It is evident that a distinct advantage of broth methods is that they permit the determination of a minimal bactericidal concentration (MBC) end point. For those laboratories involved in special clinical pharmacologic studies, the assessment of the clinical efficacy of antimicrobial compounds (older or newly formulated); detailed assays of drug levels, distribution, and toxicity; and fully quantifiable results determined by MIC end points derived from broth dilution studies are required. The MIC can in turn be used to calculate therapeutic ratios, that is, the ratio of serum (or tissue) fluid concentration to MIC. The MIC can be determined from standard quantitative broth (or agar) dilution methods and derived from regression analysis of the diameter of zone inhibition (12,13) (Table 3.1). If the laboratory has established a database and has experience with one methodology, changing procedures for an alternative method can create new problems.
The advantages of one approach over another are summarized in Table 3.1. In certain clinical therapeutic situations (e.g., in cases of endocarditis or osteomyelitis), MICs may be indicated. When MICs are obtained, they can be used to calculate therapeutic regimens to minimize the adverse effects of potentially toxic drugs, giving added confidence in the selected therapeutic regimen. If broth is used, the simultaneous or sequential determination of MIC and MBC values can be achieved or the killing rate determined (14). Furthermore, the quantitative assay of drug combinations to detect synergy is more readily achievable in liquid systems and may be required in certain clinical situations (e.g., in cases of infection with Enterococcus).
For clinical laboratories, combining two approaches could prove to be more useful and cost-effective. For example, antimicrobial susceptibility testing of urine samples could be done by agar disk diffusion, and broth dilution could be reserved for isolates recovered from putatively sterile compartments (blood, spinal fluid, synovial fluid, etc.). With this strategy, isolates recovered from nonsterile compartments would not require quantitative studies.
Results obtained from broth dilution susceptibility testing may be less than optimal with certain antimicrobial–microorganism combinations. The bacteria that have produced difficulties in the past decade are Enterococcus, methicillin-resistant Staphylococcus aureus (MRSA), Pseudomonas aeruginosa, Haemophilus influenzae, and Neisseria gonorrhoeae. Antimicrobials that delivered unreliable data included the sulfonamides, trimethoprim, nitrofurantoin, and newer combination drugs as the β-lactam–β-lactamase inhibitors. These problems were attributable to lack of appropriate standardization of inoculum and medium and have been resolved.
Several variables must be controlled to obtain accurate reproducible results. As with any assay system, deviation from standard procedure modifies the results obtained. Table 3.2 outlines some elements of the broth dilution test that have produced variable results. These are discussed in the pages that follow.
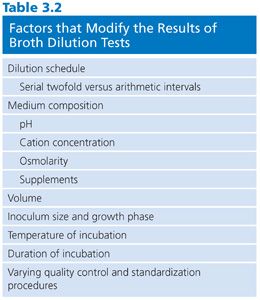
Limitations
One must recognize that the laboratory brings together in an artificial way (in vitro) the bug and drug in a setting outside of the host environment. Whether liquid (broth) or semisolid (agar) environments more truly represent the living human milieu is a subject of ongoing discussion and controversy (15). More pointedly, is the microorganism selected for study representative of the infectious agent offending the host? Because nosocomial infections predominate in hospital settings, a key question is whether the microorganisms recovered from patient specimens are representative of infection or colonization. Usually, this question cannot be answered, but it is clear that the most likely pathogen from a specific specimen should be tested and that routine testing of mixed flora should not be done. The identity of the isolate to be tested frequently dictates or influences the methodology. For certain microorganisms, such as Streptococcus pyogenes and Neisseria meningitidis, testing may not be necessary because the outcome can be readily assured using certain antimicrobial agents or the method and interpretative standard are not yet fully established for the species. For certain rare pathogens or microorganisms with slow growth potential, clearer end points may be obtainable in broth as compared with agar.
In the final analysis, it is necessary to realize that in the laboratory setting, there is a continuous exposure of 105 to 106 colony-forming units (CFU) of microorganism per milliliter to a static (albeit minimally varying) drug concentration during the entire incubation period. These conditions do not prevail in vivo, where larger (or smaller) numbers of bacteria at the infected site are exposed to fluctuating drug concentration gradients.
ANTIMICROBIAL AGENTS
Selection of Antimicrobial Compounds for Evaluation
As the number of approved compounds continues to increase (although now at a limited rate), the challenge for the laboratory is to select rationally a limited number of antimicrobial agents for testing. To test all licensed drugs would be impractical and, in this era of constrained laboratory budgets, uneconomical. In the past, the selection of agents for testing was aided in part by the U.S. Food and Drug Administration (FDA) as it recognized class compounds or, as they apply to agar disk diffusion testing, class disks. However, as the number of chemical classes and congeners within each class increases, the unique pharmacokinetic (PK) properties frequently dictate separate disks for each newly approved compound. With the current proliferation of newer β-lactam agents and quinolones, the class idea of drug selection has become even more important for achieving economy of testing, especially given the availability of the several commercial systems.
Each laboratory and clinical setting should develop a strategy for testing compounds based on previously accrued information on resistance patterns of class agents. Patient demographics as well as patterns of antibiotic use (and abuse) will assist in this selection process. Guidelines to assist laboratories in this process have been proposed by the Clinical and Laboratory Standards Institute (CLSI), formerly named the National Committee for Clinical Laboratory Standards (NCCLS) (10,11) and are included here for convenience (Table 3.3).
It should be noted that the represented groups of organisms are those for which it is most difficult to predict, with any degree of certainty, a susceptibility result based on prior experimental data. The agents in the primary and secondary groups achieve peak levels in serum, whereas those noted in the urine group attain maximum concentrations in that compartment. Final drug selection should represent a consensus opinion at each site or medical center and should include input from the clinical microbiologist, infectious disease specialist, and clinical pharmacist. As noted, these decisions require information about the antimicrobial nature (toxicity and pharmacokinetics) and the probability of resistance for each drug–bug pair previously tested. Acquisition and use costs also need to be considered. Frequently, these data are incorporated into a quarterly or annual summary report distributed by the institution.
Although costs and test constraints may limit the number of agents tested (usually 8 to 12), hospital and/or laboratory information systems can specify the distribution of reports of susceptibility test results to the patient’s chart. In a sense, the problem of selecting agents for testing prompts the following question: What information about which agent should be routinely reported? Compounds that are on restricted or limited formulary status need not be routinely reported, but the data can be stored for consultation and future consideration of routine testing and reporting. The laboratory may also wish to consider testing those agents that are soon to be approved by the FDA, in anticipation of acquiring a database for setting new priorities.
In the performance of any susceptibility assay, the laboratory needs to define its objectives clearly. These should include the following:
1. To test organisms recovered from significant sites (e.g., blood or cerebrospinal fluid) to provide a guide for rational therapy.
2. To evaluate the susceptibility of selected nosocomial agents to determine variations in resistance patterns.
3. To determine and monitor antibiotic susceptibility (resistance) patterns as epidemiologic markers and thus as evidence of the effective use of antibiotics for coverage.
4. To study the activity of recently approved and introduced agents as well as experimental agents.
For routine clinical laboratories, an integrated approach is prudent. This approach serves to discourage the potential abuse of newer agents and thus can help minimize the selective pressure on nosocomial isolates, limiting their potential for developing resistance (Table 3.3).
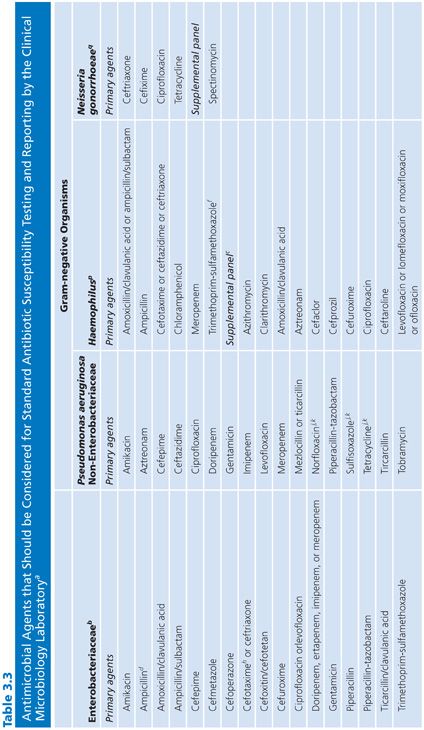
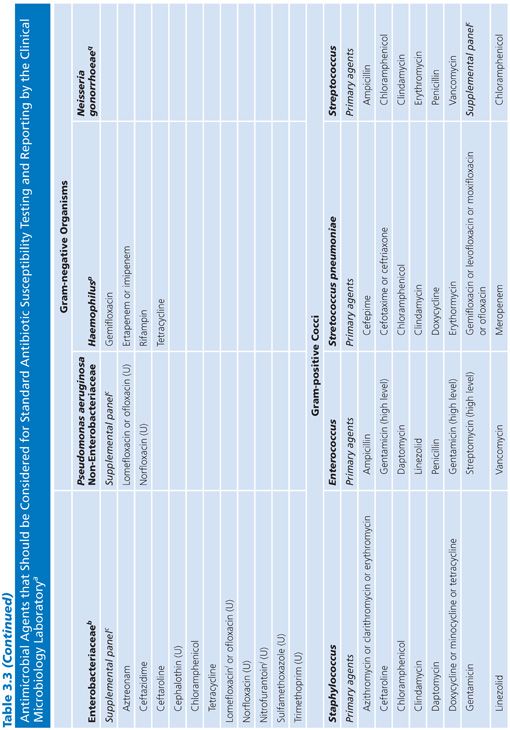
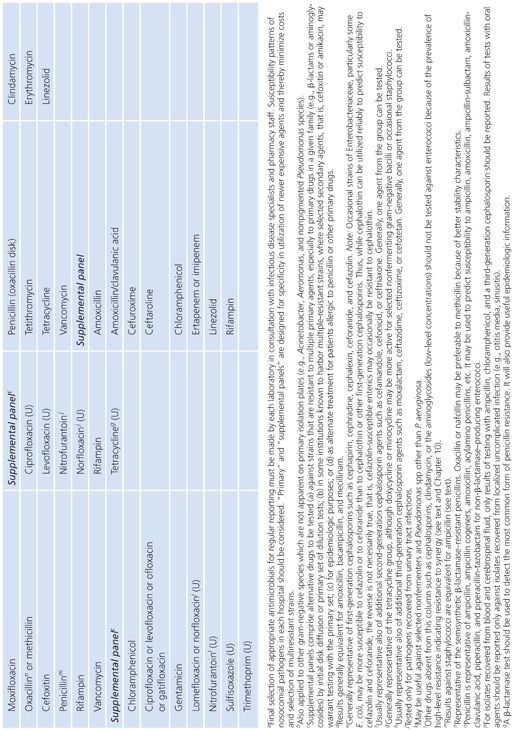
Several caveats need to be mentioned in regard to Table 3.3, which represents a guide for general test selection of antimicrobial agents against selected bacterial genera.
■ For bacteria recovered from cerebrospinal fluid, cefotaxime and ceftriaxone should be tested and reported. Several antimicrobial agents and types may not be effective for treatment. These include the following:
■ Agents administered orally
■ First- and second-generation cephalosporins (except cefuroxime)
■ Clindamycin
■ Macrolides
■ Tetracyclines
■ Fluoroquinolones
■ Rifampin should not be used as the sole antimicrobial agent.
■ The macrolides and clindamycin should not be routinely reported for microorganisms recovered from the urinary tract.
Concentration Range for Testing
When susceptibility plates are prepared in-house or ordered from a commercial supplier, specific concentrations must be selected that conform to the microtiter configuration (conventionally, 8 × 12 wells) or the containers being used. The concentrations selected must satisfy several criteria.
1. The concentrations should extend over the end points of a large series of isolates, taking into consideration whether the distribution of end points is dichotomous, unimodal, or bimodal. For example, Streptococcus pneumoniae and other streptococci (e.g., Streptococcus viridans) are exquisitely susceptible to penicillin. For these organisms, limiting the lower end of the concentration range to 0.5 or 0.1 µg/mL may fail to detect minor but significant variations in the susceptibility pattern. Although such shifts may be minor, they provide a way of tracking emerging resistance. The development of emerging resistance of S. pneumoniae to penicillin has been documented (16,17) but has limited clinical relevance, since the large doses of penicillin that can be administered to patients will kill pneumococci with an MIC 10 times higher than the normal.
2. The concentrations tested should include and exceed (by at least one dilution step) the highest concentration found in biologic fluids. For those compounds that are concentrated in the urine in their active form, such levels can be quite high. PK data for compartmentalized antimicrobics in specific compartments must be reviewed when making these selections. Similarly, for potentially toxic agents, such as the aminoglycosides, it is pointless to test expanded upper ranges of concentrations beyond one or two dilutions above the pharmacologically toxic level. Here is one example of the failure of the twofold serial dilution schedule. Because of the narrow safety margin (therapeutic index) of aminoglycosides, rather than increasing the range above (in this case) the urinary level and taking the drug outside the therapeutic index range, it would be more prudent to prepare small arithmetic incremental dilutions for gentamicin (as well as tobramycin) in the 6 to 12 µg/mL range (18) as follows: 0.5, 1.0, 2.0, 4.0, 6.0, 8.0, 10.0, 12.0, and perhaps 16.0 µg/mL. For amikacin, a similar stepping range from 1.0 to 32 µg/mL is appropriate. Table 3.4 fails to note these figures, because the panels are not commercially available and are difficult to prepare. However, they are presented here for the reader’s consideration.
