3
CHAPTER OUTLINE
Multiple brain structures are involved in the addiction process. Drug addiction involves increased and compulsive drug taking—often in the face of adverse consequences and at the expense of more socially or biologically important behaviors. Understanding the neurobiology of this phenomenon involves, at the most basic level, the identification of the neurotransmitter systems that mediate the reinforcing effects of specific drugs. However, to understand how the behavioral repertoire becomes subverted, it is necessary to consider the structures involved in decision making and in generating motivated behavior.
For the most part, the discussion of the anatomy of reinforcement and addiction maps onto structures associated with the limbic system and the basal ganglia. The first section of this chapter offers a simplified overview—a primer—of the structures involved and their interconnections. The second section, entitled Neuroanatomy of Drug Reinforcement, focuses on the systems associated with the primary reinforcing effects of psychostimulants, opioids, and cannabinoids. To the extent that drug taking is a reinforced behavior, it is critical to understand the neural mechanisms that underlie drug reinforcement. We focus on the concept of site of action, which defines the access points for a drug to influence a specific brain process. Another level of analysis is the study of how a reinforcing drug action influences other brain areas involved in the organization of the behavioral repertoire. This more broadly defined topic is the subject of the third section entitled Neuroanatomy of Drug Addiction.
PRIMER ON NEUROANATOMY
The structures most often mentioned in the context of drug abuse are closely associated with the limbic system, lateral hypothalamus, basal ganglia, and frontal cortical regions. Here we present a brief description of each of these systems. Readers interested in a more complete description of the anatomy of these regions are referred to the following reviews (1–3).
Limbic System
Research into the limbic system has a long and venerable history, but some scholars have suggested that the term may have outlived its usefulness. Nonetheless, a brief historic review of the term is a useful way of introducing some of the regions involved in drug addiction. The term limbic is derived from the Latin term limbus, meaning border, and was used to describe a ring of phylogenetically older cortex that separates the diencephalon and the neocortex. This limbic lobe consisted of the subcallosal area, cingulate, and parahippocampal gyri (Fig. 3-1). This purely anatomic distinction was expanded by MacLean (4) in 1952 to describe a functional unit that was proposed to be responsible for emotional expression. He made the distinction between the older, medial cortex and the more lateral neocortex, which is involved in cognitive functions.
FIGURE 3-1 Regions of the human brain associated with the limbic system, which includes a loop of cortex extending from the subcallosal region through cingulate cortex to the parahippocampal gyrus. Also shown are the hippocampal formation, septum, amygdala, and mammillary bodies.
MacLean’s concept was that most human behavior is the result of cooperation between three systems of the brain. The cerebral cortex is responsible for higher-order reasoning and speech, whereas the limbic system was the source of emotions, aspects of personal identity, and fight or flight instincts. The third of MacLean’s system is the reptilian brain. Early work with primates showed that when various parts of the limbic system were electrically stimulated, a range of emotional responses was produced, such as rage, fear, and joy (5). This phylogenetically older brain is responsible for the organism avoiding things that are “disagreeable” and approaching those that are “agreeable”—reactions that MacLean saw as having survival value. It is now clear that structures associated with the limbic system (such as the hypothalamus, hippocampus, and amygdala) are essential not only for learning and memory but also for the emotional context and the affective response to learned associations.
As is detailed, many drugs of abuse have their sites of action within the limbic system, and the neurochemistry within these structures is altered during the addiction process. This may help explain why decisions surrounding drug seeking and drug taking seem to be driven more by emotion and instinct rather than by logic.
From an anatomic perspective, MacLean defined the limbic system as the original limbic lobe along with other structures sharing direct connections with them. These include the olfactory cortex, hippocampal formation, amygdala, septum, hypothalamus, habenula, anterior thalamic nuclei, and parts of the basal ganglia. With further anatomic research, more and more areas were shown to share direct connections with these structures and some of these began to be included in the limbic system. The result was that the boundaries of the limbic system became overly broad. Brodal (6) observed that the term limbic system was becoming less useful, and he argued that it should be discarded altogether; however, the concept of a phylogenetically older forebrain system responsible for emotional control is now firmly entrenched.
Swanson (7) has helped crystallize the anatomic definition by characterizing the limbic system as a network of highly interconnected regions that appear to form the only major route for information transfer between the neocortex and the hypothalamus. Figure 3-1 illustrates these areas in the human brain.
