may provide breakpoints for the same antimicrobial agents. Additionally, breakpoints in the European Union (EU) are set by the European Committee on Antimicrobial Susceptibility Testing (EUCAST). The determination of a specific breakpoint is not a black-and-white decision, as many factors must be considered when selecting breakpoints.
To assist physicians in selecting antimicrobial agents to treat patients, clinical microbiologists categorize clinical isolates as drug susceptible, drug intermediate, or drug resistant. A result of “susceptible” assumes that an infection due to the isolate may be appropriately treated with the dosage of an antimicrobial agent recommended for that type of infection and infecting species. A result of “resistant” assumes that the isolate is not inhibited by the usually achievable concentrations of the agent with normal dosage schedules and/or falls in the range where specific microbial resistance mechanisms are likely and clinical efficacy has not been reliably attained in treatment studies. A result of “intermediate” assumes that an infection due to the isolate may be appropriately treated in body sites where the drugs are physiologically concentrated or when a high dosage of the drug can be used. The category of intermediate is also used as a “buffer zone” to prevent small, uncontrolled technical factors from causing major discrepancies in interpretations (1).
Ultimately, the purpose of breakpoints is to provide clinicians with information to assist in making decisions about antimicrobial treatments for patients with infections. Breakpoints serve many purposes, some of which are important for an individual patient, others for epidemiologic reasons. If breakpoints did not result in better patient care, then there would be little need to determine them other than as an academic exercise.
The remainder of this chapter focuses on breakpoints for bacteria, as these are currently the most advanced; however, many of the principles apply to the setting of breakpoints for other types of microorganisms (e.g., fungi).
HISTORICAL EVOLUTION AND CURRENT CRITERIA FOR ESTABLISHING BREAKPOINTS
CLSI has been providing standards for the testing of bacteria and breakpoints since 1975. Initially, breakpoints were established by examining scatterplots of the distributions of bacterial isolates versus the results of susceptibility testing conducted with antibacterial agents. Such scatterplots would frequently divide the bacterial isolates into two populations, one of which would appear to be more susceptible to the antibacterial agent being tested, the other less susceptible. The breakpoint would be the drug concentration separating the two populations. Establishing breakpoints in such a manner is probably suitable for epidemiologic purposes, as it allows one to easily determine shifts in the populations of organisms and to identify the emergence of resistant populations. From a clinical perspective, however, such an approach does not take into account the clinical implications.
In an attempt to improve the process for establishing breakpoints, CLSI has provided specific guidelines contained in a special document. The first version of this document was published in 1994 (2) and introduced the concept of looking at other types of data, including clinical data, in an attempt to correlate proposed breakpoints with what is likely to occur in the clinical setting. A revision of this document was published in 2001 (3). At the current time, so-called clinical breakpoints (antimicrobial susceptibility test interpretive categories) are determined by CLSI utilizing the following types of information: microbiologic data, animal modeling data, pharmacokinetic (PK) and pharmacodynamic (PD) modeling data, and human clinical data. These data are all considered and compared one against the other. In an ideal world, these data would all correlate with one another so that a breakpoint could be determined with certainty.
The microbiologic data considered consist of distributions of bacterial isolates and their minimum inhibitory concentrations (MICs). Numerous distributions are evaluated including those for a broad spectrum of organisms against which the antimicrobial agent is likely to be utilized and those for select populations of organisms that have specific types of resistance mechanisms. As clinical studies are conducted with a new antimicrobial agent, the susceptibility patterns observed in actual patients enrolled in studies are also reviewed. By utilizing data of this type, one can gain a sense of the various populations of organisms that exist and their relative susceptibility to the antimicrobial agent. Animal studies are quite useful in determining which pharmacokinetic-pharmacodynamic (PK-PD) measure one should be evaluating when trying to predict clinical efficacy. The three most common PK-PD measures are the duration of time the drug concentrations remain above the MIC (T>MIC), the ratio of the maximal drug concentration to the MIC (Cmax:MIC ratio), and the ratio of the area under the concentration time curve at 24 hours to the MIC (AUC0-24:MIC ratio). For most classes of antimicrobial agents, animal studies have demonstrated the ability to predict clinical efficacy by examining specific parameters (4). For example, it has been clearly demonstrated for β-lactam antibiotics that the most critical parameter predictive of clinical outcomes is the time that free drug plasma concentrations remain above the MIC of the causative organism (5–7). This is an example of a class of antibiotics where the predictive parameter is “time dependent.” For cephalosporin antibiotics and Streptococcus pneumoniae, it appears that clinical success is likely if the free drug concentration above the MIC of the causative organism is maintained for 40% to 50% of the dosing interval, while for penicillins, the target appears to be 30% to 40% of the dosing interval (4).