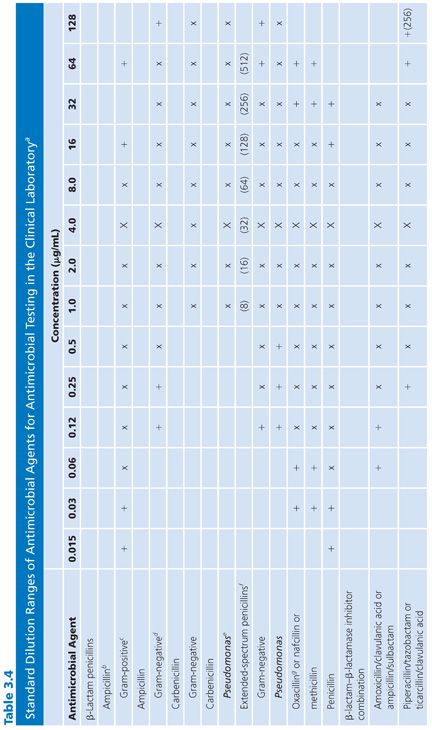
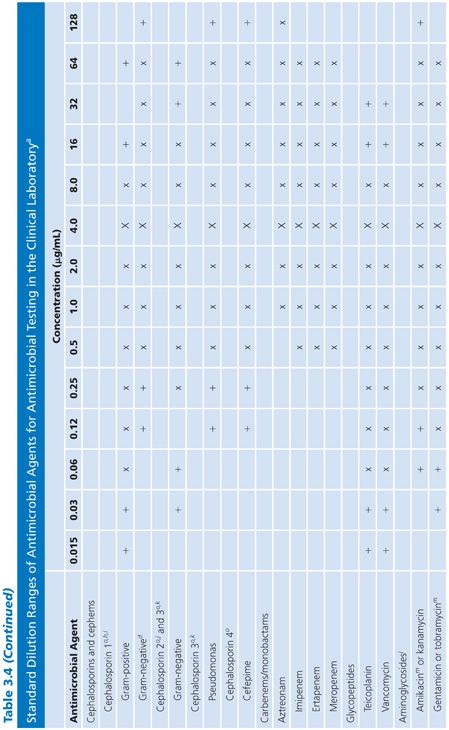
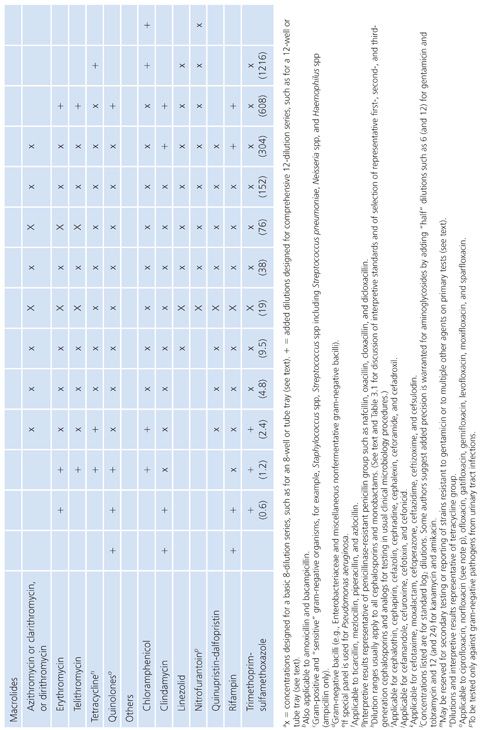
3. The range of concentrations included in the panel should permit the end point detection of quality control (QC) strains (see “Quality Control”). Although routine plates and tubes cannot be prepared in anticipation of predicting synergy-checkerboard patterns, it is worth noting that certain species, such as those in the genus Enterococcus, can be readily treated with a combination of penicillin G or ampicillin and an aminoglycoside, usually gentamicin, to produce synergistic bactericidal activity. Testing for synergistic and bactericidal activity is discussed elsewhere in this volume. Although dilution and synergy or killing curves in broth underscore a conventional basis for quantitative methods of testing synergy, such tests represent a special objective outside the scope of standard broth dilution procedures for routine susceptibility tests. However, the enterococci can be challenged with a single aminoglycoside concentration to determine whether the organisms demonstrate high-level resistance and would be susceptible to the synergistic action of a β-lactam and an aminoglycoside (19). Such high-level resistance can be determined with a single concentration outside of the clinically useful range included in Table 3.4. A 500- to 2,000-µg/mL concentration of aminoglycoside (usually gentamicin) could be included to screen for this type of resistance to synergy in the enterococci, as is common in some commercial systems. It is wise to consider whether this single high-level dilution should be added to the standard series or whether it should be part of a separate screening process for all gram-negative bacilli.
It is difficult to arrange a series of concentrations that satisfy all of the criteria noted earlier and that are applicable in the clinical laboratory setting. However, Table 3.4 presents a suggested outline of concentration ranges designed to approach the goals. Two series ranges are indicated in the table. The first (denoted by “X”) represents a basic set of eight dilutions that cover minimum concentrations. These usually include MIC breakpoints suggested for assigning a general interpretive result to three or four categories, regardless of the terminology used to define the interpretive scheme. The use of widely spaced, selected, screening dilution steps frequently employed for certain drugs in commercial microdilution trays should be avoided. The use of widely spaced (skip-step) dilutions results in a less accurate quantitative assay than does a continuum of concentrations (see “Interpretive Guidelines for Susceptibility or Resistance”) and fails to detect upward trending of resistance. Also noted in Table 3.4 are extended concentrations (denoted by “+”) for expanding the basic dilution series to provide additional relevant concentrations. These additional concentrations are added to either the upper or lower end of the range to permit better estimation of possible clinical utility against partially resistant strains in uncomplicated urinary tract infections, where high levels of drugs may be achieved in the urine (20). The addition of the end dilutions (concentrations) allows detection of modest changes in the susceptibility patterns frequently seen with highly susceptible isolates (as mentioned in the preceding section) for organisms such as S. pneumoniae or N. gonorrhoeae or occasional gram-negative bacilli that might be especially susceptible to newer agents. If the laboratory determines that the scheme outlined in Table 3.4 is unacceptable and represents a far too customized situation, a compromise standard series of dilutions can be prepared, as outlined by the ICS (6). Table 3.5 lists dilution concentrations recommended for preparation of standard twofold dilutions in 13 × 100-mm tubes. The scheme represented in Table 3.5 can be extended to include as many end points as desired. As indicated, 14 dilution steps are included, making the scheme broad enough to address most of the problems noted earlier, but it may not be practical or necessary for the average clinical laboratory. For larger volumes of work, when more tubes are necessary, the scheme outlined in Table 3.6 is recommended (6). Here again, although the series may be customized for specific bacterial agents, employing the same standard dilution for all drugs has the advantage of simplicity and utility for routine use.
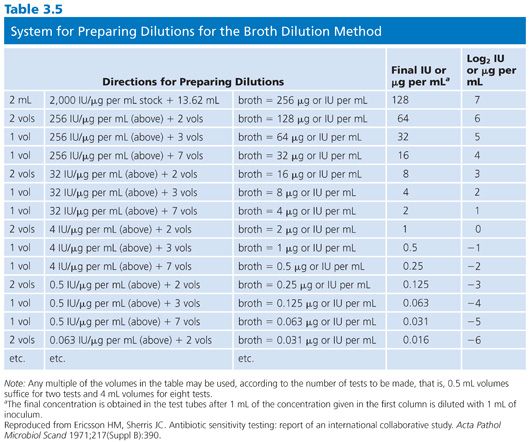
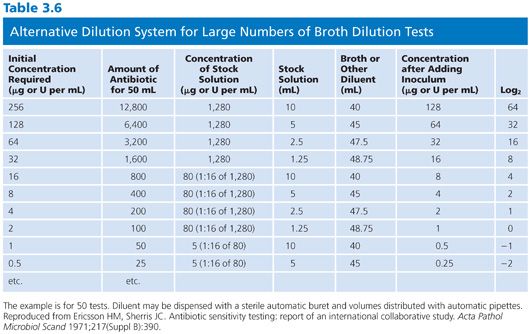
Preparation and Storage of Stock Solutions
The decision to purchase commercial test systems or to prepare in-house panels depends on the laboratory type (clinical, developmental, etc.) and the objectives. Prior to the development of commercial antimicrobial susceptibility test systems, there was little choice, except for deciding whether agar or broth dilution would be used. In either case, rigorous standards have to be followed for controlling the preparation and maintenance of stock solutions of antimicrobial agents.
Reagent-quality antimicrobial powders can be obtained from the pharmaceutical manufacturer, the FDA, or the U.S. Pharmacopeia (Rockville, MD). Drug preparations stocked by pharmacies for clinical administration should not be used in the laboratory because they may contain preservatives and may not be standardized as carefully as assay-quality powders. Additionally, some clinical preparations (e.g., chloramphenicol sodium succinate) require hydrolysis in vivo to be in the active form.
Laboratories need to maintain a detailed registry of the antibiotic powders requested that indicates the date of receipt, supplier, lot number, assay potency, outdate, and storage conditions and incorporates information supplied by the manufacturer. Frequently, along with the powder, the supplier sends a material safety data sheet (MSDS) detailing the method of disposal and other pertinent information on the use of the powder and its potential danger to the user.
Once opened, sealed vials must be stored in a desiccator; some require refrigerated storage in a desiccator. Antimicrobials vary in their storage requirements. Aminoglycosides are stable at room temperature (in a desiccator), whereas β-lactams need to be kept at 20°C or lower. A general rule to heed is that the lower temperatures add to the risk of water condensation and the associated problems of ensuring adequate desiccation. When preparing to open a sealed desiccator unit, it is necessary to hold the unit and its contents at room temperature and permit both to equilibrate. Before being returned to the freezer or refrigerator, the desiccator should be resealed and the accumulated moisture desiccated. Because of their instability, some β-lactams (ampicillin, amoxicillin, and methicillin) should not be stored for longer than 6 weeks. Although there is a paucity of data on the stability of laboratory stock standards, Table 3.7 provides some relevant information (21).
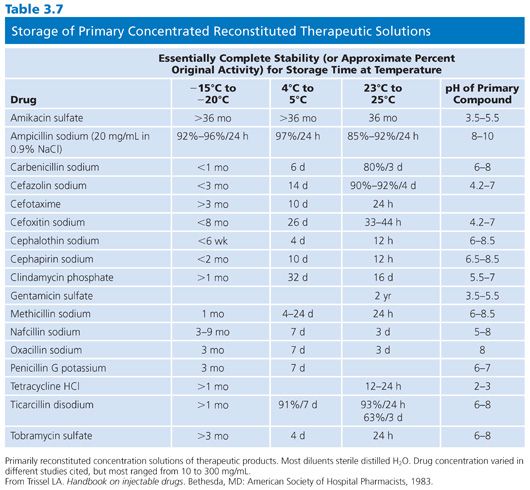
When antibiotic stock solutions are being prepared, powdered (lyophilized) material must be completely dissolved. Because all antimicrobials are not soluble in water, Table 3.8 indicates the nonaqueous solvents that are usually used. Some solvents, such as dimethylformamide (DMF), are intrinsically antibacterial. Barry and Lasner (22) found that use of DMF as a solvent for nitrofurantoin introduced errors in nitrofurantoin diluted 1:50. However, if DMF was diluted in Mueller-Hinton broth (MHB) at 1:100 to 1:200 (i.e., 0.1 to 0.2 mL of DMF), there was no detectable inhibition or antagonism associated with the residual DMF concentration when tested against S. aureus or Escherichia coli (Fig. 3.1).
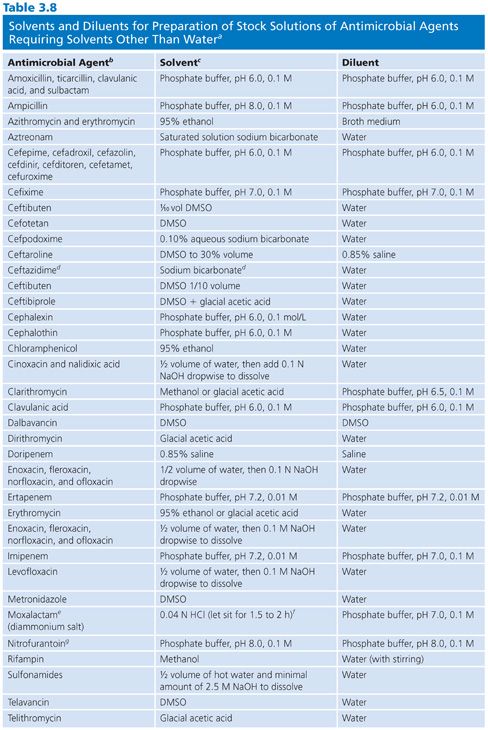
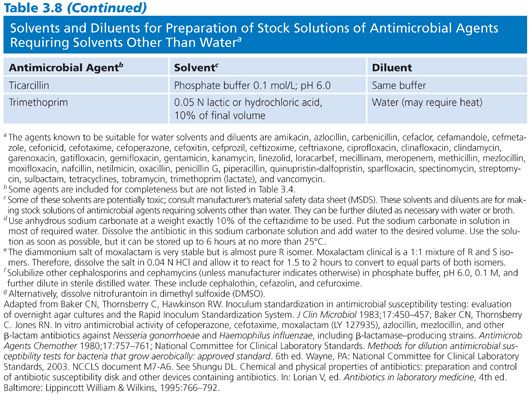
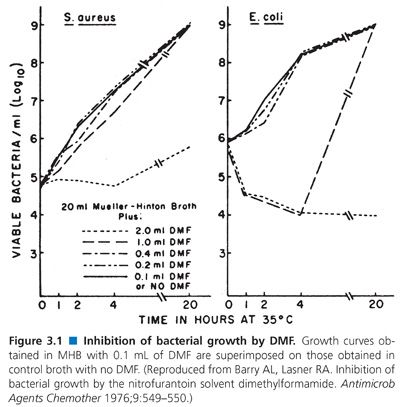
When stock solutions are prepared, they frequently need to be sterilized by filtration. At the level of concentrated stock solutions, it may not be necessary to filter-sterilize because these highly active solutions may be self-sterilizing. Aseptic precautions should be followed when further dilutions are warranted. When filtration through a matrix is needed, care should be taken to avoid the use of fiber pads owing to their absorbent nature, especially with antimicrobial agents of protein structure. The characteristics of four filter types in the retention of antimicrobial activity were studied by Murray and Niles (23) and are listed in Table 3.9.