Interface of the Limbic System and the Basal Ganglia
The basal ganglia is traditionally thought of as a motor system; however, the idea that this system deals only with motor function while the limbic system deals with reinforcement and emotion is oversimplified and entirely misleading. As later sections illustrate, there is reason to believe that parts of the basal ganglia are very much involved in memory formation and a variety of cognitive tasks. As more is learned about how the basal ganglia and limbic system communicate, it is becoming increasingly clear that the two systems are jointly involved in coordinating motivated behavior.
The largest mass associated with the basal ganglia is the striatum (caudate–putamen). The dorsal portion has long been considered part of the basal ganglia, whereas the ventral striatum is considered to be part of the limbic system (8). This dorsal/ventral distinction is clearly important; however, more recent debate has focused on the idea that gradations rather than sharp boundaries mark the transition from the dorsolateral to ventromedial striatum. Figure 3-2 shows the topographic organization of inputs to the striatum in the rat. Voorn et al. (9) make the point, as Figure 3-2 illustrates, that no histologic or immunohistochemical border exists that can be used to define or divide the region. Note that although the dorsal–lateral parts receive primarily motor inputs and the ventromedial striatal areas receive primarily limbic projections, it is a gradual topographic transition.
FIGURE 3-2 Connections of the dorsal and ventral striatum. Cortical and thalamic inputs to the striatum distribute in a dorsomedial-to-ventrolateral manner. To paraphrase Voorn et al. (9), afferents arising from the frontal cortex and projecting to the striatum are depicted. Sensorimotor projections innervate the dorsolateral striatum in a somatotopic fashion. Vicerolimbic inputs project to the ventromedial striatum. Areas in between dorsolateral and ventromedial striatum receive inputs from higher cortical association areas: ac, anterior commissure; ACd, dorsal anterior cingulate cortex; AId, dorsal agranular insular cortex; AIv, ventral agranular insular cortex; IL, infralimbic cortex; PFC, prefrontal cortex; PLd, dorsal prelimbic cortex; PLv, ventral prelimbic cortex; SMC, sensorimotor cortex. (Adapted from Voorn P, Vanderschuren LJ, Groenewegen HJ, et al. Putting a spin on the dorsal-ventral divide of the striatum. Trends Neurosci 2004;27:468–474.)
The accumbens forms the ventral portion of the striatum, thus accumbens and ventral striatum are used synonymously in the addiction literature. Famously, in 1993, Mogenson et al. (10) described the ventral striatum as the crossroad of the limbic and motor systems and “the place where motivation is translated into action.” Figure 3-2 also shows two regions identified as the nucleus accumbens (NAcc) core and shell. The involvement of these two regions in the behavioral and electrophysiologic responses to drug reinforcement has been extensively studied, and some debate has focused on which area is responsible for particular drug effects. Some of the core–shell debate might eventually give way to discussion of the function of subregions defined by projections.
In the primate, as one may expect, the organization of these striatal connections is complex. Haber et al. (11) have described the topography of striatonigrostriatal circuitry, that is reciprocal synaptic connections between the striatum and substantia nigra pars compacta/reticulata, and proposed how this pattern of connections may provide a means through which information flow between striatal regions can be achieved (Fig. 3-3). This circuitry can be conceptualized as a series of ascending spiraling connections between adjacent striatal regions via the ventral midbrain (substantia nigra), so that the accumbens shell influences the accumbens core, the core influences the central striatum, and the central striatum influences the dorso-lateral striatum in turn.
FIGURE 3-3 Striatonigrostriatal connections form a series of ascending spiraling loops. Projections from the NAcc shell terminate in both the VTA and ventromedial SNc. The VTA sends reciprocal projections back to the shell and neurons from the medial SN project forward to the NAcc core. The projections continue to go back and forth between the striatum and substantia nigra, spiraling upwards, so that the shell influences the core, the core influences the central striatum, and so on. These striatonigrostriatal connections continue to occur even past the anterior commissure (top right schematic). These posterior portions of the caudate and putamen have reciprocal connections with the dorsal portions of the SN. VTA, ventral tegmental area; SNc, substantia nigra pars compacta; SNr, substantia nigra pars reticulate. (Adapted from Haber SN, Fudge JL, McFarland NR. Striatonigrostriatal pathways in primates form an ascending spiral from the shell to the dorsolateral striatum. J Neurosci 2000;20:2369–2382.)