The area under the drug concentration time curve (AUC) is a measure of drug exposure. Mathematically, the AUC is calculated as the integral of the drug concentration time curve. Response to drugs in vivo can usually be linked to the AUC. In some instances, the shape of the concentration time curve can affect in vivo response to a drug, and thus other measures of exposure (e.g., Cmax, Cmin) can also be important. Fluoroquinolone antibiotics have been demonstrated in animal models to be “concentration dependent”; that is, clinical outcomes can be predicted based on the AUC:MIC ratio and/or by the Cmax:MIC. For the fluoroquinolones, it appears that clinical success (8,9) depends on attaining a free drug AUC:MIC ratio of around 30 for gram-positive organisms and around 100 for gram-negative organisms.
Although these general PK-PD targets tend to apply to many types of infections, one must keep in mind that levels obtained in certain tissues and body fluids may result in these targets not being predictive. For example, for drugs that are excreted by the kidneys and where active drug is concentrated in the urine, one would anticipate the ability to successfully treat organisms in the urinary tract with higher MICs. On the other hand, most drugs do not achieve high levels in the cerebrospinal fluid (CSF), and so one would anticipate that higher doses of an antimicrobial agent may be required to adequately treat an infection in that site. It would not be sufficient only to determine PK-PD targets based on responses in animal models, and so it is important that the results of clinical studies be correlated with these targets. Such work has been done for many classes of antimicrobial agents (4,8,10–14). Unfortunately, for new classes of antimicrobial agents, including LpxC inhibitors (deacetylase inhibitors of endotoxin biosynthesis), topoisomerase type-B subunit inhibitors, β-lactam–β-lactamase inhibitors, the correlations between the targets and the clinical outcomes in humans have yet to be well studied.
Once the PK-PD measure and target predicting clinical success has been identified, one can integrate this information with human PK data to estimate the probability of attaining drug exposures sufficient across a range of MIC values. One of the best ways to conduct such an analysis is by using Monte Carlo techniques (15,16). Basically, the strategy is to take (a) the PK parameters along with anticipated variability and (b) the MIC values of organisms likely to cause an infection along with the proportion of time; a specific MIC value is achieved or exceeded by in vivo concentrations of antimicrobial agent and then model patients by randomly matching up PK profiles and MICs. By using such a technique, one can easily simulate 5,000 or 10,000 patients and make predictions as to what MICs one is likely to be able to treat successfully with various dosing regimens (17,18). Ideally, this process should be accomplished in the earliest stages of drug development, as this allows one to determine the optimal dosing regimen likely to result in a successful clinical outcome while minimizing the potential for toxicity. Such PK-PD modeling can also be utilized to justify the initial breakpoint for a new antimicrobial agent prior to the availability of a large amount of clinical data (19,20).
Ultimately, the purpose of breakpoints is to provide information to the clinician for the selection of optimal antimicrobial therapy. Because of this, it is critical to evaluate clinical data from well-designed clinical studies which correlate breakpoints with clinical outcomes. Unfortunately, this is far from an exact science, as there are many factors that determine clinical outcomes other than the antimicrobial agent used. Consequently, clinical correlations are generally used to confirm susceptibility breakpoints predicted by the previously mentioned techniques and data.
For many reasons, the true limits of an antimicrobial agent are rarely tested in clinical studies conducted for the purpose of gaining regulatory approval. First, most of these studies exclude or discontinue patients whose infections are caused by organisms with an MIC above a tentative breakpoint. As a result, even if clinical studies could demonstrate a breakpoint, the probability of this happening is greatly reduced. In most cases, clinical studies can be ethically designed in a manner that would blind the investigator to susceptibility test results, allowing the decision to continue or discontinue therapy with the study drug to be determined by clinical and microbiologic responses. Clinical studies so designed are more likely to aid in determining a clinical breakpoint. Another reason that breakpoints often fail to be determined by clinical data is that many of the newer antibiotics being developed are quite potent and only a small percentage of organisms will have MICs high enough to truly test their limits. In fact, few or no patients may be enrolled who have infections due to organisms with MICs at a sufficient level to uncover the limits of the antibiotic being evaluated.