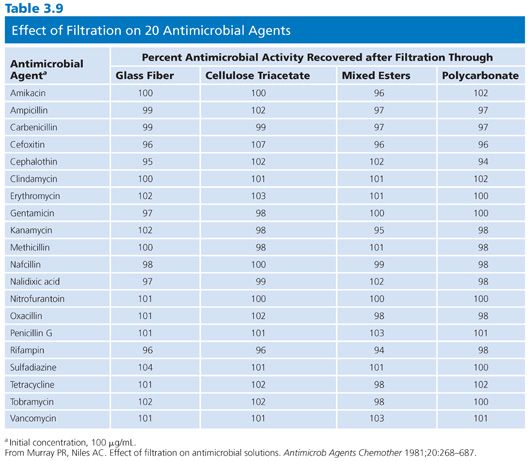
FACTORS THAT MODIFY THE RESULTS AND REPRODUCIBILITY OF THE BROTH DILUTION TEST
Several factors influence the outcome and reproducibility of broth susceptibility results. First, the microorganism manifests its own genetic background, structure, and metabolic behavior, which strongly influence the development of resistance and disease-producing potential. Second, the antimicrobial agent possesses characteristics that affect solubility, protein binding, distribution, absorption, stability, and metabolic modification. The third factor is the milieu in which these interactions are tested and evaluated. These variables are noted in Table 3.2 and are discussed in the following pages. The problems associated with dilution schedules are discussed in “Concentration Range for Testing.”
Media
Ideally, the medium in which the microorganism and antimicrobial agent interact should mimic the serum or interstitial fluid of the patient. The problem of medium influencing the results and reproducibility of susceptibility tests has long been recognized. Liquid (and agar) media suffers from variations in the lots produced by the manufacturer, in part because of the variability of raw materials and the intrinsically undefined nature of the formulations. It is clear that no single medium could ever completely satisfy the goals of providing relevant reproducible end points for all antimicrobial agents tested against all potential pathogens. The requirements for an ideal medium (liquid or solid) have been summarized by Barry (24) as follows:
1. The medium should support the growth of a variety of pathogens for which susceptibility tests are required, without the need for special supplements or enrichments.
2. Medium contents should be defined, at least to the point of specific production details for crude components such as peptone and agar.
3. Different batches of the medium prepared by different manufacturers should yield reproducible results.
4. The medium should be free of components that are known to interact with antimicrobial agents that will be tested.
5. The medium should be capable of controlling pH (especially on the acid side) during the growth of common pathogens.
6. The broth and agar versions of the medium should have the same formulation, except for the solidifying agent.
7. The medium should be approximately isotonic for bacteria, and the agar version should be able to accept the addition of blood when required for the growth of fastidious microorganisms.
Over the years, several different media representing compromises from the ideal have been used. In a coordinated effort, the ICS (6) directed a study to determine the effects of variations in medium constituents. When two media, namely Grove and Randall no. 9 medium (25) and Mueller-Hinton medium (26,27), were compared (1), it was found by the ICS that Mueller-Hinton was more suitable for supporting the rapid growth of enteric gram-negative bacilli and group A streptococci, whereas Grove and Randall no. 9 produced better growth of these gram-positive organisms: Staphylococcus aureus, the enterococci, and Streptococcus viridans, the streptococci. When two commercial sources of Mueller-Hinton were evaluated, notable differences were found only for the aminoglycosides (at that time, streptomycin and kanamycin). It is likely that varying cation content accounted for these differences (discussed later).
MHB is probably the most widely recommended liquid medium for broth dilution tests (6,28–32). However, this medium was originally intended not for susceptibility testing but for the isolation of pathogenic Neisseria species (33). Because MHB contained low levels of paraaminobenzoic acid (p-ABA), it was used in agar form to determine the susceptibility of microorganisms to sulfonamide (34). The beef extract and casein hydrolysate in MHB are poor credentials for a reference medium. The former is difficult to standardize, and the high salt content of the latter needs to be compensated for. In spite of these deficiencies, the ICS group decided to use MHB in several studies because of the relatively good reproducibility and simplicity. An added feature was the low content of p-ABA, which made the medium suitable for testing sulfonamides. When MHB, brain-heart infusion (BHI), trypticase soy (TS), and Oxoid media were used in comparing tube (macrodilution) and broth microdilution results, MHB fared extremely well (35). In a replicate test series with S. aureus, none of the means of MICs varied by more than one dilution. For gram-negative organisms, the MICs were generally lower for MHB than for the other media tested. The greatest variation was with the Oxoid medium, which produced differences of as much as three dilutions between the macrodilution and microdilution methods. In another study in which the same four media were evaluated, Tilton et al. (36) found significant differences among the media when tested against reference strains of E. coli (Table 3.10), S. aureus, and Pseudomonas spp. The highest MICs were obtained in TS broth for the gram-negative strains. Differences between the media rarely exceeded 1.5 dilution steps, and the authors concluded that MHB, at cation-adjusted concentrations, was an acceptable medium. Other researchers have reached the same conclusion (37).
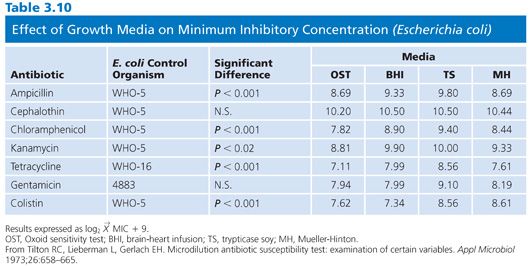
The issue of Mueller-Hinton being designated as a reference medium, even though its deficiencies have been recognized, has become moot. The medium has been used extensively, and there appears to be no proposals or contenders to replace it. CLSI (NCCLS) has established a reference lot for Mueller-Hinton agar (38). Cation-adjusted Mueller-Hinton broth (CAMHB), once recommended by CLSI (NCCLS) for routine testing of commonly encountered microorganisms, is now recommended when testing all species and antimicrobial agents. CAMHB is available from commercial manufacturers with Ca2+ (20 to 25 mg/L) and Mg2+ (10 to 12.5 mg/L) supplemented for convenience and uniformity. It is necessary to use CAMHB when aminoglycosides are tested against P. aeruginosa and when tetracycline is tested against other bacteria.
pH, Buffer, and Incubation
The mechanisms of the effect of antimicrobial pH are not precisely understood and are not consistent from drug to drug. In addition, the pH of the medium affects the activity of certain antibiotics. For some drugs, the pH variation is minor. For example, the nonionized side chain of penicillin G is slightly more active in acidic medium, but the effect is inconsequential, in that it can be demonstrated only by utilizing special experiments. The effect of the pH of the medium on the activity of six classes of antimicrobial agents is indicated in Table 3.11.
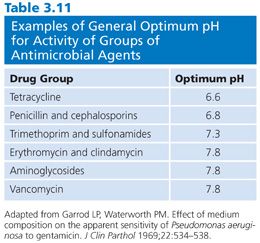
Penicillins that are nonionized or weak acids demonstrated minor variations according to the broth medium used, even when the pH was adjusted to approximately 7.0, and the MICs observed were generally within experimental error (one or two dilutions) for five different broths (39). This situation is in contrast to that of the aminoglycosides. Streptomycin is 500 times more active in alkaline medium than in acidic medium (40). Other aminoglycosides show similar but less drastic shifts in activity with changes in pH (Table 3.12).
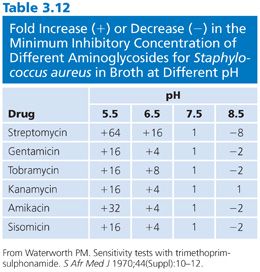
The buffering capacity inherent in the formulation of a medium contributes to the stability of the pH during incubation; the inclusion of glucose in the medium results in some lowering of pH during the growth of strains that can ferment the substrate. These differences were observed in medium with and without glucose when E. coli was used as the test organism (24). The effect of adding specific buffers to stabilize a defined synthetic amino acid medium (SAAM) (41) has also been demonstrated.
Does the pH of the medium modulate the outcome of susceptibility results to the extent that the interpretation of the test and its clinical relevance would be altered? Can the pH environment of the patient be clinically manipulated significantly for therapeutic purposes? The answer to both questions is in the affirmative. Such examples probably occur more readily with the macrolide and aminoglycoside groups, which are more active in slightly basic media, and the tetracyclines, which are more active in acidic environments. Definitive changes in interpretive results have occurred with gentamicin, kanamycin, erythromycin, novobiocin, and tetracycline (15) (Table 3.13).
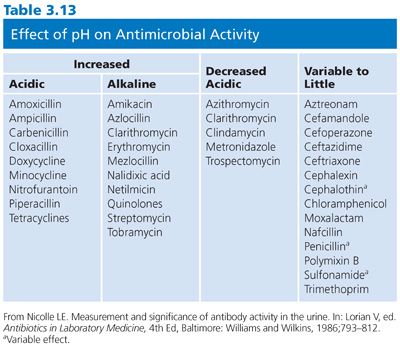
The marked pH effect on the aminoglycosides may be explained by the degree of ionization of these compounds. However, this explanation is not plausible for changes with the prototypic penicillin G, which is intrinsically slightly acidic but allows for minimal changes of antibacterial activity in the range pH 6 to 8. Another mechanism proposed for nonionized erythromycin may be directly responsible for antimicrobial activity (42). The antimicrobial activity of this macrolide is enhanced vividly as the pH approaches a pKa of 8.6 (Fig. 3.2). This enhanced activity of erythromycin at alkaline pH extends the spectrum of activity of this compound to include gram-negative organisms, particularly E. coli (43). These observations have been used in the clinical treatment of urinary tract infections. It has been shown that alkalinization of the urine is sufficient to activate erythromycin and produces successful clinical treatment (42). Theoretically, laboratories should set up an environment that mimics this pH difference, but rarely is this needed or accomplished.
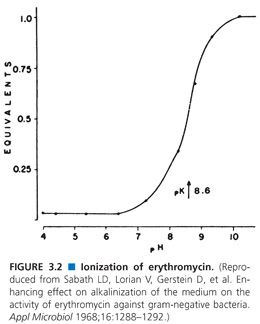
Another example of the effect of pH on the outcome of susceptibility test results and treatment can be found in studies with Helicobacter species. During the past several years, Helicobacter organisms have been implicated in a wide variety of conditions involving the stomach and duodenum, from gastritis to dyspepsia, as well as potentially gastric carcinoma (44). Helicobacter pylori was formerly referred to as Campylobacter pylori and is generally regarded as the agent associated with or implicated in these conditions. H. pylori is susceptible to a wide variety of antimicrobial agents, including penicillins, cephalosporins, macrolides, nitrofurantoins, and quinolones (44,45). The relative efficacy of these treatments is unknown, and many patients have gastric intolerance as a side effect (44). It is uncertain at which pH antimicrobial agents demonstrate maximum effectiveness in vivo. Similarly, it is questionable at what pH antimicrobial susceptibility testing should be executed. Recently, Grayson et al. (46) tested the susceptibility of 22 isolates of H. pylori (obtained from gastric mucosal biopsies) against eight antimicrobial agents. Antimicrobial susceptibility studies utilized the agar dilution methodology with an inoculum of 5 × 105 CFU/spot. The comparative efficacies of the agents are shown in Table 3.14. The macrolides, quinolones, and clindamycin demonstrated diminished activity at acidic pH, compared with their activity at pH 7.4. In the alkaline pH range, MIC90 values were moderately but uniformly decreased (increased potency) for these same drugs. The MICs for ampicillin and metronidazole were essentially constant and within clinically achievable levels in the three pH ranges tested. The authors concluded, based on the results of their study, that if the pH of a human gastric environment was only slightly below pH 7.4, a significant loss of antimicrobial activity could result. They suggested that the modulating effect of pH on antimicrobial activity may explain the apparent discrepancy between in vitro susceptibility results and observed therapeutic failures and that clinicians need to consider the effect of pH when selecting an agent for the treatment of H. pylori. Added data from a study of 30 clinical isolates of H. pylori with trospectomycin, ampicillin, metronidazole, clarithromycin, azithromycin, and clindamycin under varying pH conditions showed that an acidic environment unfavorably affected the activity of all of the agents tested (47).
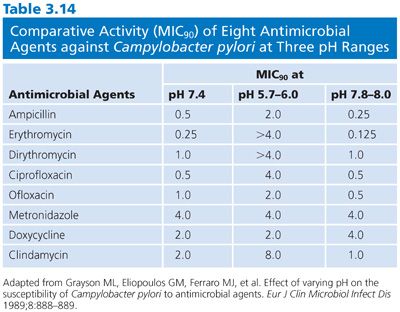
The gaseous environment is important in stabilizing the pH of media, particularly solid media; it can also affect broth. The presence of carbon dioxide (CO2) in the incubation atmosphere, for example, may produce acidic changes, at least early in the course of incubation. Standardized susceptibility testing should not be performed in a CO2 environment. If capnophilic organisms are being tested, their growth would be scanty in an aerobic atmosphere, and so they can be incubated in CO2, but interpretation of the results is not considered routine. When susceptibility results obtained in an aerobic environment and a 10% CO2 incubator were compared, the MICs of aminoglycosides and erythromycins were higher (i.e., the antimicrobials were less active) and tetracyclines, methicillin, and novobiocin were more active in the CO2 environment (6). These studies pertained to diffusion tests; however, the net effect of the 10% CO2 environment was a lowering of pH.
