Learning Outcomes
After completing this chapter, you will be able to
Describe the historic development of nuclear pharmacy practice.
Explain the basic concepts of radioactivity and nuclear medicine as they relate to nuclear pharmacy practice.
Explain the role of nuclear medicine and nuclear pharmacy in patient diagnosis and treatment.
Describe the various aspects of nuclear pharmacy practice.
Identify the common areas for technician involvement in the practice of nuclear pharmacy.
Explain the role of pharmacy technicians in compounding specialties such as veterinary pharmacy practice.
Compare the advantages and disadvantages of the types of infusion systems available for use in a patient’s home.
Key Terms
activity units (mCi) | Radiopharmaceuticals are described by activity units, which relate to the number of atoms that give off a radioactive emission per unit of time. The most common activity unit used in the United States is the Curie (Ci). In a nuclear pharmacy, the amount of radioactivity is small, so the millicurie (mCi) unit is mainly used. |
assay | Describes the activity per unit of volume, measured as mCi/mL. |
gamma photon | Type of radioactive emission. Gamma photons are electromagnetic waves (like x-rays) that have the ability to travel far enough to leave the patient’s body and be detected by nuclear medicine imaging equipment. |
Location of Nuclear Pharmacies
Restricted and Nonrestricted Areas
Unique Aspects of Nuclear Pharmacy
Handling Radioactive Materials
Using Specialized Instrumentation
Future Directions of Nuclear Pharmacy
Unique Aspects of Veterinary Pharmacy |
|
The pharmacy profession has always provided multiple practice opportunities for practitioners, both pharmacists and technicians alike. The profession has evolved, as practitioners of the past recognized that the multifaceted training and skill set that form the backbone of the pharmacy profession could be applied to a variety of unique practice settings. With the formation of the Board of Pharmaceutical Specialties (BPS) in 1976, a mechanism was put into place that allowed these practitioners to unify and establish standards that recognize the significance of these nontraditional practice settings.
The role of the pharmacy technician has expanded into these new areas, providing a broader number of choices for professional development and advancement. This chapter focuses on two specialties: nuclear pharmacy (the first specialty area recognized by the BPS) and veterinary pharmacy (a unique compounding practice site). The intent of this chapter is to give a pharmacy technician a brief overview of these two areas of practice and increase awareness of these (and other) specialty areas of practice. It does not attempt to provide every detail that would be needed to work in these settings. Although specialty areas of practice are not as common as traditional pharmacy employment opportunities (and may be more challenging to identify and pursue), they provide yet another choice for technicians who are interested in broadening their experience and expertise as pharmacy practitioners.
Nuclear Pharmacy
Nuclear pharmacy is an area of pharmacy practice that involves the preparation and dispensing of radioactive pharmaceutical products, or radiopharmaceuticals, for use in the diagnosis and treatment of disease. Nuclear pharmacy works closely with nuclear medicine departments in hospitals and clinic settings to provide these agents in a safe, effective, and timely manner for use in their patient population. The use of radioactivity in the products that are compounded and dispensed from nuclear pharmacies adds a significant layer of complexity to the process; as a result,
the practice of nuclear pharmacy involves considerable regulatory oversight to ensure the safety of both patients and practitioners who are involved in the preparation and dispensing process.
Basic Concepts
Radioactivity involves the transfer of excess energy into a radioactive emission. Within an atom, the nucleus contains protons (positive [+] charge) and neutrons (neutral charge). Surrounding the nucleus are electrons (negative [-] charge). Some atoms contain an imbalance in the ratios between the protons and neutrons within the nucleus, which makes them unstable. In order to return to a more stable state, the atom must release some of the excess energy. In some atoms, this excess energy is given off as a radioactive emission.
Radioactive material has a distinct characteristic called a half-life. The half-life of a radioactive material is the amount of time that it takes for one-half of the material to give up its excess energy in the form of a radioactive emission.
