CHAPTER 20 Structure and Function of the Respiratory System
The primary function of the lung is gas exchange, which consists of movement of O2 into the body and removal of CO2. The lung also plays a role in host defense by functioning as a primary barrier between the outside world and the inside of the body. Finally, the lung is a metabolic organ that synthesizes and metabolizes numerous compounds. This chapter provides an overview of lung anatomy (i.e., upper and lower airways, muscles, innervation), growth and development of the normal and aging lung, and the fluids lining various anatomic sites, with special emphasis on unique features relative to the lung. Metabolic features of the lung are discussed in Chapter 25.
LUNG ANATOMY
Upper Airways—Nose, Sinuses, Larynx
The respiratory system begins at the nose and ends in the most distal alveolus. Thus, the nasal cavity, the posterior pharynx, the glottis and vocal cords, the trachea, and all divisions of the tracheobronchial tree are included in the respiratory system. The upper airway consists of all structures from the nose to the vocal cords, including sinuses and the larynx, whereas the lower airway consists of the trachea, airways, and alveoli. The major function of the upper airways is to “condition” inspired air so that by the time it reaches the trachea, it is at body temperature and fully humidified. The nose also functions to filter, entrap, and clear particles larger than 10 μm in size. Finally, the nose provides the sense of smell. Neuronal endings in the roof of the nose above the superior turbinate carry impulses through the cribriform plate to the olfactory bulb. The volume of the nose in an adult is approximately 20 mL, but its surface area is greatly increased by the nasal turbinates, which are a series of three continuous ribbons of tissue that protrude into the nasal cavity (Fig. 20-1). In humans, the volume of air entering the nares each day is on the order of 10,000 to 15,000 L. Resistance to airflow in the nose during quiet breathing accounts for approximately 50% of the total resistance of the respiratory system, which is about 8 cm H2O/L/sec. Nasal resistance increases with viral infections and with increased airflow, such as during exercise. When nasal resistance becomes too high, mouth breathing begins.
The major structures of the larynx include the epiglottis, arytenoids, and vocal cords (Fig. 20-1). With some infections, these structures can become edematous (swollen) and contribute significantly to airflow resistance. The epiglottis and arytenoids “hood” or cover the vocal cords during swallowing. Thus, under normal circumstances, the epiglottis and arytenoids function to prevent aspiration of food and liquid into the lower respiratory tract. The act of swallowing food after mastication (chewing) usually occurs within 2 seconds, and it is closely synchronized with muscle reflexes that coordinate opening and closing of the airway. Hence, air is allowed to enter the lower airways and food and liquids are kept out. Patients with some neuromuscular diseases have altered muscle reflexes and can lose this coordinated swallowing mechanism. Thus, they may become susceptible to aspiration of food and liquid, which poses a risk for pneumonia.
Lower Airways—Trachea, Bronchi, Bronchioles, Respiratory Unit
The right lung, located in the right hemithorax, is divided into three lobes (upper, middle, and lower) by two interlobular fissures (oblique, horizontal), whereas the left lung, located in the left hemithorax, is divided into two lobes (upper, including the lingula, and lower) by an oblique fissure (Fig. 20-2). Both the right and left lungs are covered by a thin membrane called the visceral pleura and are encased by another membrane called the parietal pleura. The interface of these two pleuras allows for smooth gliding of the lung as it expands in the chest and produces a potential space. Air can enter between the visceral and parietal pleuras because of either trauma, surgery, or rupture of a group of alveoli creating a pneumothorax. Fluid can also enter this space and create a pleural effusion or, in the case of severe infection, empyema.
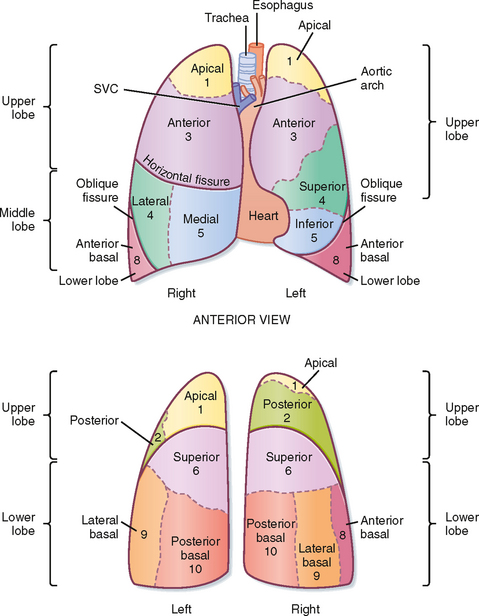
Figure 20-2 Topography of the lung demonstrating the lobes, segments, and fissures. The fissures (or chasms) demarcate the lobes in each lung. Numbers refer to specific bronchopulmonary segments, as presented in Figure 20-3. SVC, superior vena cava.