Although one would expect that the target temperature for incubation of antimicrobial susceptibility tests would be between 35°C and 37°C, the temperature of incubation has been studied, particularly in regard to the detection of MRSA. Because MRSA strains are known to grow at reduced rates, it would be anticipated that these would be favored at lower incubation temperatures, compared with their more rapidly growing susceptible counterparts. In a study by Mackowiak (48), incubation at 35°C proved satisfactory for routine growth of MRSA strains as well as for all susceptibility tests. However, the heteroresistance of MRSA may be missed at 37°C. At elevated temperatures (from 35°C to 41.5°C), incubation of routine susceptibility tests (microtiter and serum bactericidal tests) indicated that more than 20% of the MIC values were lowered significantly (Fig. 3.3), and serum activity was enhanced at the higher temperatures.
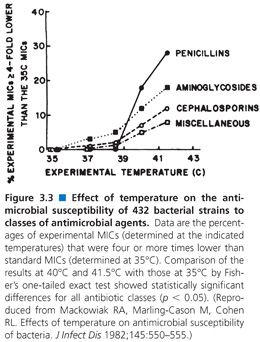
The molarity (i.e., the strength) of a buffer used in the formulation of a medium can also affect the antimicrobial activity of certain compounds. In tobramycin assay systems, it was shown that the activity of the drug was generally increased with decreasing molarity, and the effect was dependent on the type of assay system used (49). For gentamicin, increasing concentrations of phosphate buffer in concert with shifts in pH produced a greater degree of error.
The extent of incubation has been studied in relationship to its effect on MIC. As incubation was prolonged, increasing MICs of cephalothin were observed beyond 12 hours (50). Such effects may be partly due to progressive antibiotic inactivation with increasing incubation interval and are more likely to be encountered with the relatively unstable penicillins and cephalosporins (51,52). Broth and agar dilution methods were compared (53) in relationship to the duration of incubation (12 and 24 hours) (Table 3.15).
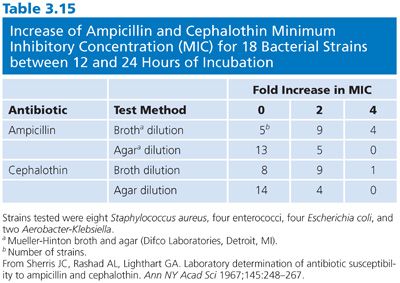
For the broth dilution techniques, the MICs increased at least twofold more than twice as often with prolonged incubation, compared with the changes that occurred between 12 and 24 with agar dilutions. This effect can be observed for ampicillin and cephalothin as well as newer broad-spectrum β-lactams. In broth dilution systems, the best reproducibility and minimal alteration in MICs from the late growth of residual persister cells can be seen if a routine is followed of reading broth dilution end points after overnight incubation (usually 18 hours). (See “Automated, Rapid, and Instrument-Associated Methods” for the adverse effects of short-term incubation.)
When microorganisms are exposed in broth to antibiotic concentrations for short durations and then the antibiotic is removed or inactivated, the resultant effect has been termed the postantibiotic effect, defined by Craig and Gudmundsson (54). This phenomenon may be relevant to clinical responses to therapy and can be used to establish dosage schedules (55,56). Extended incubation over a number of hours in antibiotic-deficient medium must be used to determine the residual effect of brief exposure (0.5 to 4 hours) to the antimicrobial agent, so this type of assay cannot be considered a routine or rapid test.
Cation Concentration and Osmolality
The divalent cations calcium and magnesium have a profound modulating impact on the effects of the aminoglycosides, especially gentamicin. For example, the general results of broth dilution tests with gentamicin show MICs 1 log2 unit higher than the results for agar medium, probably reflecting variations in cation concentrations. Other aminoglycosides, as well as tetracycline, are also affected. Varying cation content has posed significant problems in agar medium (57). However, different concentrations of magnesium in broth medium have also been responsible for marked MIC variations in the activity of gentamicin against many Pseudomonas strains (58). Falsely low MICs are observed with media not supplemented with calcium and magnesium. A similar but less pronounced effect can be seen against other gram-negative organisms in unsupplemented media (58,59). The data in Table 3.16 indicate results obtained by varying the concentrations of magnesium, calcium, and sodium salts in nutrient broth for susceptibility studies of Pseudomonas with gentamicin. After extensive surveys (60), it was suggested that final concentrations of magnesium of 20 to 35 mg/L and concentrations of calcium of 50 to 100 mg/L should supplement broth. These concentrations modify the medium and bring it into the physiologic concentration range found in serum (Table 3.17). In commercial microdilution systems, the medium is cation-supplemented.
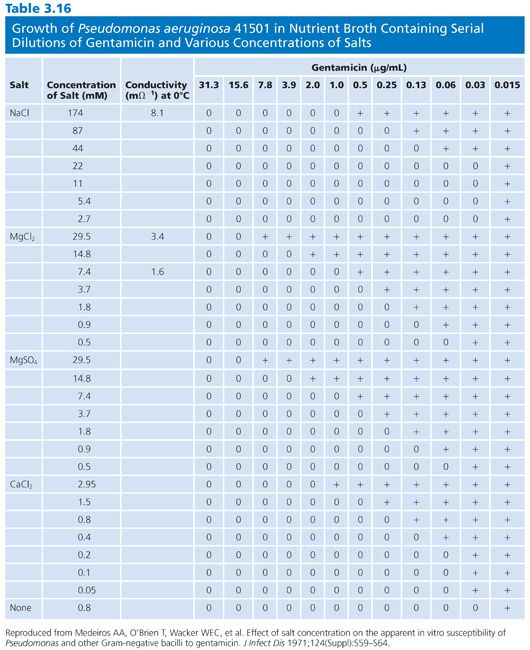
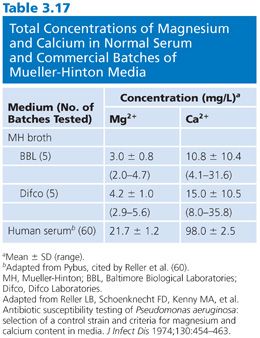
In studies with the tetracyclines, different MICs were obtained in TS broth compared with MHB (62). These differences were attributed to variations in cation concentrations. MHB produced eightfold lower MICs against S. aureus, E. coli, and Klebsiella species, but the antibiotic inhibitory effect of the TS broth was reversed by the apparent chelating effects of phosphate, oxalate, or citrate. The opposite effects (increased MICs) on tetracycline in MHB were produced by adding the cations magnesium, calcium, or iron (62).
Sodium chloride (NaCl) concentrations change the osmolarity of medium and have a marked effect on the activity of the aminoglycosides gentamicin (63) and tobramycin (49). A sixfold decrease in activity and increase in MIC was noted by changing (increasing) the NaCl concentration from 22 to 174 mmol/L.
β-Lactams that bind to varying penicillin-binding proteins (PBPs) may be uniquely affected by the osmolarity and, in turn, the conductivity of the test medium. This effect of osmolarity varies from strain to strain of bacterium. For some strains of Proteus or Klebsiella tested against mecillinam, a 500-fold increase in MIC as a result of increasing molarity from 185 to 402 mOsmol/L was observed. In contrast, a strain of Enterobacter cloacae showed no change in the MIC for mecillinam between the same osmolarity levels in the same broth (NIH medium) (64).
Supplements and Other Additives
Recently, MHB has been shown to be acceptable for a wide variety of antimicrobial susceptibility tests and to be well suited for standard, rapidly growing pathogens such as enteric gram-negative bacilli, Pseudomonas spp, staphylococci, and Enterococcus spp. For organisms that grow rapidly and have been studied adequately, there are well-standardized interpretive guidelines and QC standards (10,11). For bacteria that do not grow readily on this medium, other supplements or alternative media may be required. The addition of blood or hemoglobin or of special additives such as IsoVitaleX (Baltimore Biological Laboratories, Baltimore, MD) has been proposed to enhance the growth of many fastidious organisms that may frequently require susceptibility testing (see “Fastidious and Unusual Pathogens”). Because some of the supplements produce an opacity that cannot be readily used in standard macrodilution or microdilution broth systems, they are more suitable to solid agar medium. The susceptibility testing of H. influenzae has long been a problem in this regard. Only recently, Jorgensen et al. (65) studied the problem and developed a special test medium, Haemophilus test medium (HTM), which has become commercially available and provides a solution to this problem.
The addition of various supplements of unknown chemical composition may in some instances alter antibiotic activity. However, if adequate controls are incorporated, standards are developed for appropriate interpretation, and additional control strains are utilized in the testing protocol, media supplemented with various components may be used successfully for broth dilution susceptibility tests.
Supplementation of test media with previously used (but undefined) components can result in unusual effects. Eliopoulos et al. (66) reported on the effect of 5% sheep blood added to Mueller-Hinton agar on the activity of cefotaxime and other cephalosporins against Enterococcus faecalis. They determined that the activities of cefotaxime and other aminothiazoyl oxime cephalosporins (e.g., cefpirome, ceftazidime, cefmenoxime, ceftriaxone, and ceftizoxime) against E. faecalis were enhanced by the addition of 5% sheep blood. This effect was not documented for aztreonam (a nonoxime aminothiazoyl), cefotiam, or other cephalosporins and was specific to the syn-configuration of the oxime moiety. Enhancement of cefotaxime activity was shown against 50% of 85 clinical isolates and could be demonstrated only with low bacterial inocula. The α-globulin fraction of serum mimicked this enhancing activity, whereas α1-, β-, and γ-globulin fractions and albumin frequently antagonized or did not significantly affect the antimicrobial activity. Although these observations may not have direct clinical relevance, they present a possible explanation for the relatively infrequent occurrence of enterococcal superinfection in patients treated with cefotaxime, which demonstrates poor in vitro activity.
When 5% sheep blood or 10% fetal calf serum was added to liquid media for testing H. pylori susceptibility (67), sheep blood inhibited growth. When the effect of bismuth was evaluated, it was found that the compound inhibited growth in medium containing starch but that the inhibition was neutralized in medium containing serum (Fig. 3.4).
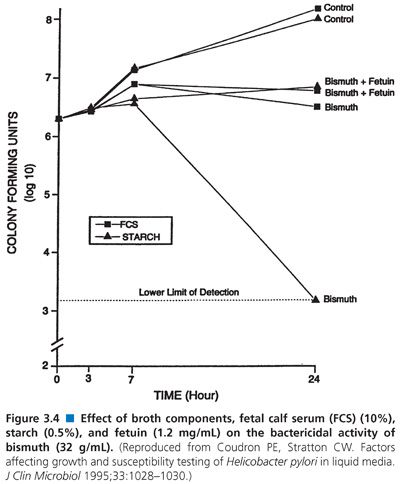
Prior to the development of HTM, broth dilution tests were satisfactorily done using MHB (68). Today, susceptibility testing of sulfonamides does not pose a serious, relevant clinical problem. Sulfonamides are rarely used in treatment, and their testing is not usually required. Media enriched with various digests or supplements may contain p-ABA, thus obviating sulfonamide inhibition and rendering the tests inaccurate or useless. Testing of trimethoprim or a combination of trimethoprim and sulfamethoxazole presents similar problems, in that the activity of trimethoprim or sulfamethoxazole is antagonized by the high thymidine content of enriched media. This problem exists in vitro although apparently not in vivo, because thymidine and thymine both seem to be present in sufficiently low levels in blood and urine so as not to interfere with in vivo bacteriostatic and bactericidal activity during treatment (69,70). Thus, media have to be adjusted so that the thymidine content is diminished or absent. A suitable formulation was designated SF medium base (71). Dramatic differences between a standard broth medium and one enriched with 5% lysed horse blood can be seen in Figure 3.5. The supplement of 5% lysed horse blood contains sufficient thymidine phosphorylase to inactivate thymidine in various media (71,72). In the United States, most commercial lots of Mueller-Hinton medium have proven satisfactory for overnight incubation as a result of careful QC measures by the manufacturers. Other technical problems encountered with broth dilution methods when testing sulfonamides and trimethoprim result in hazy end points. These problems have been resolved by manipulation of the medium and the use of small inocula (72).
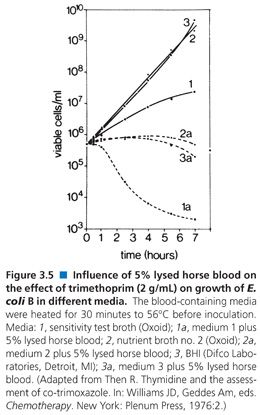
Other Media
It appears that MHB and CAMHB have established a foothold in the antimicrobial susceptibility literature. Presently, no other medium has been studied sufficiently to replace them. There have been investigations into one defined medium, namely, SAAM (41). Although this medium proved comparable in growth support and antagonism of sulfatrimethoprim, it has not gained acceptance for routine use. Similarly, another defined medium free of purines or pyrimidines (73) has been studied. However, a large proportion of Streptococcus and Staphylococcus strains failed to grow on this medium; thus, it is unsuitable for use in routine clinical laboratories. There has been little investigation into other media that would meet the ideal criteria defined by Barry (88,89). It is more likely that, with the aid of organizations like CLSI (NCCLS), reference lot media may be designed and accepted. As noted, a reference lot has been defined for Mueller-Hinton agar with regard to susceptibility testing of H. influenzae on agar surfaces.