Radioactive materials can occur naturally or be created using various techniques. Naturally occurring radioactive material (NORM) is the radioactive form of a naturally occurring element. Radon gas, which is a concern to many homeowners, is a radioactive emission that occurs due to the elemental composition of the earth. The sunand energy-creating events that occur throughout the universe create cosmic radiation, a naturally occurring radiation source that is enhanced when traveling by air or residing at high altitudes. Even the human body contains small amounts of radioactive carbon and potassium. Naturally occurring radiation is always present in small amounts, and it is impossible to completely remove radioactivity from daily life. Naturally occurring radioactive material is not particularly useful for medical imaging because most such elements have a very long half-life.
Most medical applications use radioactive materials that are created in a nuclear reactor or an accelerator. People are generally familiar with the concept of a nuclear reactor, which can be used to generate power. Medical isotopes are produced using the same technology, but on a significantly smaller scale. In a reactor, a large atom (usually uranium) is split in a process called fission. Some of the byproducts generated are radioactive and can be collected and purified to be used in medical applications. In an accelerator, a stable atom is bombarded with a positively or negatively charged particle which creates an imbalance in the atom. These agents have shorter half-lives and are more useful for performing nuclear medicine imaging.
Nuclear medicine plays an important role in the diagnosis and treatment of disease. Although detailed information about this radiologic practice specialty is beyond the scope of this book, in broad terms, diagnostic nuclear medicine studies involve attaching a small amount of radioactive material to a compound (called a ligand) that is known to behave a certain way when it is introduced into the body. Once administered, the ligand moves through the patient’s body, following a physiologic process that occurs within that patient (e.g., elimination of waste through the kidney, remodeling of bone structure, and blood flow to the heart muscle). As the ligand moves through the body, the radioactive material gives off a radioactive emission called a gamma photon, which passes out of the patient’s body and into a detector located near the patient. The radioactive emissions are detected and mapped to identify how the tracer moves through the patient’s body. From these data, physicians can gain information about how the particular organ is working, as well as the physiologic process that should be occurring. As an example, in renal imaging, the organ of interest would be the kidney, while the physiologic process of concern would be excretion of waste products. The agents used for renal imaging are taken up by the kidney just like typical waste products in the blood would be. The agent is removed from the kidney in the urine and moves to the bladder. By watching the movement of the radioactive emissions as the agent moves through the kidney, it is possible to determine if there is adequate blood flow to the kidneys, if the kidneys are functioning properly, and if urine is able to pass out of the kidney and into the bladder. Examples of common renal images are found in figure 6-1.
The functional information provided by nuclear medicine studies is used to augment the anatomic information that is obtained by traditional imaging modalities such as x-ray, computed tomography (CT), or magnetic resonance imaging (MRI). Although these modalities provide excellent information about what the organ in question looks like, they can provide only limited information about how well the organ is working.
Nuclear medicine is also used as a form of treatment for certain diseases by taking advantage of the destructive effects of certain types of radioactive emissions to provide localized treatment of disease. The most common example of this is the use of a radioactive form of iodide for the treatment of thyroid diseases such as overactive thyroid or thyroid cancer. The thyroid gland typically takes iodide from the bloodstream to make thyroid hormones that play an important role in maintaining basic functions of the body. When a radioactive form of the iodide molecule is administered, the thyroid gland takes up the radioactive iodide molecule just like it takes up the normal iodine molecule. Once in the gland, the radioactive emissions from the molecule cause damage to the thyroid tissue. In patients with an overactive thyroid, this is enough to return function back to normal levels. In thyroid cancer, the dose is substantially higher. The goal is to completely damage all thyroid tissue, which treats the cancer and prevents disease recurrence. There are more than 100 different nuclear medicine imaging procedures available today (table 6-1), with approximately 16 million imaging and therapy procedures performed annually in the United States alone, the majority being related to cardiac and oncology applications. These procedures can be performed on any patient population, including pediatric patients.1
Figure 6–1. Nuclear Imaging of the kidney (a) An example of normal renal function—good uptake in kidney with radioactivity moving to bladder. (b) Renal scan showing good function of left kidney, with extremely poor function of right kidney due to blockage of blood flow. (c) Complete absence of renal function (no kidneys seen) in 3-day-old child with polycystic kidney disease.