The trachea bifurcates (branches) into two main stem bronchi (Fig. 20-3). These main stem bronchi then divide (like the branches of a tree) into lobar bronchi (one for each lobe), which in turn divide into segmental bronchi (Figs. 20-3 and 20-4) and into smaller and smaller branches (bronchioles) until reaching the alveolus (Fig. 20-5). The region of the lung supplied by a segmental bronchus is the functional anatomic unit of the lung. Bronchi and bronchioles differ not only in size but also by the presence of cartilage, the type of epithelium, and their blood supply (Table 20-1). The airways continue to divide in a dichotomous or asymmetric branching pattern until they form terminal bronchioles that are distinguished by being the smallest airways without alveoli. Each branching of the respiratory bronchioles results in decreased diameter; however, the total surface area for that generation increases in size and number until the respiratory bronchiole terminates in an opening to a group of alveoli (Fig. 20-5).
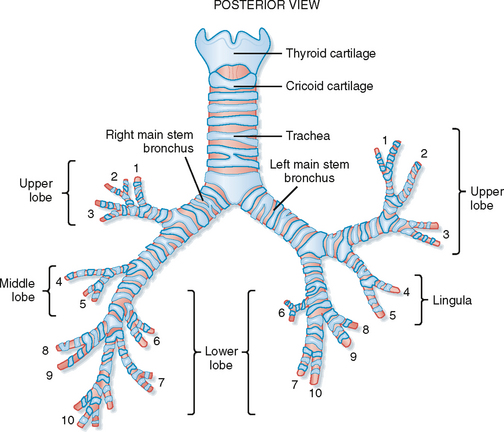
Figure 20-3 Bronchopulmonary segments, anterior view: 1, apical; 2, posterior; 3, anterior; 4, lateral (superior); 5, medial (inferior); 6, superior; 7, medial basal; 8, anterior basal; 9, lateral basal; 10, posterior basal. The numbers are the same as in Figure 20-2.
The region of the lung supplied by a segmental bronchus is called a bronchopulmonary segment and is the functional anatomic unit of the lung. Because of its structure, segments of the lung that have become irreversibly diseased can easily be surgically removed. The basic physiological unit of the lung is the respiratory or gas-exchanging unit (respiratory unit), which consists of the respiratory bronchioles, the alveolar ducts, and the alveoli (Figs. 20-4 and 20-5). Bronchi that contain cartilage and nonrespiratory bronchioles (i.e., lacking alveoli) in which cartilage is absent serve to move gas from the airways to the alveoli and are referred to as the conducting airways. This area of the lung is approximately 150 mL in volume (or ≈30% of a normal breath), does not participate in gas exchange, and forms the anatomic dead space. The respiratory bronchioles with alveoli and the area from the terminal or nonrespiratory bronchioles to the alveoli are where all gas exchange occurs. This region is only approximately 5 mm long, but it is the single largest volume of the lung at approximately 2500 mL and has a surface area of 70 m2 when the lung and chest wall are at the resting volume (Table 20-1).
The alveoli are polygonal in shape and about 250 μm in diameter. An adult has around 5 × 108 alveoli (Fig. 20-6), which are composed of type I and type II epithelial cells. Under normal conditions type I and type II cells exist in a 1 : 1 ratio. The type I cell occupies 96% to 98% of the surface area of the alveolus, and it is the primary site for gas exchange. The thin cytoplasm of type I cells is ideal for optimal gas diffusion. In addition, the basement membrane of type I cells and the capillary endothelium are fused, which minimizes the distance for gas diffusion and thereby facilitates gas exchange. The type II epithelial cell is small and cuboidal and is usually found in the “corners” of the alveolus, where it occupies 2% to 4% of its surface area. Type II cells synthesize pulmonary surfactant, which reduces surface tension in the alveolar fluid and is responsible for regeneration of the alveolar structure subsequent to injury.