INOCULUM SIZE, EFFECTS, AND STANDARDIZATION
The density of inoculum in an antimicrobial susceptibility assay is critical for the generation of reliable and reproducible susceptibility test results. The adjustment of inoculum density is more important for broth dilution tests than for disk diffusion and agar dilution methods. The reason is that, with agar methods, visual (macroscopic) inspection of growth permits semiquantitative evaluation of inoculum density. In contrast, it is difficult to estimate the growth density in the growth control tubes (Table 3.1).
Susceptibility test results differ according to the species, strain, and antimicrobial agent tested (36,53,74,75). During growth of a particular bacterial strain, it can be assumed that homogeneous progeny develop. However, some degree of inoculum size effect may result from the MICs of individual bacterial cells that follow a normal distribution. Thus, with larger inocula, there is a greater probability that there will be some cells or variants from the more resistant end of the distribution curve. In broth, the cells from this extreme of the normal distribution are more likely to survive and grow.
As mentioned, large bacterial populations are less promptly and completely inhibited than smaller ones. Also, the likelihood of the emergence of resistant mutants is greater in a large population of bacterial cells. This is exemplified by the emergence and recognition of MRSA and methicillin-resistant Staphylococcus epidermidis (76). To detect and define these particular populations more accurately, it is necessary to depress the growth of the more rapidly growing, susceptible population and to extend the incubation interval beyond the usual 18 to 24 hours so as to readily detect the presence of the more slowly growing, resistant population (77). There is less chance of observing the resistant population if the critical inoculum is small. According to Sanders et al. (78–80), low-frequency mutant subpopulations of antimicrobial-resistant organisms are best detected by broth dilution systems at an inoculum concentration of more than 105 CFU/mL.
The inoculum effect, first reported in 1940 for the interaction of Streptococcus haemolyticus and a sulfa compound (81), was later described for penicillin and S. aureus (273). The effect has been found in several bacterial species and is particularly widespread among the β-lactam antimicrobial agents when their activity is directed against β-lactamase–producing bacteria. Although the inoculum effect has been most widely studied in the staphylococci, it has been shown to be associated with a variety of bacterial species and almost every class of antimicrobial agent. Table 3.18 outlines the antibiotic–organism pairs that generally exhibit an inoculum effect. This effect is generally attributed to the inactivation of the antimicrobial agent by β-lactamase (82). However, it is known to occur with antimicrobial agents lacking the β-lactam ring. Other possible causes of the inoculum effect include the selection of resistant mutants and drug breakdown by other drug-targeted inactivating enzymes. The inoculum effect can be defined as a significant increase in MIC (plus two dilutions) when the inoculum size is increased (at least by 0.5 log unit). In some studies, the effect was noted when the inoculum was varied by four orders of magnitude. Early studies investigating this phenomenon need to be considered in light of the standardized elements of testing recommended by CLSI (NCCLS) (10). Additionally, studies elucidating the inoculum phenomenon have not been well standardized; they vary in incubation interval, reagent volume, and size of the test vessel.
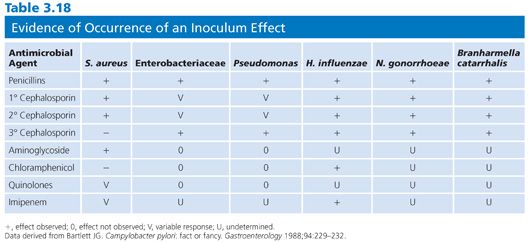
The clinical implications of the inoculum effect are uncertain. Clearly, the inoculum standard established by CLSI (NCCLS) (5 × 105 CFU/mL final concentration for broth dilution and 104 CFU/spot) is not applicable to all clinical situations. At best, it represents a compromise between various clinical infections and the procedural manipulation for eliminating the potential for trailing end points. This methodologic result occurs when the inoculum exceeds a final concentration of 107 CFU/mL. With several of the antibiotic–organism pairs that have exhibited the inoculum effect (Table 3.18), the final MIC result, although elevated compared with the result obtained with a smaller inoculum size, would still have yielded an interpretation of susceptible based on the MIC:level ratio.
Of the several factors that can modulate the outcome of antimicrobial susceptibility tests, the inoculum size, besides being relatively easy to measure, should be among the easiest to standardize. The role of inoculum size was shown in studies of penicillin-sensitive and penicillin-resistant strains of S. aureus treated with cephalexin (83,84). Marked changes in MICs and MBCs were observed for 100-fold changes in inoculum density (Fig. 3.6). The effects of 10-fold dilutions of inoculum, from 103 to 107 CFU/mL, can be seen for several antibiotic groups used against E. coli (Table 3.19), gram-positive and gram-negative organisms (Table 3.20), and Pseudomonas spp (Tables 3.21 and 3.22).Against E. coli, tetracycline was found to be the most refractory to inoculum effects, whereas significant changes were recorded with the other antimicrobial agents, especially at the high end of the inoculum range (Table 3.10). For P. aeruginosa, the effect of varying inoculum size on the activity of the antimicrobials tested was related to the agent tested (75). When the inoculum was increased to 5 × 105 CFU/mL, the MIC90 values for all drugs tested were increased (Table 3.21).When the inoculum was increased further to 5 × 107 CFU/mL, the MIC90 values could be determined only for gentamicin and thienamycin. The MBC90 values at an inoculum of 5 × 105 CFU/mL ranged from 8 µg/mL for gentamicin and thienamycin to 128 µg/mL for cefotaxime (Table 3.21). With the largest inoculum, the MBC90 values for gentamicin and thienamycin remained constant but the MBC90 values for the other drugs tested were less than 128 µg/mL. The susceptibility results for H. influenzae are seriously influenced by the size of the inoculum (85–87). The effect is more pronounced for ampicillin-resistant isolates (β-lactamase producers?) and penicillin than for cephalosporins. Now that inoculum size and medium have been defined for H. influenzae susceptibility testing, variation in susceptibility test results is anticipated to diminish.
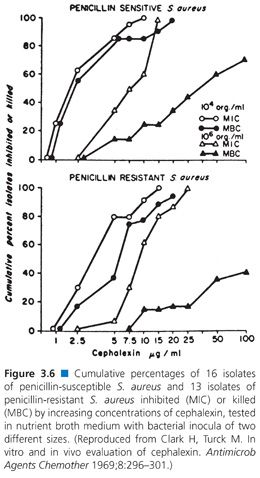
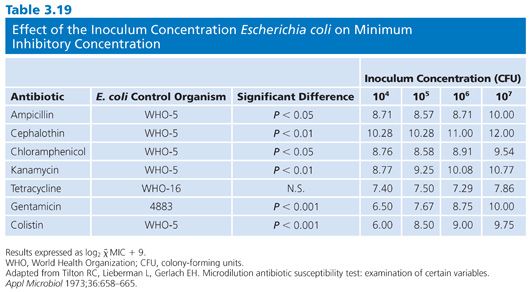
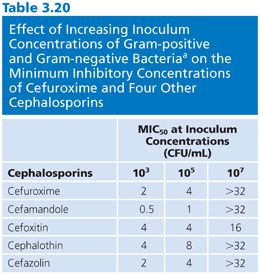
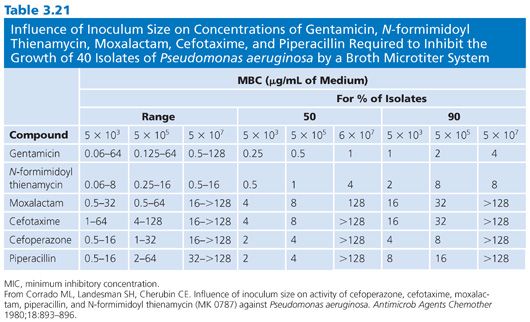
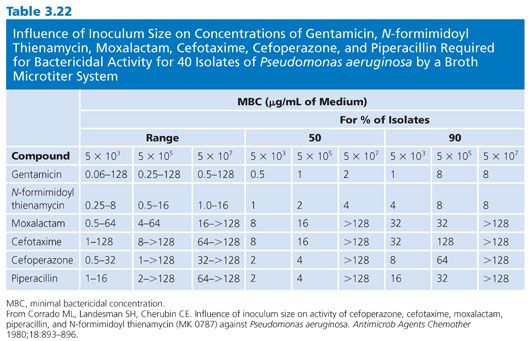
The effect of inoculum density variation on various antimicrobial agents tested against several species must be considered in any recommendation for a standard or reference method. Most investigations support the earlier work of the ICS (6), which suggested that an inoculum of 105 to 106 CFU/mL would yield acceptable results in a macrobroth dilution test. Some workers adjust the inoculum closer to the range of 1 to 5 × 105 CFU/mL. For microdilution, the average recommended inoculum is 1 × 106 CFU (30).
Methodology for Standardizing Inocula
For the reasons mentioned earlier, it is imperative that each culture inoculum be individually standardized. The procedures frequently used for both the macrodilution and microdilution systems involve either adjustment of a logarithmic-phase broth culture to a McFarland 0.5 turbidity standard (7,10) or defined direct dilutions from 0.5-mL volumes of stationary-phase broth cultures (10). Several discrete colonies, usually three to seven, are subcultured to the inoculum growth broth, to avoid single-colony variance. The inoculum is usually cultured in the same broth medium used for the test, such as MHB or CAMHB (richer media such as TS broth and BHI can also be used satisfactorily). For rapidly growing pathogens, overnight broth cultures (4 to 8 mL) grow to approximately 109 CFU/mL. A 1:1,000 or 1:2,000 dilution of this growth brings the inoculum density to the range of 5 × 105 to 5 × 106 CFU/mL. Results have generally been satisfactory with such methods, because most rapidly growing pathogens achieve stationary-phase growth at density levels that fall within a reasonable range. Alternatively, one can adjust the turbidity of a 4- to 8-mL overnight culture or a 4- to 6-hour broth culture (both are considered to be in the stationary phase) to a standardized density. This can be accomplished nephelometrically with instruments frequently provided with automated units for determining susceptibility or manually by dilution to match the visual turbidity of the McFarland 0.5 BaSO4 standard, which approximates 105 CFU/mL. The 4- to 6-hour broth culture offers a significant time advantage compared with the overnight incubation. However, for some more slowly growing, more fastidious strains, such as Haemophilus or Neisseria strains, overnight growth may be required. Satisfactory results have been obtained by emulsifying colony plate growth to match either nephelometric or McFarland turbidity standards. Then, appropriate dilutions (e.g., 1:2,000) are made for the final inoculum.
It has been shown that turbidity-adjusted direct suspensions can also yield reproducible and accurate results for commonly encountered gram-negative or gram-positive, rapidly growing microorganisms (44). When the growth phase of the inoculum was studied systematically by Barry et al. (88), they found that generally satisfactory reproducibility with control strains was dependent on whether the inoculum standard utilized required harvesting direct suspensions from overnight colonies or from logarithmic-phase (2- to 4-hour) or stationary-phase (5- to 6-hour) broth cultures.
After an inoculum has been prepared, variability has been observed in the delivery of the inoculum to the test system. In some systems, this was more dependent on a mechanical inoculum preparation and transfer system and independent of the growth phase of the organism. Inocula prepared with Pseudomonas strains demonstrated lower counts, perhaps due to clumping, than did those prepared with other organisms (89). Final MIC results were generally reproducible and consistent regardless of the method of inoculum preparation and despite the system-dependent inoculum variations from 2 × 104 to 1 × 106 CFU/mL (44). Table 3.19 indicates that, with some drug–organism combinations (10%), statistically significant differences in geometric mean MICs resulted from different growth or inoculum preparation methods tested in two different microdilution systems (89).
Numerous techniques have been developed for inoculum preparation, standardization, and transfer and been incorporated into the expanding range of commercial microdilution test systems (see “Standard Broth Dilution Procedures”). Several systems adjust turbidity suspensions instrumentally. However, most recommend the use of a mechanical device that involves touching five colonies directly with a wand containing grooves at its bottom crosshatch, followed by emulsification in an appropriate volume of saline. This system, referred to as RISS and marketed as PROMPT, eliminates prior broth incubation and turbidity adjustment (90,91). Figure 3.7 illustrates how the CFU/mL values in the inoculum are affected by increasing the number of colonies touched; a maximum colony count is achieved after selecting four colonies.
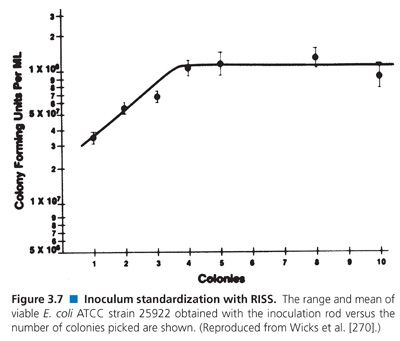
STANDARD BROTH DILUTION PROCEDURES
The focus of this chapter is on broth dilution procedures. However, it is worthwhile to note that comparisons of results of broth and agar dilution methodologies have shown them to be similar (92). In some instances, as noted by Lorian (93), the differences for quinolones have been significant. There has been a considerable range of variability in studies, depending on the method used, the antimicrobial agent studied, and the organisms tested. A number of investigations have also compared microdilution and macrotube dilution techniques (37,94,95). Tables 3.23 and 3.24 present some of the results. Clearly, for the majority of agents tested, there were few strains that varied by more than plus or minus one dilution.