Procedure | Purpose |
Bone imaging | Evaluation of metastatic cancer that has spread to bone |
Evaluation of small fractures | |
Evaluation of trauma | |
Cardiac imaging | Evaluation of myocardial perfusion (blood flow to cardiac muscle) |
Evaluation of cardiac ejection fraction (amount of blood pumped per heartbeat) | |
GI imaging | Evaluation of rate of gastric emptying |
Evaluation of presence and extent of gastric reflux | |
Liver/spleen/biliary imaging | Evaluation of liver function |
Evaluation of bile formation and excretion | |
Renal imaging | Evaluation of renal (kidney) function (GFR, ERPF) |
Evaluation of post-renal transplant function | |
Evaluation of vesicourethral reflux (reflux of urine from bladder to kidney) | |
Thyroid imaging/therapy | Evaluation of hyperthyroidism (type and severity) |
Evaluation of thyroid nodules | |
Effectiveness of treatment of hyperthyroidism and thyroid carcinoma |
Historical Perspective
The development of the specialty of nuclear pharmacy is primarily credited to Dr. John Christian, a professor at the Purdue University School of Pharmacy, and Captain William H. Briner of the National Institutes of Health (NIH). As early as 1948, Christian recognized the potential relationship between pharmacy and the use of radioactivity.2 Throughout his career in academia, Christian continued to focus on the role that pharmacy could play in the medical use of radioactivity by establishing a series of training courses at Purdue University that are still in existence today. Captain Briner established the first radiopharmacy at the NIH in 1958, and he is widely recognized in the nuclear pharmacy community as the “Father of Radiopharmacy.”2
In the 1970s, several leaders in the yet unrecognized field of nuclear pharmacy began to focus on creating formalized educational programs within several schools of pharmacy, recognizing that pharmacists with specialty training would be best prepared to provide the services needed to support the nuclear medicine community. In 1978, thanks to the work of several pioneers in the field, nuclear pharmacy was recognized by the American Pharmaceutical Association (APhA) as the first specialty area of pharmacy practice.2 Development of a board certification specialty examination (BCNP) followed, with the first exam administered in 1982.3 Currently, there are more than 500 nuclear pharmacists who have earned board certification.4
Nuclear pharmacy practice today takes many different forms. Early practitioners of nuclear pharmacy were hospital pharmacists who took an interest in the agents used in the nuclear medicine department of their institution. Hospitals that used radiopharmaceuticals were primarily academic medical centers that used their own “in-house” radiopharmacies to dispense radiopharmaceuticals to patients treated in their particular institutions.5
In the late 1970s and early 1980s, several centralized nuclear pharmacy companies were established that placed nuclear pharmacy “labs” at various locations throughout the United States. This type of nuclear pharmacy provided unit dose service to hospitals, clinics, and physicians’ offices within a localized geographic area. Unit doses are individual doses, generally dispensed in a syringe, that contain the correct amount of radioactivity for the actual time of administration to the patient.
Currently, there are three major chain nuclear pharmacies with more than 200 locations, and more than 100 independently owned nuclear pharmacies throughout the United States.6 The majority of pharmacist and technician employment opportunities are found in the centralized nuclear pharmacy practice setting.7 There are still several hospital-based pharmacies that employ nuclear pharmacists and nuclear pharmacy technicians; however, these are mostly limited to very large hospital systems or academic medical centers.
RX for Success
While PTCB training does not cover the scope of nuclear pharmacy practice at this time, many states and some nuclear pharmacy providers require PTCB certification in order for nuclear pharamacists and technicians to work in the pharmacy.
Some nuclear pharmacists choose to practice in specialized facilities that focus on the production and dispensing of a slightly different type of radiopharmaceutical agent for performing positron emission tomography (PET) imaging. PET is a type of nuclear imaging study that uses very short-lived radioactive materials.8 PET imaging has become more popular in recent years and, as a result, has led to increased numbers of dedicated facilities that specialize in this area.