< div class='tao-gold-member'>
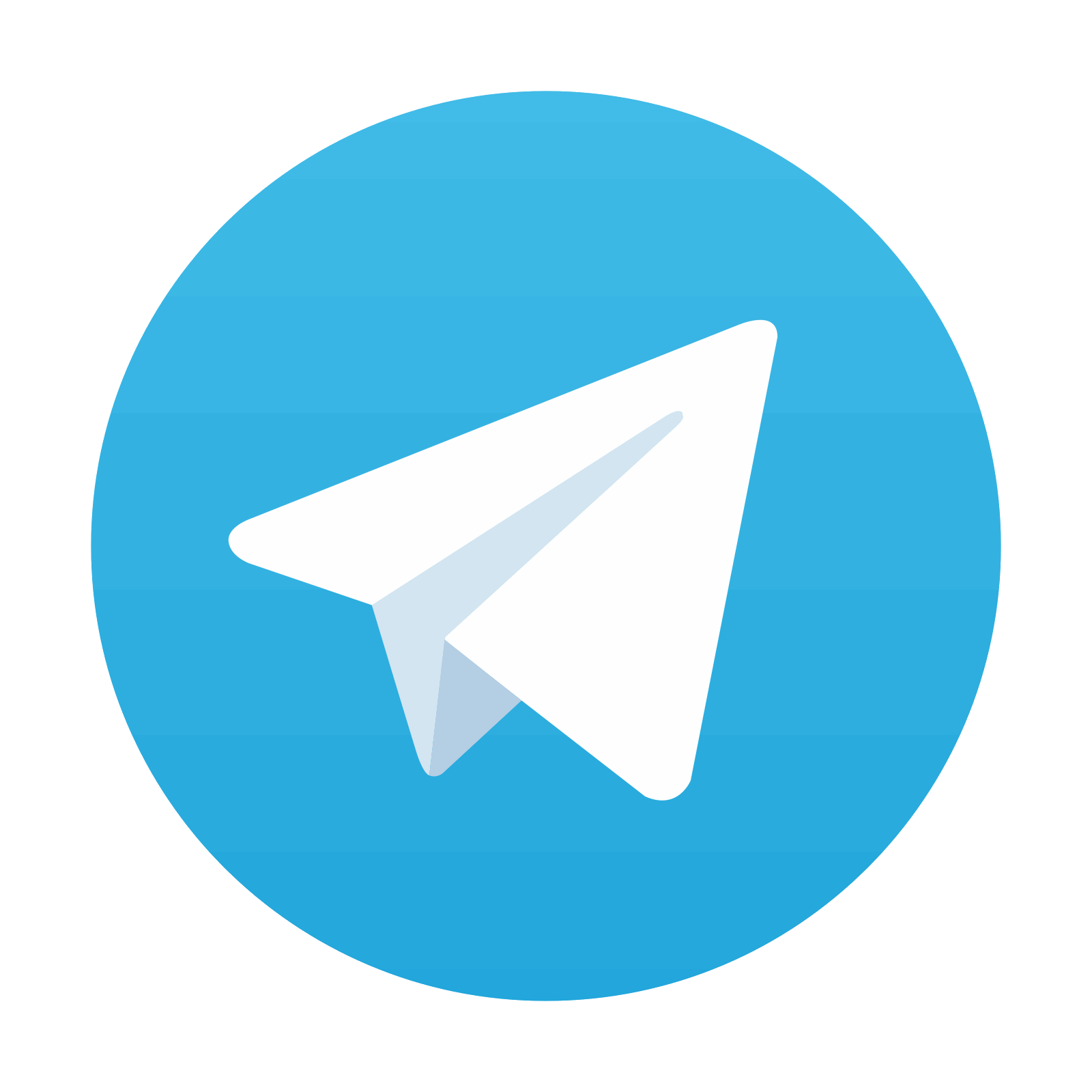
Stay updated, free articles. Join our Telegram channel

Full access? Get Clinical Tree
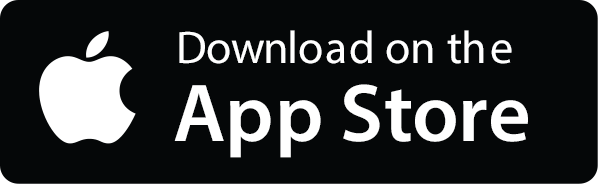
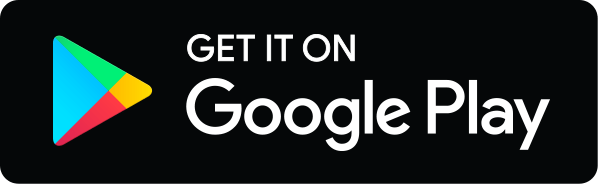