OBJECTIVES
After studying this chapter, youshould be able to:
Name the types of touch and pressure receptors found in the skin.
Describe the receptors that mediate the sensations of pain and temperature.
Define generator potential.
Explain the basic elements of sensory coding.
Explain the differences between pain and nociception, first and second pain, acute and chronic pain, hyperalgesia, and allodynia.
Describe and explain visceral and referred pain.
Compare the pathway that mediates sensory input from touch, proprioceptive, and vibratory senses to that mediating information from nociceptors and thermoreceptors.
Describe processes involved in modulation of transmission in pain pathways.
List some drugs that have been used for relief of pain and give the rationale for their use and their clinical effectiveness.
INTRODUCTION
We learn in elementary school that there are “five senses” (touch, sight, hearing, smell, and taste); but this dictum takes into account only those senses that reach our consciousness. There are many sensory receptors that relay information about the internal and external environment to the central nervous system (CNS) but do not reach consciousness. For example, the muscle spindles provide information about muscle length, and other receptors provide information about arterial blood pressure, the levels of oxygen and carbon dioxide in the blood, and the pH of the cerebrospinal fluid. The list of sensory modalities listed in Table 8–1 is overly simplified. The rods and cones, for example, respond maximally to light of different wavelengths, and three types of cones are present, one for each of the three primary colors. There are five modalities of taste: sweet, salt, sour, bitter, and umami. Sounds of different pitches are heard primarily because different groups of hair cells in the cochlea are activated maximally by sound waves of different frequencies.
Sensory System | Modality | Stimulus | Receptor Class | Receptor Cell Types |
---|---|---|---|---|
Somatosensory | Touch | Tap, flutter 5–40 Hz | Cutaneous mechanoreceptor | Meissner corpuscles |
Somatosensory | Touch | Motion | Cutaneous mechanoreceptor | Hair follicle receptors |
Somatosensory | Touch | Deep pressure, vibration 60–300 Hz | Cutaneous mechanoreceptor | Pacinian corpuscles |
Somatosensory | Touch | Touch, pressure | Cutaneous mechanoreceptor | Merkel cells |
Somatosensory | Touch | Sustained pressure | Cutaneous mechanoreceptor | Ruffini corpuscles |
Somatosensory | Proprioception | Stretch | Mechanoreceptor | Muscle spindles |
Somatosensory | Proprioception | Tension | Mechanoreceptor | Golgi tendon organ |
Somatosensory | Temperature | Thermal | Thermoreceptor | Cold and warm receptors |
Somatosensory | Pain | Chemical, thermal, and mechanical | Chemoreceptor, thermoreceptor, and mechanoreceptor | Polymodal receptors or chemical, thermal, and mechanical nociceptors |
Somatosensory | Itch | Chemical | Chemoreceptor | Chemical nociceptor |
Visual | Vision | Light | Photoreceptor | Rods, cones |
Auditory | Hearing | Sound | Mechanoreceptor | Hair cells (cochlea) |
Vestibular | Balance | Angular acceleration | Mechanoreceptor | Hair cells (semicircular canals) |
Vestibular | Balance | Linear acceleration, gravity | Mechanoreceptor | Hair cells (otolith organs) |
Olfactory | Smell | Chemical | Chemoreceptor | Olfactory sensory neuron |
Gustatory | Taste | Chemical | Chemoreceptor | Taste buds |
Sensory receptors can be thought of as transducers that convert various forms of energy in the environment into action potentials in sensory neurons. The cutaneous receptors for touch and pressure are mechanoreceptors. Proprioceptors are located in muscles, tendons, and joints and relay information about muscle length and tension. Thermoreceptors detect the sensations of warmth and cold. Potentially harmful stimuli such as pain, extreme heat, and extreme cold are mediated by nociceptors. The term chemoreceptor refers to receptors stimulated by a change in the chemical composition of the environment in which they are located. These include receptors for taste and smell as well as visceral receptors such as those sensitive to changes in the plasma level of O2, pH, and osmolality. Photoreceptors are those in the rods and cones in the retina that respond to light.
This chapter describes primarily the characteristics of cutaneous receptors that mediate the sensations of touch, pressure, pain, and temperature; the way they generate impulses in afferent neurons; and the central pathways that mediate or modulate information from these receptors. Since pain is one of the main reasons an individual seeks the advice of a clinician, this topic gets considerable attention in this chapter. Receptors involved in the somatosensory modality of proprioception are described in Chapter 12 as they play key roles in the control of balance, posture, and limb movement.
SENSE RECEPTORS & SENSE ORGANS
Sensory receptors can be specialized dendritic endings of afferent nerve fibers, and they are often associated with nonneural cells that surround them forming a sense organ. Touch and pressure are sensed by four types of mechanoreceptors (Figure 8–1). Meissner corpuscles are dendrites encapsulated in connective tissue and respond to changes in texture and slow vibrations. Merkel cells are expanded dendritic endings, and they respond to sustained pressure and touch. Ruffini corpuscles are enlarged dendritic endings with elongated capsules, and they respond to sustained pressure. Pacinian corpuscles consist of unmyelinated dendritic endings of a sensory nerve fiber, 2 μm in diameter, encapsulated by concentric lamellae of connective tissue that give the organ the appearance of a cocktail onion. These receptors respond to deep pressure and fast vibration. The sensory nerves from these mechanoreceptors are large myelinated Aα and Aβ fibers whose conduction velocities range from ~70–120 to ~40–75 m/s, respectively.