Nuclear Pharmacy Operations
Stepping inside a nuclear pharmacy is a distinctly different experience than in most other types of pharmacies. In addition, nuclear pharmacy involves some unique workflow patterns that are followed on a daily basis. The pharmacy technician is an integral part of almost every aspect of nuclear pharmacy practice and an essential member of the nuclear pharmacy team. It is important to realize that the role of the pharmacy technician varies from pharmacy to pharmacy, in part based on the pharmacy regulations set forth by each state’s board of pharmacy.
RX for Success
Nuclear pharmacy technicians generally receive most of their training “on-the-job.” Having no experience in nuclear pharmacy is expected when hiring new pharmacy technicians in this area of practice.
Nuclear pharmacy is a 24-hour-a-day operation. Even though not every nuclear pharmacy is physically open all hours of the day, several nuclear medicine studies are considered emergent, and radiopharmaceuticals must be made available at any time of the day. As such, most nuclear pharmacists also have an “on-call” component to their job, during which they must be available at any time of the day or night to provide appropriate services to the end user.
The most critical component of the preparation and dispensing of radiopharmaceuticals is the presence of the radioactive material in the final preparation. The concept of radioactive decay fundamentally drives all of the processes and procedures in nuclear pharmacy operations. When a radioactive material gives off a radioactive emission, it eventually becomes nonradioactive, or stable. Because every isotope has a distinct half-life, we can predict approximately how long a product will give off enough emissions to allow imaging to take place. Radioactive decay means that the radiopharmaceutical products essentially become inactive, which creates significant logistical issues when preparing and dispensing products.
Unique aspects of nuclear pharmacy operations include
Location of nuclear pharmacies
Workflow and staffing
Restricted and nonrestricted areas
Preparation and dispensing
Location of Nuclear Pharmacies
The location of nuclear pharmacies is unique— centralized nuclear pharmacies are not located on every street corner in every city, as you might see with traditional community pharmacies. Nuclear pharmacies are generally found in larger metropolitan areas and other locations where there are several hospitals in a fairly close geographic area. Generally, because of the challenge of working with radioactivity, most pharmacies can only deliver final materials to hospitals within a twoor three-hour travel distance from the pharmacy.
The outward appearance of a nuclear pharmacy also differs from what most people expect in a pharmacy. Nuclear pharmacies are often located in strip malls, medical office complexes, and, occasionally, as freestanding buildings. In fact, there may be few outward signs that a pharmacy is located in the building. Movement into and out of nuclear pharmacies is fairly restricted due to the strict regulations involving control of radioactive materials, so it is unlikely that an individual would be able to just walk into a nuclear pharmacy off the street. Because nuclear pharmacies must deliver their products to the end users, nuclear pharmacies are often located in areas where there is ample room for a fleet of delivery vehicles to be maintained. Due to the emergent need and time sensitivity associated with radiopharmaceuticals, most nuclear pharmacies have quick access to major transportation routes (interstates, highways, etc).
Workflow and Staffing
Because nuclear pharmacies are either open or available 24 hours a day, 7 days a week, 365 days a year, there are generally several dedicated shifts. The most important and productive time in a nuclear pharmacy is the early/opening shift, also known as the “night shift.” Because radiopharmaceuticals must be compounded on a daily basis due to the radioactive component of the products, the nuclear pharmacy must prepare, dispense, and deliver agents to their customers before the first patient is seen in the nuclear medicine department. Most nuclear medicine departments begin seeing patients as early as 7 a.m., so a majority of the work done in the nuclear pharmacy is performed during the early morning shift. In pharmacies that are not open 24 hours, the early shift consists of at least one pharmacist and one technician who both begin work between midnight and 2 a.m. In larger nuclear pharmacies, there may be more than one technician who works the early shift. A majority of the doses dispensed from the pharmacy will be en route to the hospitals or clinics before 6 a.m. each day.