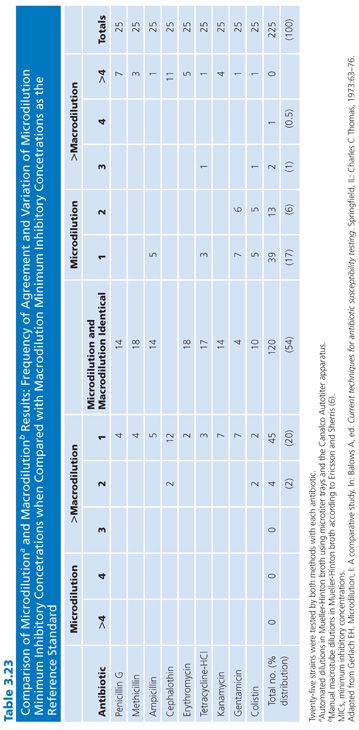
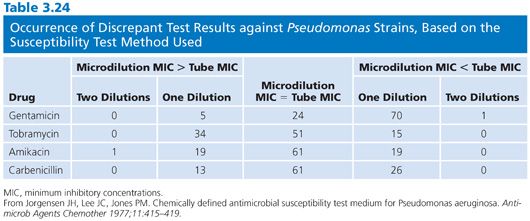
Comparative evaluations of the microdilution and macrodilution broth procedures are noted here to provide a historical perspective. Since the 1980s, there have been no evaluations of these two broth methodologies, owing in part to the popularity of the microdilution procedure. Furthermore, commercial applications of the miniaturized technique that began in the mid-1970s have made it practical for laboratories to provide MICs for all isolates, or at least for all those they wish to report on. In general, microdilution test results are essentially equivalent to those obtained with the standardized macrobroth dilution procedure. For certain organisms, such as gram-negative bacilli, the microdilution results are approximately one dilution step lower than those obtained with the macrodilution broth technique. This seems to be a general feature of the microdilution technique and may be partially due to the way end points are read. Minimal turbidity, which is visible in a test tube, may not be readily observed in a microtiter well. Another possible explanation may involve the inoculum. Although in their final concentrations the inocula in the two systems contain approximately 5 × 105 organisms and are comparable, the absolute number of viable cells delivered into the microtiter well is 1 log unit less than that inoculated into the test tube.
Standard Microdilution Broth Procedure
The performance of microdilution tests has been described by a number of authors using in-house panels prepared by mechanical semiautomated or automated equipment (29,94,96–101) or commercial test panels routinely prepared or customized for the user. Commercially prepared test panel systems employ either frozen antimicrobial solutions in medium or dried preparations that need to be rehydrated by adding diluent and inoculum to each well. The test panel state of some commercial preparations and their method of inoculation are noted in Table 3.25.
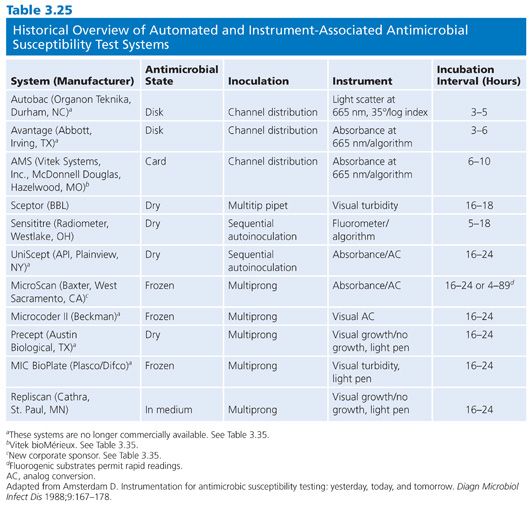
For preparation of standard microdilution panels in the laboratory, the following general recommendations and directions can be followed (29,102). The antimicrobial agent stock solutions are prepared and stored as noted in Tables 3.4 and 3.7 and as found in CLSI (NCCLS) standard M7-A6 (10). The recommended dilutions have been included in Table 3.4, and the flexibility dilution schedule need not be limited to serial twofold increments.
The recommended broth for testing is Mueller-Hinton supplemented with 50 mg/L Ca2+ and 25 mg/L Mg2+. These concentrations are achieved by the addition of the appropriate amounts of filter-sterilized CaCl2 and MgCl2 stock solutions to cold sterile broth. Ideally, microdilution trays should be prepared each day they are used. However, with the availability of semiautomated dispensing devices for preparing dilutions and dispensing prediluted agent, it is possible to prepare large numbers of trays at one time and store them frozen until required. As trays are filled, they are stacked in groups of 5 to 10 and are either covered with an empty tray on top or placed and sealed in plastic bags and frozen at 20°C or 70°C. Household freezers are satisfactory, but they should not contain self-defrosting units, because fluctuations in temperature during the defrost cycle thaw and refreeze the antimicrobial agents and thus contribute to their deterioration. Trays quick-frozen at 70°C and stored at 20°C have a useful shelf life of about 6 weeks. Storage at 70°C significantly increases the shelf life to approximately 3 months. Once a group of trays is removed from the freezer, they should be allowed to warm at room temperature prior to use. Unused thawed trays should be discarded and never refrozen.
Actively growing broth cultures are diluted to a McFarland value of 0.5 (as described earlier). Multipoint plastic or metal replicators (inoculators) are used in several commercial and semiautomated systems. After inoculation, each well should contain approximately 5 × 105 CFU/mL (5 × 104 CFU/well).
After inoculation of microdilution trays, they should be covered with sealing tape to minimize evaporation. Alternatively, large numbers of trays can be stacked and covered with an empty tray. After 16 to 20 hours of incubation, trays may be examined from below with a reflective viewer and MICs determined. The trays can also be read visually from the top. The end point MIC is the lowest concentration of drug at which the microorganism tested does not demonstrate visible growth. In judging the end point, it is necessary to compare the growth (or absence of growth) in the test wells with the growth (or absence of growth) in the well without the antimicrobial agent. End points are easily read as turbid wells or clear wells. For some antimicrobials, such as the sulfonamides and trimethoprim, end points may trail. The end point for these drugs should be read as an 80% to 90% decrease in growth compared with growth in the control well.
Standard Macrodilution Broth Procedure
The variables that affect the outcome of dilution tests were outlined earlier and were reviewed in the preceding section. The procedures for performing the macrodilution broth test have been described by several authors (6,24,25,32,103–106). Errors and statistical variability of dilution procedures have been reviewed (30). The basic methodology described in the ICS report (6) has proven satisfactory for many laboratories, and Table 3.5 presents a scheme recommended in the ICS report for the preparation of antibiotic dilutions. An alternative dilution schedule for preparing a larger number of tubes is shown in Table 3.6. Because the dilutions are pipetted directly into blocks of three dilution tubes with one pipette, the chance of error is significantly reduced. A sequential twofold dilution method is summarized from the work of Jones et al. (107) as follows. The working antimicrobial solution is prepared by diluting the drug and MHB to the highest final concentration desired. The test is performed in 13 100-mm screw-capped (or cotton-plugged, etc.) test tubes. For a limited number of tests, twofold dilutions are prepared directly in test tubes as follows: 2 mL of the working solution of the drug is added to test tube 1 of the dilution series. To each remaining tube, 1 mL of MHB is added. With a sterile pipette, 1 mL is transferred from tube 1 to tube 2. After thorough mixing, 1 mL is transferred (using a separate pipette for this and each succeeding transfer) to tube 3. This process is continued to the next to last tube, from which 1 mL is removed and discarded. The last tube receives no antimicrobial agent and serves as a growth control. The final concentrations of antimicrobial agents in this test are half those of the initial dilution series because of the addition of an equal volume of inoculum in broth. The inoculum is prepared and adjusted, as noted earlier, to contain 105 to 106 CFU/mL, by adjusting the turbidity of the broth culture to match the McFarland 0.5 standard. It is then further diluted 1:200 in broth, and 1 mL of the adjusted inoculum is added to each test tube. Tubes are incubated at 35°C for 16 to 20 hours. The lowest concentration of antimicrobial agent that results in complete inhibition of visible growth represents the MIC. A very faint haziness or a small button is usually disregarded.
Quality Control
For in vitro susceptibility test results to be meaningful for selecting appropriate antimicrobial agents and monitoring their use for the treatment of infection, they need to be accurate and reproducible. Because of the potential for variation, emphasis has been focused on strict adherence to methods and reference procedures (10,11). The development of QC parameters has played a significant role in the high level of performance obtained by most laboratories. Keys to this performance are the application of standard reference strains with known reactivity and the assessment of qualitative and quantitative end points.
The ideal reference strain for QC of dilution susceptibility methods should have MIC end points near the middle of the range of concentrations being tested for a given drug, or at least no closer than two dilutions from the extremes of the test range included (102). Thus, in QC for dilution testing, it has been necessary to deviate from established QC strains that have been used for disk diffusion testing. For example, S. aureus American Type Culture Collection (ATCC) strain 29213, a weak β-lactamase producer, is recommended instead of ATCC strain 25923. Additionally, S. faecalis ATCC strain 29212 and E. coli ATCC strain 35218 have been recommended as controls for β-lactamase inhibitors such as clavulanic acid and sulbactam. A sixth control strain, H. influenzae ATCC strain 49247, has recently been proposed (by CLSI [NCCLS]) for testing drugs against H. influenzae (10).
Studies of the precision and accuracy of macrodilution and microdilution MIC end points with the older established QC strains have shown that reproducibility should be within plus or minus 1 log2 dilution interval for 95% of the replicates (32,103) and that most tests should fall at the modes. The ranges of MICs expected for several contemporary antimicrobial agents against the reference strains are indicated in Table 3.26.
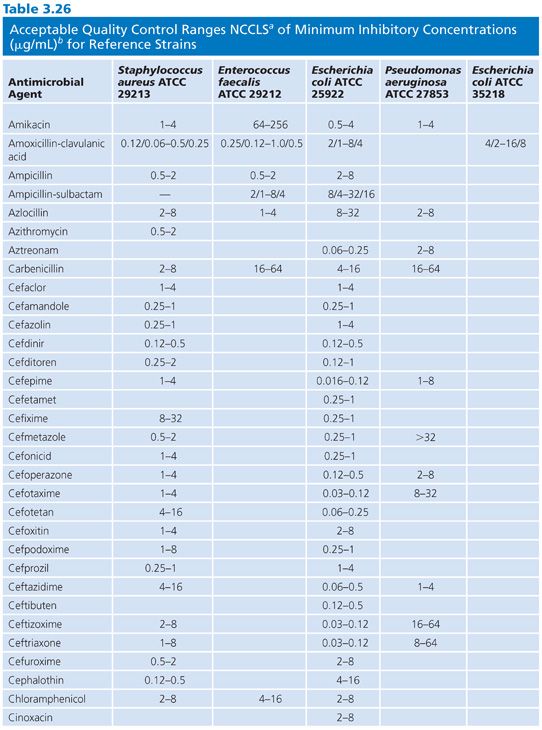
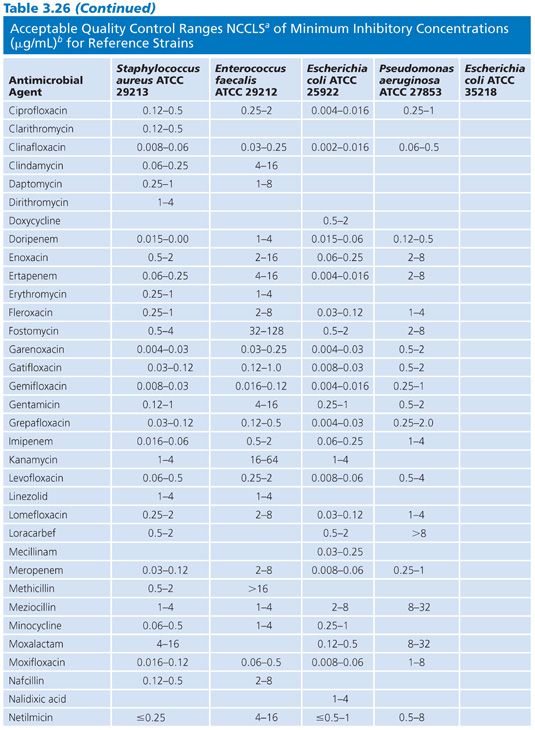
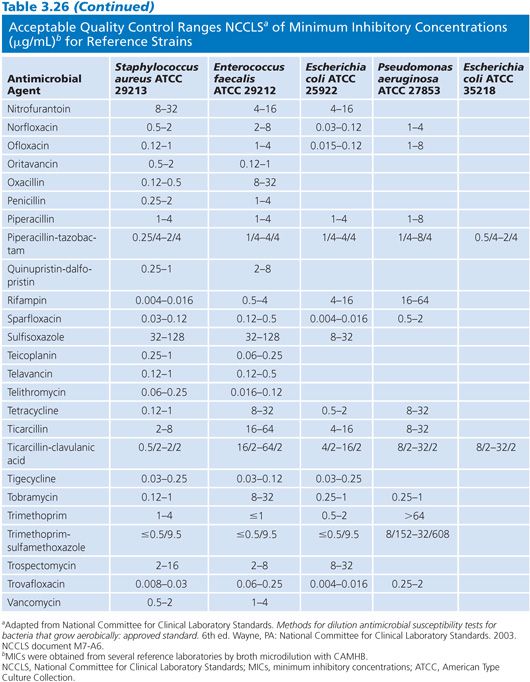
Control reference strains should remain genetically and phenotypically stable over many replications and long-term storage. Control strains must be stored using procedures designed to minimize chances of mutation or variant selection (32). It is suggested that, for long-term storage, QC strains be lyophilized or frozen in a stabilizing medium such as whole sheep blood or 15% glycerol in an enriched broth such as BHI or 50% serum in broth. Freezing at 60°C (or below) is preferable to storing in conventional freezers (20°C). Strains can be maintained for short-term storage at 4°C for approximately 2 weeks on agar (soybean digest casein).