Executive Function and Cortical Involvement
Executive function involves control over many aspects of behavior. Some of these include the ability to differentiate among conflicting thoughts, determine good and bad, better and best, same and different, future consequences of current activities, working toward a defined goal, expectation based on actions, behavioral inhibition, and social “control.” The prefrontal cortex (PFC) is thought to be the “hub” of executive function in the brain.
The PFC in humans is subdivided into three main regions: (i) the orbitofrontal and the ventromedial areas, which are thought to be involved in processing reward (12); (ii) the dorsolateral PFC, more broadly involved in decision making (13); and (iii) the anterior and ventral cingulate cortex, which helps to control whether or not a particular behavior will be performed and to what intensity (14).
NEUROANATOMY OF DRUG REINFORCEMENT
“Site of action” is a pharmacologic concept that defines the access point for a drug to produce a specific response. If that response is defined behaviorally (e.g., anorexic, convulsant, antidepressant effect), then the site of action identifies the receptors and brain regions responsible for that particular behavioral response. It is one thing to describe all possible sites where a drug can affect the brain; it is a more difficult matter to narrow down the possibilities to a particular binding site in a circumscribed region. Research into the site of action for the reinforcing effects of psychostimulant drugs, such as cocaine and amphetamine, offers a good example of how this investigative process occurs.
In vitro experiments have shown that cocaine binds to dopamine, noradrenaline, and serotonin (DA, NA, and 5-HT) transporters and blocks the reuptake of these neurotransmitters (15); amphetamine acts additionally as a releasing agent. Both of these actions result in an increased concentration of monoamine neurotransmitters in the synapse. Therefore, psychostimulant drugs act as indirect agonists everywhere these transmitters are found.
An examination of the anatomic projections of the catecholamine systems shows that they have extensive and diffuse projections throughout the neural axis. The cell bodies of these transmitter systems are loosely organized in an anteroposterior fashion. Based on their histochemical mapping studies, Dahlstrom and Fuxe (16) proposed a nomenclature wherein cell clusters were numbered from posterior to anterior and given a letter prefix according to whether they were dopaminergic/noradrenergic (A) or serotoninergic (B). Figure 3-4A shows the distribution of NA fibers. The locus coeruleus (LC) (A6) is located in the dorsal brain stem and sends ascending projections to terminal regions of the cortex, hippocampus, and cerebellum. More caudal and ventral NA cells groups (A1–A5) innervate the hypothalamus and brain stem. Dopaminergic innervations are more circumscribed (Fig. 3-4B). DA cell groups within the ventral tegmental area (VTA) and substantia nigra (A8, A9, and A10) project in a topographic manner to the striatum. The more medial group (A10) sends projections to the ventral striatum, whereas the more lateral groups form a nigrostriatal bundle that innervates the caudate–putamen. This latter projection is known to degenerate in Parkinson disease and is thus associated with motor function. An additional cluster of DA cells in the hypothalamus composes the tuberoin-fundibular DA system, which innervates the external layer of the median eminence. Dahlstroem and Fuxe (17) also described 5-HT cell groups (B1–B9), which lie near the mid-line of the pons and upper brain stem. Later studies showed clusters of cells also in the caudal LC, area postrema, and interpeduncular nucleus (14). Generally, the more caudal cell groups innervate the medulla and spinal cord, whereas the more anterior clusters project rostrally (Fig. 3-4C).
FIGURE 3-4 Schematic diagram illustrating the distribution of the main central neuronal pathways containing noradrenaline (A), dopamine (B), and serotonin (C). The location of cell bodies of origin is indicated by circles with the projections indicated by arrows.
The main point to be taken from an examination of the areas innervated by DA, NA, and 5-HT is that there is hardly a region that is not innervated by at least two of the mono-amines. Given that psychostimulants have an effect at the terminal regions of each of these systems, every area of the brain would be expected to be affected to some extent by an injection of cocaine or amphetamine. It has been a considerable challenge, therefore, sorting out what transmitter in which particular area produces toxic effects and adverse reactions on the one hand and pleasurable or positive reinforcing effects on the other.