Customers will have “add-on” doses that will be needed throughout the day, so nuclear pharmacies also have typical day shifts, generally beginning as early as 6 a.m. to meet these needs throughout the day. Again, depending on the size of the pharmacy, there may be one or two pharmacists and pharmacy technicians working at any given time in the pharmacy. The day-time shift consists of filling add-on doses called in by customers, performing daily regulatory tasks, taking prescription orders for materials needed the next day, and setting up the pharmacy to get ready for the preparation of the next day’s doses.
Generally, if a pharmacy is not open 24 hours, the end of the workday occurs between 4 and 5 p.m. each day. Once the last pharmacist leaves the pharmacy, the pharmacist who is on-call begins coverage. Some pharmacies implement a rotating shift so that all pharmacists and technicians work every shift, whereas other pharmacies have dedicated staff working the opening shift to maintain continuity. The staffing and scheduling of a nuclear pharmacy is specific to each nuclear pharmacy and is usually established based on customer demand, workload levels, and input from the pharmacy staff.
Restricted and Nonrestricted Areas
Commercial nuclear pharmacies are often referred to as “labs” because of the way they are set up. Every nuclear pharmacy has two distinct areas: the restricted (radioactive) and nonrestricted (nonradioactive) areas. The nonrestricted areas are the general areas of the pharmacy where the staff and visitors can be assured of having no contact with radioactive materials. Examples of these areas include the kitchen and eating areas, office areas for the pharmacy manager and other staff members, bathrooms, and the general reception area. These areas are designed so there is no reason for radioactive materials to be brought into them, either intentionally or unintentionally. The nonrestricted areas are monitored on a regular basis to ensure there has been no inadvertent introduction of radioactive materials.
The restricted area is the area of the pharmacy where all radioactive materials are stored, handled, and dispensed. Because the radioactive materials are being manipulated, there is a risk that individuals might come into contact with radioactivity. Each radiopharmacy has its own specific layout to meet the daily preparation, dispensing, and storage needs of the facility, but all restricted areas of radiopharmacies contain the following:
Order entry area—All radiopharmaceutical doses are dispensed pursuant to an order from a nuclear medicine physician. A nuclear medicine technologist working at a hospital usually calls orders into the pharmacy each afternoon and at various times throughout the day. Depending on state regulations, telephone orders can be taken by pharmacists and, in some cases, pharmacy technicians. Telephone orders are entered into the pharmacy computer system, which allows for prescription labels to be generated that will accompany the patient’s dose to the hospital.
Compounding area—Because most radiopharmaceuticals are administered via the intravenous (IV) route, to ensure compliance with sterile product compounding guidelines, the agents are compounded in a laminar flow hood. These hoods are located in a fairly segregated area in the pharmacy, away from major traffic areas, yet fairly close to the areas where radioactive materials are stored for easy access. Pharmacists are primarily responsible for the compounding of radiopharmaceuticals in most nuclear pharmacies.
Dispensing area—The dispensing of unit doses is done in a laminar flow hood found in close proximity to the compounding area, within the same segregated area of the pharmacy. Pharmacy technicians are primarily responsible for taking the batch of prepared radiopharmaceutical (prepared in the compounding area) and withdrawing a designated amount of the material from the source vial into one or more patient doses.
Blood labeling area—One common procedure performed in most nuclear pharmacies is the isolation and radiolabeling of white blood cells that have been withdrawn from a patient in the hospital nuclear medicine department to assist in the detection of the body’s infection sites. The blood labeling area is usually segregated from the rest of the radiopharmacy due to the biohazard risk of handling blood products, but it still must be contained in the restricted area due to the use of radioactive materials. It is also located in a segregated area away from traffic to comply with sterile product compounding guidelines. In many pharmacies, both pharmacists and pharmacy technicians are responsible for the isolation and radiolabeling process.