ESTABLISHING BREAKPOINTS BY SITE OF INFECTION
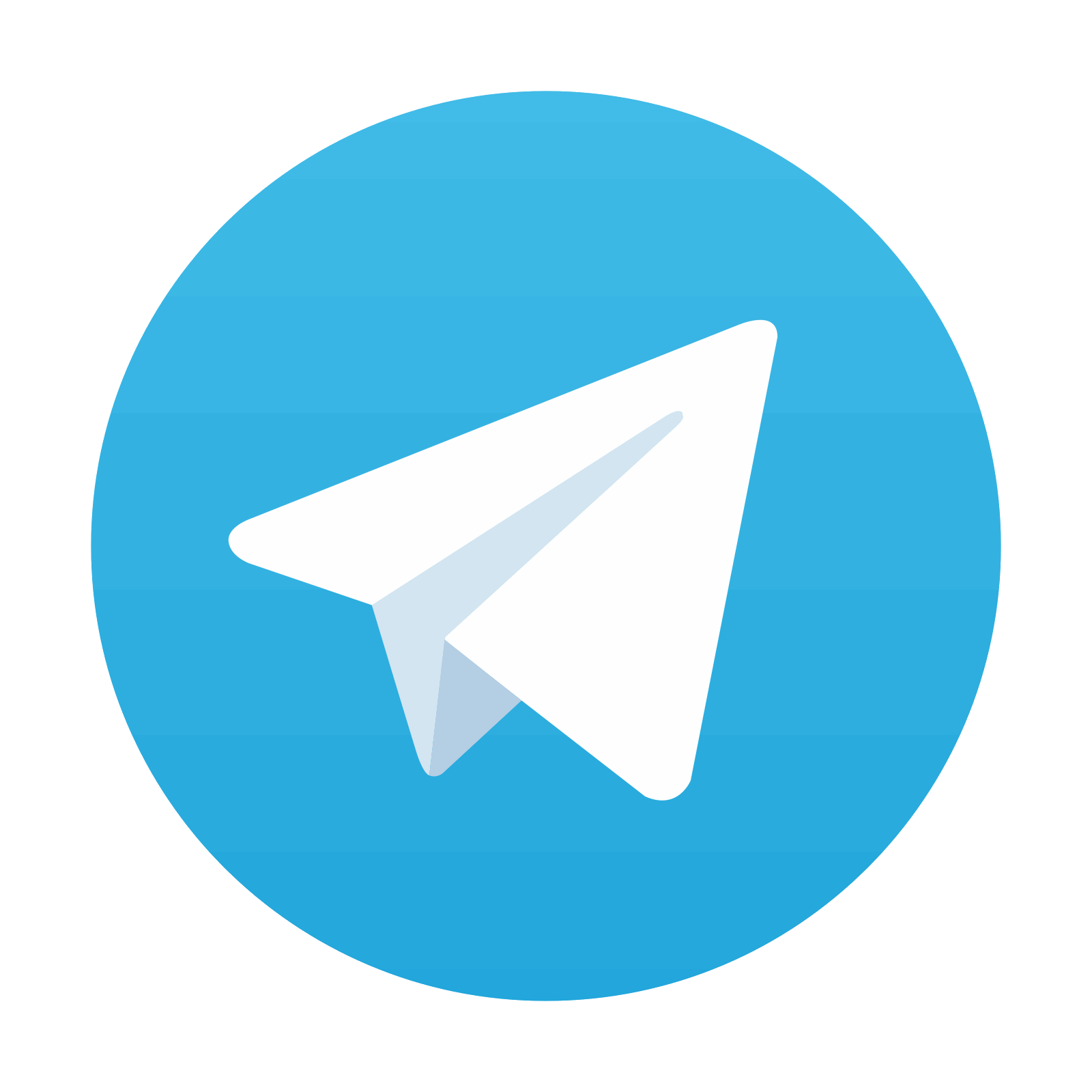
Stay updated, free articles. Join our Telegram channel

Full access? Get Clinical Tree
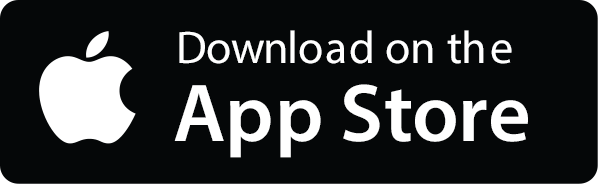
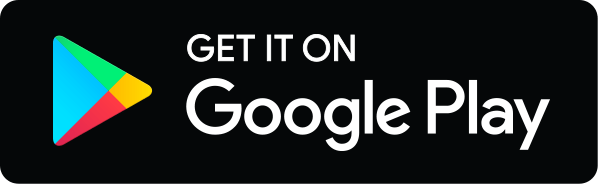