Preclinical Studies of Drug Reinforcement
Experiments with nonhuman primates and a variety of other laboratory animals have helped identify the important sites of action for drug reinforcement. It is important to note that, early on, there was considerable skepticism whether anything useful could be learned from laboratory animals regarding human drug taking. Given the premise that only humans abuse drugs, along with the observation that what distinguishes the human brain from other mammals is the development of the neocortex, the logical conclusion was that this region is responsible for drug addiction. This idea fit well with the idea that drugs were “mind expanding” and that the reasons why a person might take drugs has to do with their effects on consciousness. Interviews with drug users and introspective analysis of drug-taking behavior suggested that some of the reasons that people take drugs is for “pleasure, curiosity, the desire to experiment, the sense of adventure, the search for self-knowledge, the relief of stress and tension, depression, the feeling of powerlessness, and the lack of belief in the future” (18). Although these observations are entirely appropriate for discussion at one level, they also serve to perpetuate the idea that human consciousness and reasoning are the bases for drug reinforcement. The demonstration that nonhuman primates and rodents will voluntarily self-administer drugs such as cocaine and heroin has prompted a consideration of concepts other than human consciousness to account for drug abuse, forcing an examination of brain regions other than the cortex.
There is an extremely high correlation between the drugs that are abused by humans and drugs that are self-administered by other mammalian species such as rat, dog, cat, rabbit, and nonhuman primate (19,20). These data support the idea that drugs of abuse have their reinforcing actions on brain structures that have been relatively conserved through the course of human evolution—that is, limbic and brainstem areas. This being the case, the development of self-administration techniques, through intravenous (IV) (21–23), intracranial (24–26), and inhalation (27) routes, has provided a means to study brain structures responsible for drug reinforcement in animal models.
Psychostimulants
Pharmacologic experiments were the first to narrow down the range of possible sites of action for the reinforcing effects of psychostimulant drugs (28,29). In these studies, rats and monkeys were trained to self-administer cocaine and amphetamine until they showed a stable baseline level of responding (Fig. 3-5). They were then pretreated with a variety of agonists or antagonists in an effort to identify the specific transmitter systems that modulate reinforcing efficacy. Importantly, it was shown that pretreatment with DA receptor antagonists caused the animals to self-administer cocaine and amphetamine more frequently (30–32). This is the same result one sees if the concentration of drug is diluted: the animals appear to compensate for the reduction in drug effect by increasing their intake. Note that pretreatment with NA or 5-HT antagonists did not have consistent effects on drug intake. These data prompted Wise (33,34) to champion the idea that stimulation of DA receptors must be essential for psychostimulant reinforcement.
FIGURE 3-5 In self-administration studies, animals are implanted with permanent indwelling catheters and placed in an experimental chamber. The catheter is connected to a syringe pump through a fluid swivel that allows free movement throughout the chamber. A computer detects responses on a lever and controls the timing of drug delivery according to the schedule of reinforcement. For example, on a fixed ratio one schedule (FR1), every response on the lever results in an infusion of drug.
Peripheral injections of DA antagonists narrowed the site of action to DA receptors, but it remained unclear which brain regions mediated this effect. Central manipulations were necessary to identify which brain regions were important. Neurotoxins specific to catecholamine and indolamine neurons provided a useful tool to examine whether the loss of a particular fiber system would affect drug self-administration. A considerable literature developed around the neurotoxin 6-hydroxydopamine (6-OH-DA) that, depending on the site of injection and other parameters, could be used to completely deplete various brain regions of either DA or NA. Early experiments showed that removing the noradrenergic innervation of the entire forebrain had almost no effect on cocaine self-administration. By contrast, removal of the DA innervation of the NAcc resulted in a substantial reduction in cocaine’s reinforcing effects (35–37). In fact, animals will no longer self-administer cocaine if DA levels in the NAcc are reduced by more than 80%. Destroying the DA neurons in the VTA also drastically reduced cocaine self-administration (38). These data were the first to draw attention to the NAcc (more recently referred to as ventral striatum) as a site for action for psychostimulant reinforcement.
6-OH-DA lesions in other brain regions have had much less dramatic effects. Lesions of the dorsal striatum do not change the rate of cocaine intake, supporting the idea that the ventral striatum has a preferential involvement (39). Additionally, destruction of DA terminals in the medial PFC or amygdala has only minor effects on the cocaine dose–response curve, which seems to reflect changes in the reinforcing threshold or a change in the anxiogenic effects of cocaine (40,41).