Packaging and transport area—Because all doses dispensed must be delivered to the end user at a facility outside of the radiopharmacy, a large area of the pharmacy is dedicated to the safe packaging and transport of these doses. Driver/courier staff members in the pharmacy are responsible for ensuring that the dose containers are not contaminated with external radioactive material, placing the correct doses in appropriate shipping containers, completing inventory before shipping, and following the appropriate regulatory requirements for shipping radioactive materials. Technicians are sometimes involved in these activities once they have completed the dose drawing process to expedite the packaging and transport process.
Radioactive material storage area—In the restricted area, there will always be significant amounts of radioactive materials that are not in use, and these are generally stored in a designated area within the pharmacy. This area is designed to prevent the unintended release of radioactive materials into the work area, as well as to provide increased protection to the pharmacy staff.
Radioactive waste areas—One of the major benefits of a centralized nuclear pharmacy for the customer is the fact that waste materials (e.g., used syringes) can be returned to the pharmacy. Because these materials will still be radioactive for some period of time, all radioactive waste must be stored until the radioactivity decay is below a certain level. Because most nuclear medicine departments have limited space for holding this waste, the ability to ship it back to the nuclear pharmacy for storage and disposal is a major benefit of using a centralized radiopharmacy service. Once the radioactive waste (which is also biohazardous because it was most likely injected into a patient’s blood stream and may have some blood contamination) has reached appropriate levels of radioactivity, it is sent to a medical waste facility for disposal. This is a highly regulated area of the pharmacy; very often, pharmacy technicians are responsible for maintaining appropriate recordkeeping and waste disposal.
There is no mandated size for a commercial radiopharmacy’s restricted area; it generally depends on how many doses are dispensed from the facility and how much equipment and staff are needed to perform the daily tasks.
One interesting change that will occur in most commercial nuclear pharmacies relates to the recent release of the United States Pharmacopeia (USP) Chapter “<797> Sterile Product Compounding.” The USP is an official public standards-setting authority for medications, food ingredients, and dietary supplements. In response to increasing concern about the quality of sterile products (e.g., IVs and eye drops), this group released updated standards for preparation, dispensing, and release of sterile products, which became official in June of 2008. Because the primary dosage form for radiopharmaceuticals is an IV injection, the preparation and dispensing of radiopharmaceuticals falls under the scope of this document. Radiopharmaceuticals are specifically addressed, along with detailed requirements for equipment, training, appropriate attire when compounding and dispensing, and cleaning of the work and surrounding areas. Many commercial nuclear pharmacies are making significant changes to their compounding facilities to become compliant with these new recommendations.9 The general scope of USP <797> is described in other chapters of this textbook, but should be reviewed for nuclear pharmacy-specific requirements when employment is begun in a commercial nuclear pharmacy setting.
Preparation
In nuclear pharmacies, a significant amount of preparation must take place before the first product is made each day. The same general procedure for the preparation of radiopharmaceuticals is followed by most nuclear pharmacy operations, with minor deviations depending on pharmacy-specific needs.
Prescription Processing. As stated above, most prescriptions for radiopharmaceutical agents will be called in to the pharmacy the evening before they are needed. A majority of patients who are treated in nuclear medicine departments are seen on an outpatient basis and are scheduled well in advance. Most hospital facilities have add-on doses that are ordered during the nighttime hours or put on the schedule as a “STAT” (at once, immediately) procedure, but these are only a few doses each day, generally.
When a prescription for a radiopharmaceutical is taken, there are some differences when compared to traditional prescription orders. First, radiopharmaceuticals are not dispensed based on the milligram (mg) amount of the product, but instead on the amount of radioactivity that will be administered to the patient. Therefore, assays are used rather than traditional concentration values (e.g., mg/mL). An assay in nuclear pharmacy describes the number of activity units (mCi) per mL and is expressed as mCi/mL. Just like concentration values for non-radiopharmaceuticals, the assay of radiopharmaceutical products is extremely important when radioactive doses are prepared and dispensed. A challenge in the nuclear pharmacy environment is the fact that the assay of the products is constantly changing due to the continual loss of activity from radioactive decay. This is one of the most difficult concepts for new practitioners (pharmacists and technicians) in a nuclear pharmacy practice.