When new batches or lots of microdilution trays are received from a commercial source or prepared, they should be tested with reference strains to determine their acceptability. The MICs resulting from QC testing should be no more than one dilution interval above or below the anticipated MIC. If the difference is greater, either the batch is rejected or the results with the affected antimicrobial agent are not recorded or reported. Additionally, representative uninoculated trays should be tested for sterility of the medium. QC should be done on a periodic basis after reproducibility and accuracy have been documented by daily QC practice (10,108). These QC procedures provide a review of variables such as antimicrobial potency and stability, instrument function, and technical proficiency. The MICs obtained with each reference strain should be maintained in a record book for ongoing review.
Some additional QC procedures are necessary for broth dilution tests but are less critical for agar or disk diffusion tests. A purity control plate for each isolate tested is subcultured, for isolation on an appropriate agar medium, directly from the final inoculum suspension to detect potential contamination or mixed cultures. A growth control tube (or well) free of antimicrobial agents is included within each panel set to ensure adequate growth. This also serves as a turbidity control (a comparative aid) when reading end points. The inoculum should be measured periodically by a direct dilution plate count of the time 0 inoculum from the growth tube or well. Finally, the proficiency of the observers determining end points should be monitored periodically by comparing their results with those from a standard reader to ensure uniformity between different observers reading the same plate.
There are several types of susceptibility test results that, when obtained, require further confirmation or investigation. Forty-five nonsusceptible phenotypes are listed in Table 3.27, and these may be associated with pre- or postanalytical (test) errors. In any event, these phenotypes warrant follow-up study.
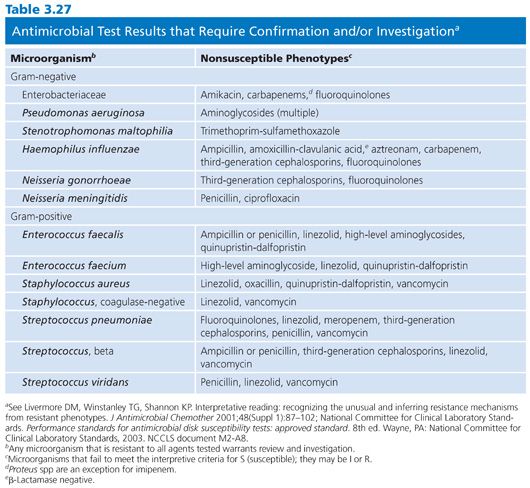
FASTIDIOUS AND UNUSUAL PATHOGENS
The standard broth procedures described thus far are applicable to routine antimicrobial susceptibility testing. The term routine reflects the fact that the testing is of rapidly growing, nonfastidious pathogens frequently encountered in the clinical setting. Routine susceptibility procedures, however, may not be applicable to predictably slow-growing microorganisms with prolonged lag times and/or slow generation times (109). Some clinically significant bacteria have characteristics that preclude their being tested by standard methods. They may grow too slowly, may require special nutrients or atmospheres, or simply may not have been tested with enough frequency to demonstrate that they can be tested accurately and reproducibly by the standard methods. These microorganisms have been termed fastidious and/or unusual. Specifically, fastidious organisms do not readily grow on Mueller-Hinton medium without supplementation. Unusual organisms may grow well on Mueller-Hinton medium, but studies have not been completed to demonstrate that they can be tested reliably by standard methods. Testing the susceptibility of unusual organisms to antimicrobial agents may also present special problems.
In the past, many of these organisms did not require susceptibility tests because they were known to be universally susceptible to an appropriate antimicrobial agent that was not toxic and could reach sufficient levels to effect a clinical cure. “Resistant” strains have emerged, however. Resistance to β-lactams is most often due to production of β-lactamase, which may be constitutive or inducible and may be mediated by either chromosomal or plasmid genes (80,110). In some species, such as H. influenzae and N. gonorrhoeae, penicillin and ampicillin resistance has been typically caused by the acquisition of plasmids that mediate constitutive β-lactamase production by the organisms (111,112). In other species, such as S. pneumoniae, penicillin resistance is not due to β-lactamase production caused by plasmids but is chromosomally mediated and is due to the alteration of PBPs (113).
These developments have produced challenges for clinicians because previously employed empirical therapies associated with these fastidious or unusual organisms may not be adequate. Additional laboratory testing is sometimes required to support a prediction of therapeutic success.
For some organisms, such as group A streptococci, susceptibility tests are not necessary because these organisms have maintained (with some exceptions) universal susceptibility to penicillin, the drug of choice. However, for S. pneumoniae, especially if it was isolated from a putatively sterile site, a test to determine susceptibility to penicillin is indicated, because some of the strains may be relatively or completely resistant to penicillin. If it becomes necessary to perform susceptibility tests on clinical isolates for which no standard method has been described, it is usually best to determine the MIC using the general broth dilution method described earlier and in CLSI (NCCLS) standard M7-A6. For testing of infrequently isolated or fastidious, see CLSI document M45-A2. Table 3.28 details the antimicrobial susceptibility methods that can be used for these fastidious or unusual organisms. It is obvious that certain microorganisms have been omitted, notably anaerobes, Mycobacterium tuberculosis, Chlamydia spp, Mycoplasma spp, and spirochetes. For these groups, readers can refer to appropriate chapters in this volume.
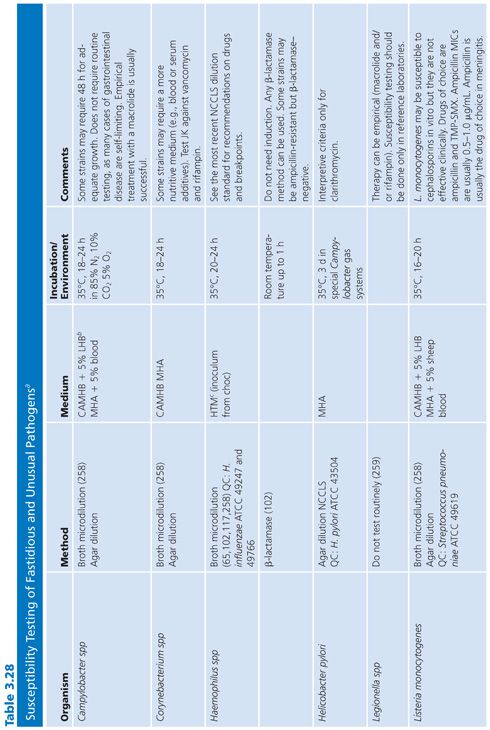
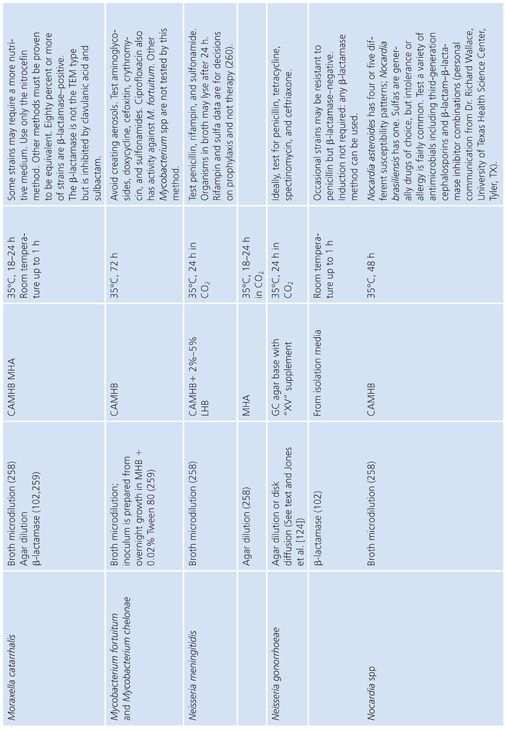
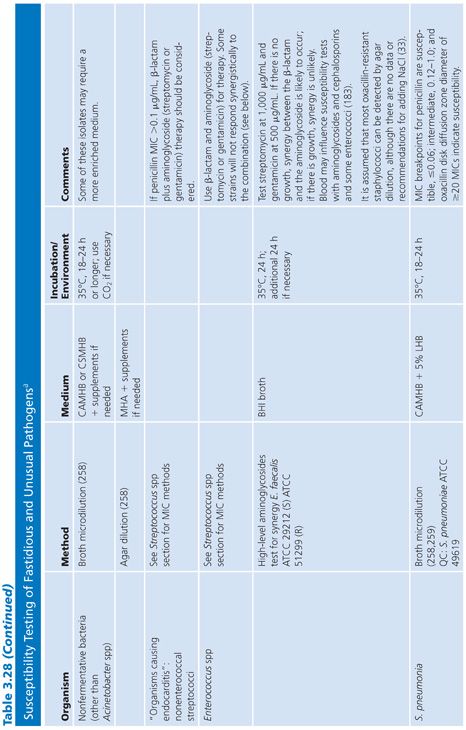
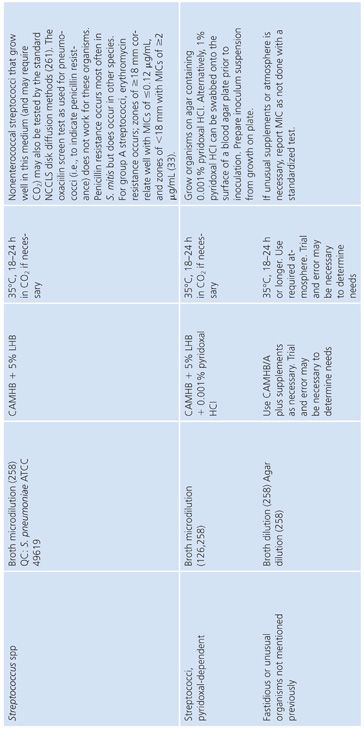
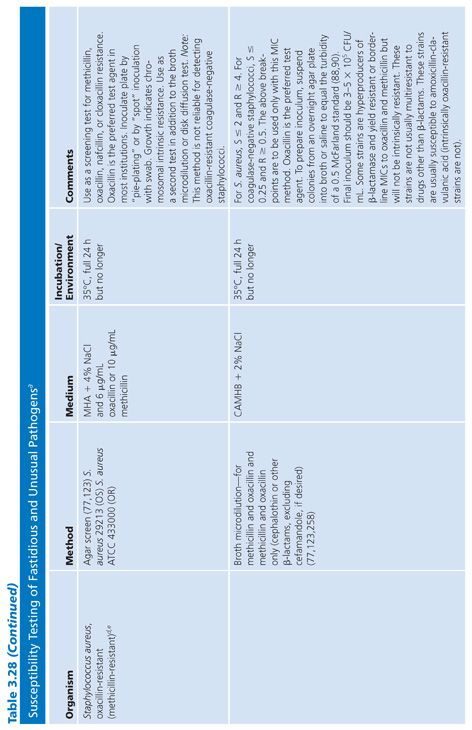
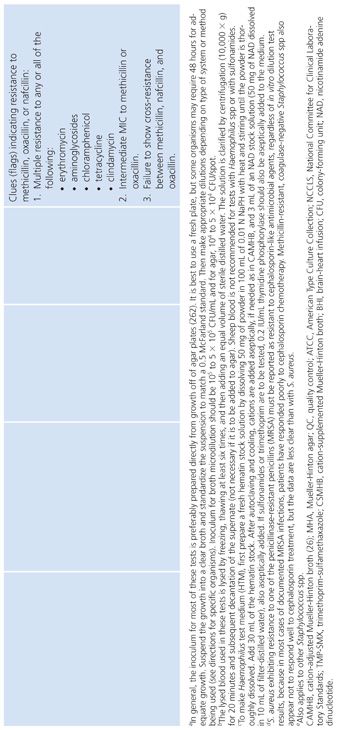
For the antimicrobial susceptibility testing of fastidious and/or infrequently encountered bacteria, the CLSI has published guidelines for testing with interpretive breakpoints (114). These guidelines encompass the following bacterial groups.
Aeromonas spp
Bacillus spp (other than Bacillus anthracis)
Campylobacter coli and Campylobacter jejuni
Corynebacterium spp
Erysipelothrix rhusiopathiae
HACEK group (Aggregatibacter actinomycetemcomitans and Aggregatibacter aphrophilus, Cardiobacterium hominis, Eikenella corrodens, and Kingella kingae)
Lactobacillus spp
Leuconostoc spp
Listeria monocytogenes
Moraxella catarrhalis
Pasteurella spp
Pediococcus spp
Plesiomonas shigelloides
Streptococci, nutritional dependent spp (now Abiotrophia and Granulicatellas spp)
In addition to the microorganisms listed earlier, the CLSI has proposed guidelines for the standardized testing of the generally recognized agents of bioterrorism, namely Bacillus anthracis, Francisella tularensis, Brucella spp, Yersinia pestis, and Burkholderia pseudomallei. (See Table 2 of CLSI M45-A2 [114].) As of this writing, standardized testing for other fastidious bacteria, for example, Legionella and Bordetella, are unavailable because these less frequently encountered bacterial infections generally respond to clinically recommended antimicrobial agents.
Haemophilus influenzae
H. influenzae and other Haemophilus species are examples of common clinical pathogens with special growth requirements. The widespread emergence of β-lactamase–producing strains has made rapid detection of β-lactamase production and, in turn, detection of ampicillin resistance in Haemophilis important objectives (102,115–117).