Injections of DA antagonists directly into the brain have also been used to identify the important anatomic sites involved in cocaine action. The evidence consistently shows that the DA receptors in the ventral striatum are an important site of action. Blockade of D2 receptors produce an apparent decrease in potency. That is, animals compensate by increasing their hourly drug intake and will “work” less hard for each injection. Injections of D2 antagonists into the dorsal striatum and the medial PFC produce similar effects, albeit less strongly (42,43).
In summary, the data presented are from a wide range of studies using neurotoxins, and specific pharmacologic agents are consistent with the idea that the most important site of action for the reinforcing effects of cocaine and amphetamine is at DA terminals in the ventral striatum. It should be emphasized however that, although the ventral striatum is essential for the reinforcing effects of cocaine and amphetamine, other DA projection areas (such as the PFC, amygdala, and dorsal striatum) also contribute to some extent.
The site of action for the locomotor-activating effects of psychostimulant drugs seems to largely overlap with the regions responsible for drug reinforcement. Experiments using either lesion or intracerebral injections of DA drugs have shown that stimulation of the DA receptors in the ventral striatum produces behavioral activating effects (locomotion); stimulation of DA receptors in the dorsal striatum produces stereotypy (44), which is characterized by repetitive sequences of movements, such as licking and grooming in rodents. 6-OH-DA–induced destruction of DA terminals in the ventral striatum almost completely abolishes the locomotor stimulant effects of cocaine, while lesions of the dorsal striatum abolish psychostimulant stereotypy. Similarly, direct injections of DA agonists into the ventral striatum produce locomotion, whereas injections into the dorsal striatum produce stereotypy (45–47).
The stereotypic response elicited from the dorsal striatum may have relevance to addiction. In rats, stereotyped behavior is often measured on a categoric scale, which includes licking, chewing, and gnawing. These high-frequency movements have the appearance of being “hard-wired” responses that are elicited by the drug. It should be emphasized, however, that almost any behavior can become stereotyped. In their influential article, Randrup and Munkvad (48) described how the precise forms of stereotyped behavior differ across species and offer the example of a man who repeatedly rebuilt his car engine. They suggested that inhibition of drug-induced stereotypy might be useful in screening antipsychotics. We know now that this is true because of the relationship between the importance of DA in stimulating stereotypy and the action of neuroleptic drugs. To reemphasize, anything can become stereotyped.
Behaviors that occur with high frequency have a high likelihood of becoming stereotyped (i.e., they occur repetitively and ritualistically) if they occur in the presence of a drug (49). Lyon and Robbins (50) argue that stereotypy is a process in which there is an increase in frequency, in a diminishing number of response categories. The effect is that psychostimulant drugs narrow the behavioral repertoire such that only a few predominant behaviors remain. Put another way, the behavioral repertoire becomes focused around the things that occur most frequently. In the case of a person with addiction, the frequent behaviors associated with drug seeking and drug taking become repetitive and ritualistic.
In conclusion, strong evidence from studies using widely different strategies suggests that stimulation of DA receptors in the ventral striatum is associated with drug reinforcement. There is a wealth of evidence from a parallel literature showing that the mesolimbic DA system is also involved in reward from natural behaviors such as feeding (51–53), drinking (54,55), sexual behaviors (56,57), and intracranial self-stimulation (58–60). This enormous amount of literature has resulted in the mesolimbic DA system being called a reward pathway. Whether this term is accurate or biologically meaningful (we think it is not) lies outside the scope of this chapter. However, the immense interest in the mesolimbic DA pathway demands some discussions of its interconnections.
Data from electrophysiologic studies show that VTA-DA neurons respond to primary reinforcing stimuli (e.g., food) and to environmental cues that predict the presentation of rewards (61,62). It is therefore surprising that these neurons receive no direct input from visual, auditory, or somatosensory systems (63). It appears instead that the VTA is a part of, and receives inputs from, a widespread collection of neurons that belong to the “isodendritic core” (64). This system is a network of neurons stretching from brainstem to telencephalon. The neurons within the network have similar morphology, send out long projects, and are themselves the target of a great number of contacts from distant sources (65). It would appear that this network serves an integrative function and responses to changes in the environment that are biologically significant. At present, it remains unclear whether the VTA-DA neurons respond differentially than others in the network and whether they are specifically activated by reward or more generally by any other important stimulus.