When a prescription order for a radiopharmaceutical is placed, the following information must be obtained:
1. agent to be administered
2. amount of radioactivity (mCi) to be dispensed to the patient
3. time of administration to the patient
4. patient name
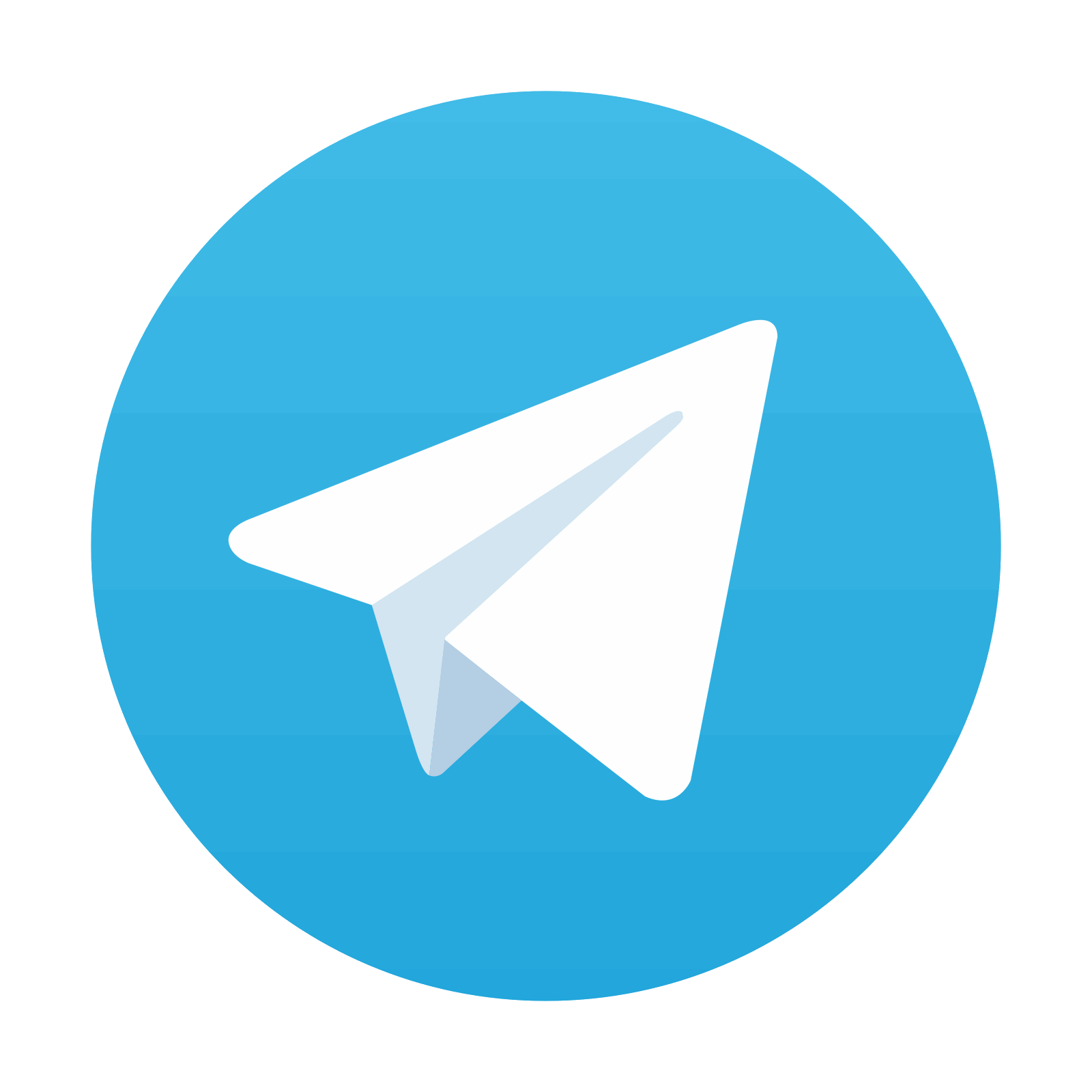