Antimicrobial resistance among clinical isolates of H. influenzae has been monitored in the United States, Canada, and Europe. In a comprehensive study (118) involving isolates in the United States, 20% of all H. influenzae isolates were ampicillin-resistant by virtue of β-lactamase production. Enzyme-mediated resistance to ampicillin is approximately twice as common in serotype B strains (31.7%) as in non–B strains (15.6%). As a result of the production of the inactivating enzyme chloramphenicol acetyltransferase (119), occasional resistance has been noted with chloramphenicol, as well as resistance to tetracycline, trimethoprim-sulfamethoxazole, rifampin, and first-generation cephalosporins.
Because of the worldwide prevalence of β-lactamase–producing strains of H. influenzae, it is imperative that clinical laboratories routinely perform β-lactamase studies on all clinically significant isolates. Several media formulations have been employed for dilution or diffusion susceptibility testing with H. influenzae (65,120). Although individual methods may have been useful in individual laboratories, a common problem with these media formulations has been the complexity of their preparation and their opaque nature. Recently, a new simplified medium, HTM, has been developed; it avoids many of these problems (65). HTM is optically clear, stable, and reproducible from lot to lot and is currently available commercially from several manufacturers. The use of HTM has been advocated by CLSI (NCCLS) for both dilution and diffusion tests with H. influenzae (10). Guidelines for the interpretation of MIC and QC results with an H. influenzae reference strain are included in this chapter and are contained in Table 3.28.
In addition to H. influenzae, other problem organisms have been tested, as indicated in Table 3.28. Following is a discussion of current approaches to the testing of MRSA, N. gonorrhoeae, S. pneumoniae, S. viridans, L. monocytogenes, E. corrodens, and Chlamydia spp, along with appropriate references. In general, the use of a full incubation interval of 24 hours and the addition of 2% NaCl to cation-supplemented MHB have facilitated the testing of MRSA and have greatly enhanced the reliability of standard microdilution tests in detecting such organisms (121–123). Variable results have been obtained with different dilution methods for testing MRSA strains with cephalosporins, and many strains appear fully susceptible to cephalosporins, which is inconsistent with treatment results. This has led to the standard recommendation that cephalosporin tests not be reported for MRSA and methicillin-resistant S. epidermidis (11).
Neisseria gonorrhoeae
The changing antimicrobial susceptibility of N. gonorrhoeae is an example of how clinical laboratories have had to modify their approaches and strategies in susceptibility testing. N. gonorrhoeae has developed resistance to all of the agents that have been recommended for gonorrhea therapy. When penicillin was the recommended therapy for N. gonorrhoeae infection, most isolates were initially susceptible. From the mid-1940s to the 1970s, there was a 24-fold increase in the dosage of procaine penicillin (2 × 105 units to 4.8 × 106 units). Penicillin is no longer the recommended therapy.
The resistance of N. gonorrhoeae to antibacterials is due either to multiple chromosomal mutations or to R factor plasmids. In 1987, the increasing prevalence of strains with β-lactamase plasmids prompted discontinuation of the use of penicillin as a single-dose therapy. Determining resistance, which is primarily a laboratory responsibility, affects epidemiologic surveillance and patient care.
A standardized laboratory method for monitoring the susceptibilities of gonococcal isolates was formulated and has been recommended by CLSI (NCCLS) based on a multicenter laboratory study whose purpose was to standardize disk diffusion and agar susceptibility tests (124). The recommended test medium is GC agar base with a defined XV-like supplement. Three QC organisms are required: N. gonorrhoeae ATCC strain 49226 (CDC F-18), N. gonorrhoeae WHO strain V, and S. aureus ATCC strain 25923.
The publication of the multicenter guidelines does not alter the need or methodology for detecting penicillinase-producing N. gonorrhoeae. Penicillinase-producing N. gonorrhoeae strains may be identified by detection of β-lactamase with a nitrocefin substrate (Table 3.28). Strains of N. gonorrhoeae that have chromosomally mediated resistance to antimicrobial agents or plasmid-mediated resistance to penicillin and/or tetracycline may be detected by measuring their susceptibilities by disk diffusion (124) and are not discussed here. Agar dilution methodology is preferred to broth dilution, as N. gonorrhoeae is known to autolyze in liquid media.
Streptococcus pneumoniae
Throughout the world, there have been increasing reports of the relative resistance of S. pneumoniae to penicillin as well as to other drugs such as tetracycline, erythromycin, clindamycin, and chloramphenicol (16,17). Nonsusceptible strains have been isolated in the United States with increasing frequency; thus, routine testing of isolates of S. pneumoniae is probably warranted. Satisfactory broth dilution tests can be performed with S. pneumoniae, provided that the broth medium is appropriately supplemented by adding 5% defibrinated sheep blood to freshly thawed MHB in microdilution trays that have been stored for no more than 2 months at 20°C. Commercially prepared test systems are available but have limitations and need to be verified and validated (125). Tests for other streptococci are indicated in Table 3.28.
Special studies related to susceptibility testing of several other species have been performed and are noted here. MICs, MBCs, and killing curves have been studied for L. monocytogenes using TS broth (126,127). Pasteurella multocida was tested in microtiter panels using MHB supplemented with 10% horse serum (128). Vanhoof et al. (129) evaluated inhibition and killing of Campylobacter jejuni in MHB. E. corrodens susceptibility testing has been accomplished using MHB supplemented with 0.5% lysed sheep blood (130). Mycoplasma spp have also been studied (131) in macrotube systems and by microdilution susceptibility testing (132,133). Unlike conventional bacteria, which can grow in artificial media, Chlamydia trachomatis has been tested against a variety of antimicrobial agents by incorporating antibiotic dilutions in cycloheximide-treated McCoy cell cultures (134,135). Utilizing this approach, MICs and MBCs can be determined (see Chapter 7).
Nocardia spp and Actinomycetes
In large part, problems associated with the susceptibility testing of this group of microorganisms relates to the new directions in the methods used for their identification. In the last 15 years, newer approaches such as molecular testing using 16S ribosomal RNA sequencing and Matrix-Assisted Laser Desorption Ionization-Time of Flight (MALDI-TOF) methodologies have been adopted (136,137). Although broth microdilution is the recommended methodology, false resistance using this approach has been documented, specifically for Nocardia spp (138). The generally recommended antimicrobial agents for primary testing are amikacin, amoxicillin-clavulanate, ceftriaxone, ciprofloxacin, clarithromycin, imipenem, linezolid, minocycline, moxifloxacin, trimethoprim-sulfamethoxazole and tobramycin. Secondary testing compromises cefepime, cefotaxime, doxycycline, rifampin, and vancomycin. Susceptibility testing for the aerobic actinomycetes requires 2 to 5 days, contrasted to 24 to 48 hours for species of Rhodococcus.
METHODS FOR DETECTION OF ANTIMICROBIAL RESISTANCE
Although there has been limited development of new antimicrobial agents and classes over the past 10 years, microorganisms have developed novel and, in some cases, multiresistant mechanisms to limit the clinical use of the available compounds. Several phenotypic and genotypic methods are available as screening tests, which do not result in an MIC but possess the necessary accuracy that confirmatory testing is not required and results can be used to guide antimicrobial therapy. Molecular, that is, genotypic, methods are reviewed by Hegstad et al. in Chapter 9 of this volume “Molecular Methods for Detection of Antibacterial Resistance Genes: Rationale and Applications”; phenotypic approach will be discussed here.
β-Lactamase Detection
Three iterations of this assay are available: acidometric, iodometric, and chromogenic; the latter being the most common. Results are typically available within 60 minutes. Although β-lactamase production inactivates ampicillin, amoxicillin, and penicillin for N. gonorrhoeae, it detects only one form of resistance and for chromosomally mediated resistance with PBPs, resistance can only be detected by disk diffusion or agar dilution methods. Because some β-lactamase–producing organisms (e.g., Staphylococcus) may produce detectable levels of the enzyme after induction, it is generally recommended that for serious infections requiring penicillin therapy an MIC be performed following the rapid phenotypic β-lactamase assay.
Detection of Methicillin Resistance: Methicillin-Resistant Staphylococcus aureus and Related Resistance Phenotypes
Rapid and early diagnosis of MRSA is necessary for prompt initiation of appropriate antimicrobial therapy. The most common method is culture modified more recently with the application of chromogenic agars (139). Other than molecular approaches there are no equivalent rapid “broth” phenotypic approaches. However, broth phenotypic resistance screening tests for S. aureus are extant and address mecA-mediated oxacillin resistance, inducible clindamycin resistance, and high-level murpirocin resistance (Table 3.29).
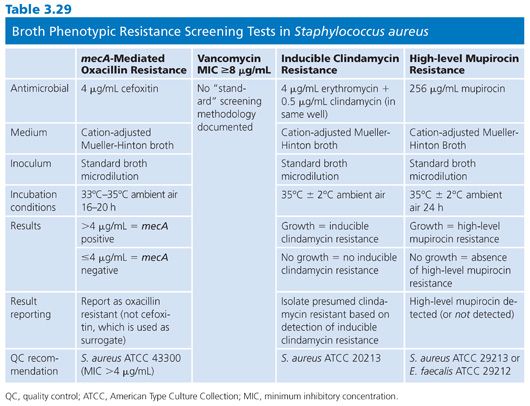
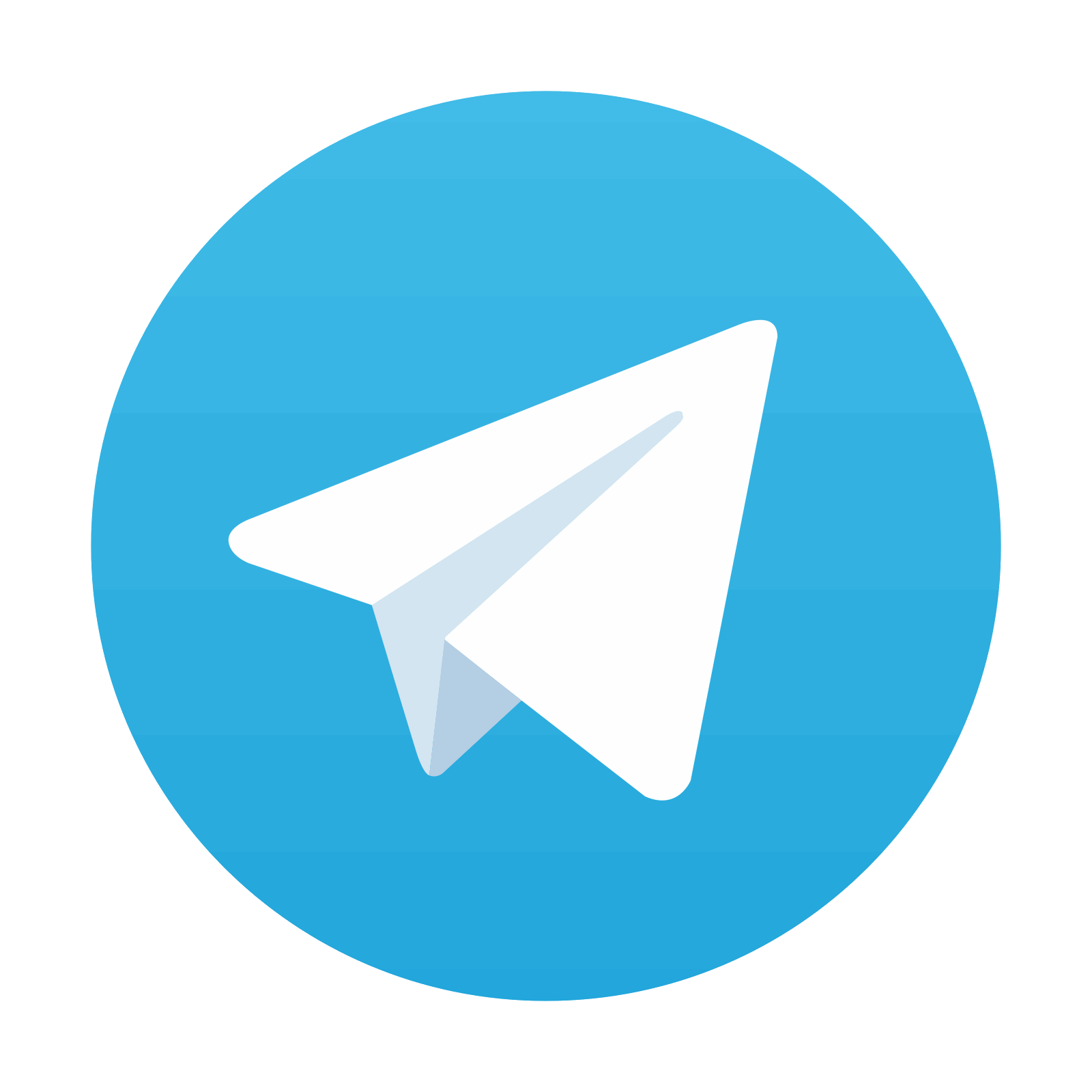
Stay updated, free articles. Join our Telegram channel

Full access? Get Clinical Tree
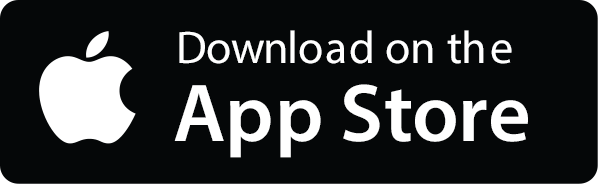
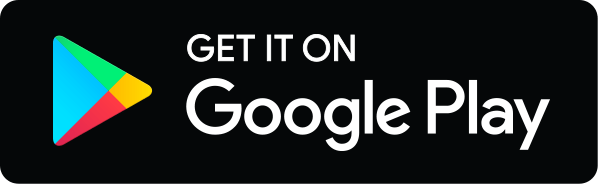