Opioids
Again, the process of identifying the site of action for the reinforcing effects of opioid begins with identifying all possible receptors sites. Opioid receptors are expressed throughout the brain, especially in limbic and limbic-related structures; they are found in the amygdala, insular cortex, caudate, anterior hypothalamus, cortex, parietal cortex, putamen, thalamus, and periaqueductal gray (66). There are three different types of G protein–coupled opioid receptors: μ, κ, and δ, which are acted on by both endogenous and exogenously applied opioids (67). Selective μ agonist drugs, such as morphine, heroin, and most clinically used opioid analgesics, produce analgesia, euphoria, respiratory depression, emesis, and antidiuretic effects. Selective κ agonist drugs, such as the experimental compounds ethylket-azocine and bremazocine, produce analgesia, dysphoria, and diuretic effects, but no respiratory depression. There is less known about the direct role of δ receptors. Agonists at μ receptor are more likely to have abuse liability than κ agonists (68–70). Within the dorsal and ventral striatum, there are areas of overlap between expression of opioid receptors; however, their expression patterns tend to differ. μ Receptors are expressed in patches, and κ and δ receptors are more diffusely distributed (71).
Almost all that is known about the neurobiology of opioid reinforcement is derived from animal models. Three approaches have been used to investigate the involvement of various brain regions in opioid reward: (i) intracerebral self-administration of opioid agonists, (ii) blockade of IV heroin self-administration by intracerebral injections of opioid antagonists, and (iii) disruption of IV heroin self-administration by lesions. Generally, the focus has been on areas associated with the mesolimbic DA system (ventral striatum and VTA), although other regions have also been implicated.
Self-administration of drugs directly into various brain regions would seem to be the most straightforward test of their involvement in reinforcement processes; however, the procedures have a number of technical problems that limit their appeal. Issues involving diffusion, osmolarity, and tissue damage demand thoughtful controls (see 72 for review). Nonetheless, several papers have provided evidence that opioid-like compounds are self-administered into discrete brain regions. The early work focused on the lateral hypothalamus (24,73,74) because this area was intensely studied for its ability to support intracranial electrical self-stimulation (75). Later, because of interest in the mesolimbic system, interest switched to the ventral striatum and the VTA. The role of the lateral hypothalamus has been challenged, and it is possible that the early results were due to diffusion of drug to other areas (76). The ventral striatum appears to support intracranial self-administration of morphine (77) and methionine-enkephalin (78). These data fit well with the demonstration that intra-NAcc opioids produce a conditioned place preference (79). Techniques have also been developed to study intracerebral self-injection in mice by using a Y-maze. Selection of one arm of the maze results in a morphine injection, whereas the other arm results in a saline injection. Using this method, mice have been shown to self-inject morphine into the lateral septum (80) and the ventral striatum but not the dorsal striatum (81).
By far the most sensitive site for intracerebral self-administration of opioids is the VTA. Both μ and δ opioids are self-administered into this region at doses that are not supported in other areas (26,82–84). The idea that opioids have a significant impact on reinforcement mechanisms through an action in the VTA is supported by a variety of other techniques. For example, injections of opioid agonists into the VTA also produce a conditioned place preference (85), facilitate brain stimulation reward (86,87), and reinstate extinguished lever responding that was trained under IV heroin reward (88).
It should be noted that there are a few reports of reinforcing effects produced by intracerebral injections of opioids into the hippocampus (89) and periaqueductal gray. The doses used in these studies are relatively high, and it remains unclear whether these are important but less sensitive sites or whether diffusion of the drug to other areas accounts for the data.
When self-administering IV heroin, animals respond to a decrease in the unit injection dose by taking injections more frequently. A similar phenomenon can be observed when animals are treated with a systemic injection of naloxone (a μ antagonist), suggesting that animals compensate for a reduced drug effect by increasing their intake (90). Several laboratories have used this compensatory response to evaluate the effects of opioid antagonists injected into various brain regions. Increases in IV heroin self-administration have been shown after injections of low doses of opioid antagonists into the NAcc (91–93), periaqueductal gray (92), stria terminalis (94), and lateral hypothalamus, but not the PFC (95). Surprisingly, injections of an opioid antagonist into the VTA have relatively little effect (96). It remains unclear why many studies have shown that the VTA is one of the most sensitive brain sites for intracerebral self-administration of opioid agonists, whereas it is one of the least effective sites for disrupting IV heroin self-administration studies with an intracerebral injection of opioid antagonists.
Lesions offer a third method for identifying critical brain areas responsible for the reinforcing effects of opioids. Zito et al. (97) showed that the size of a kainic acid–induced lesion of the NAcc correlated with impaired heroin self-administration. This effect is site specific because lesions of other areas, such as the lateral hypothalamus, do not necessarily affect heroin self-administration (98). More recent studies have attempted to define the relative contribution of subregions within the ventral striatum comparing acquisition of heroin self-administration after excitotoxic lesions of the NAcc core or shell. Rats with lesions of the NAcc core lesion group showed impairments in acquisition, whereas the group with lesions of the NAcc shell was similar to controls. This effect was found either with acquisition of low-dose heroin on a simple fixed ratio schedule (99) or with acquisition of a second-order schedule with a high injection dose (100). These data suggest a relatively greater role for the NAcc core in the acquisition of heroin-seeking behavior.
Martin et al. (101) examined regional differences in the ventral striatum by using beta-FNA. This drug is an irreversible antagonist at the μ-opioid receptor producing what amounts to a reversible lesion. Beta-FNA blocks the receptor rendering it unavailable for many days, until new receptor populations can be synthesized. Beta-FNA was found to produce site-specific effects, attenuating heroin self-administration when injected into the caudal but not rostral NAcc.
The hypothesis that the mesolimbic DA systems mediate the reinforcing effects of opioids has been proposed. Certainly, it is clear that psychostimulants and opioids have independent sites of action at the receptor level. DA antagonists potently affect cocaine but not heroin self-administration; conversely, opioid antagonists potently affect heroin but not cocaine self-administration (102,103). However, it has been shown that opioids indirectly affect DA cell firing through inhibition of GABA interneurons in the VTA (104). This disinhibition can result in enhanced DA release in the NAcc (105). Heroin self-administration increases DA in the accumbens, and this has been argued to be the mechanisms of action for heroin reinforcement (106).
Curiously, DA cells bodies in the VTA seem to be more important for heroin self-administration than the DA innervation of the ventral striatum. Bozarth and Wise (107) showed that 6-OHDA lesions of the VTA impair the acquisition of IV heroin self-administration. By contrast, 6-OHDA–induced depletion within of the NAcc has very little effect on heroin self-administration in spite of the fact that 6-OHDA such lesions dramatically reduce or abolish cocaine self-administration (108,109). It appears that some, but not all, of the reinforcing effects of opioids are mediated through an action on DA mechanisms.
Cannabinoids
The characterization of cannabinoid receptors in brain has been an important first step in identifying the site of action for the reinforcing effects of marijuana. Two cannabinoid receptors (CB1 and CB2) have been identified to date. Both are G protein–coupled receptors and function to inhibit adenylate cyclase. They are acted on by endogenous can-nabinoids and exogenous activators such as marijuana. Figure 3-6 represents CB1 expression in the rat brain. As in humans, the CB1 receptor is highly expressed in the brain and found in the basal ganglia, hippocampus, cerebellum, cerebral cortex, and striatum (110). This expression may explain some of the behavioral effects of marijuana (motor, memory, or cognitive impairment; see ref. (111)). The CB2 receptor was once thought to be only expressed in peripheral immune cells but has recently been identified in the brain at low levels (112).
FIGURE 3-6 Cannabinoid receptor expression in the rat brain. Areas with brightest colors indicate a greater expression. Cannabinoid receptors are expressed in high numbers in the cerebellum (Cer), hippocampus (Hipp), globus pallidus (GP), external globus pallidus (Ep), and the substantia nigra pars reticulate (SNr). Spinal cord, Sp Cd. (Courtesy of Dr. Allyn Howlett.)
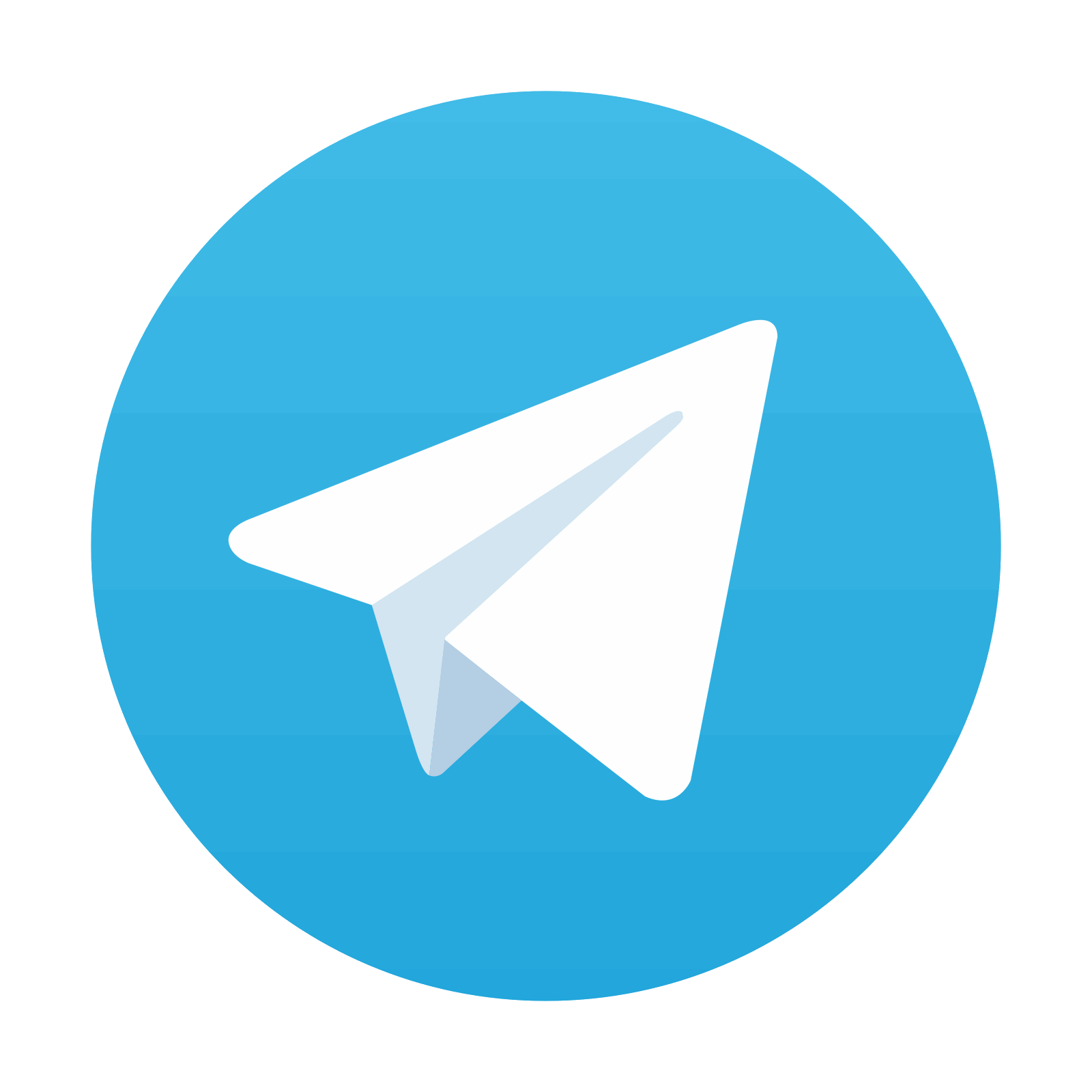
Stay updated, free articles. Join our Telegram channel

Full access? Get Clinical Tree
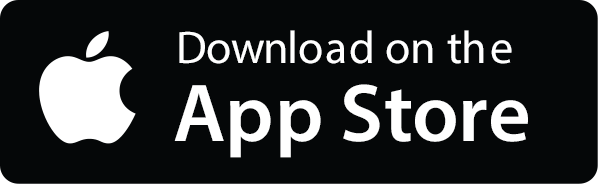
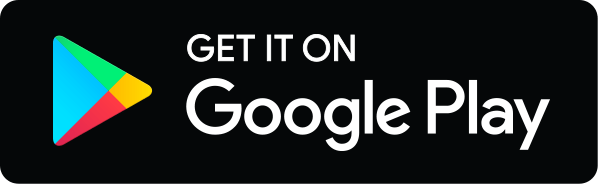