FIGURE 8–1
Sensory systems encode four elementary attributes of stimuli: modality, location (receptive field), intensity, and duration (timing). A) The human hand has four types of mechanoreceptors; their combined activation produces the sensation of contact with an object. Selective activation of Merkel cells and Ruffini endings causes sensation of steady pressure; selective activation of Meissner and Pacinian corpuscles causes tingling and vibratory sensation. B) Location of a stimulus is encoded by spatial distribution of the population of receptors activated. A receptor fires only when the skin close to its sensory terminals is touched. These receptive fields of mechanoreceptors (shown as red areas on fingertips) differ in size and response to touch. Merkel cells and Meissner corpuscles provide the most precise localization as they have the smallest receptive fields and are most sensitive to pressure applied by a small probe. C) Stimulus intensity is signaled byfiring rates of individual receptors; duration of stimulus is signaled by time course of firing. The spike trains indicate action potentials elicited by pressure from a small probe at the center of each receptive field. Meissner and Pacinian corpuscles adapt rapidly, the others adapt slowly. (Reproduced with permission from Kandel ER, Schwartz JH, Jessell TM [editors]: Principles of Neural Science, 4th ed. New York, NY: McGraw-Hill; 2000.)
Some cutaneous sensory receptors are not specialized organs but rather they are free nerve endings. Pain and temperature sensations arise from unmyelinated dendrites of sensory neurons located throughout the glabrous and hairy skin as well as deep tissue. Nociceptors can be separated into several types. Mechanical nociceptors respond to strong pressure (eg, from a sharp object). Thermal nociceptors are activated by skin temperatures above 42°C or by severe cold. Chemically sensitive nociceptors respond to various chemicals such as bradykinin, histamine, high acidity, and environmental irritants. Polymodal nociceptors respond to combinations of these stimuli.
Impulses from nociceptors are transmitted via two fiber types, thinly myelinated Aδ fibers (2–5 μm in diameter) that conduct at rates of ~12–35 m/s and unmyelinated C fibers (0.4–1.2 μm in diameter) that conduct at low rates of ~0.5–2 m/s. Activation of Aδ fibers, which release glutamate, is responsible for first pain (also called fast pain or epicritic pain), which is a rapid response and mediates the discriminative aspect of pain or the ability to localize the site and intensity of the noxious stimulus. Activation of C fibers, which release a combination of glutamate and substance P, is responsible for the delayed second pain (also called slow pain or protopathic pain), which is the dull, intense, diffuse, and unpleasant feeling associated with a noxious stimulus. Itch and tickle are also related to pain sensation (see Clinical Box 8–1).
There are a variety of receptors located on the endings of nociceptive sensory nerves that respond to noxious thermal, mechanical, or chemical stimuli (Figure 8–2). Many of these are part of a family of nonselective cation channels called transient receptor potential (TRP) channels. This includes TRPV1 receptors (the V refers to a group of chemicals called vanilloids) that are activated by intense heat, acids, and chemicals such as capsaicin (the active principle of hot peppers and an example of a vanilloid). TRPV1 receptors can also be activated indirectly by initial activation of TRPV3 receptors in keratinocytes in the skin. Noxious mechanical, cold, and chemical stimuli may activate TRPA1 receptors (A, for ankyrin) on sensory nerve terminals. Sensory nerve endings also have acid-sensing ion channel (ASIC) receptors that are activated by pH changes within a physiologic range and may be the dominant receptors mediating acid-induced pain. In addition to direct activation of receptors on nerve endings, some nociceptive stimuli release intermediate molecules that then activate receptors on the nerve ending. For example, nociceptive mechanical stimuli cause the release of adenosine triphosphate (ATP) that acts on purinergic receptors (eg, P2X, an ionotropic receptor, and P2Y, a G-protein–coupled receptor). Tyrosine receptor kinase A (TrkA) is activated by nerve growth factor (NGF) that is released as a result of tissue damage.
FIGURE 8–2
Receptors on nociceptive unmyelinated nerve terminals in the skin. Nociceptive stimuli (eg, heat) can activate some receptors directly due to transduction of the stimulus energy by receptors (eg, transient receptor potential (TRP) channel TRPV1) or indirectly by activation of TRP channels on keratinocytes (eg, TRPV3). Nociceptors (eg, mechanoreceptors) can also be activated by the release of intermediate molecules (eg, ATP). ASIC, acid-sensitive ion channel; P2X, ionotropic purinoceptor; P2Y, G-protein–coupled purinergic receptor.
CLINICAL BOX 8–1 Itch & Tickle
Itching (pruritus) is not a major problem for healthy individuals, but severe itching that is difficult to treat occurs in diseases such as chronic kidney disease, some forms of liver disease, atopic dermatitis, and HIV infection. Especially in areas where many free endings of unmyelinated nerve fibers occur, itch spots can be identified on the skin by careful mapping. In addition, itch-specific fibers have been demonstrated in the ventrolateral spinothalamic tract. This and other evidence implicate the existence of an itch-specific path. Relatively mild stimulation, especially if produced by something that moves across the skin, produces itch and tickle. It is interesting that a tickling sensation is usually regarded as pleasurable, whereas itching is annoying and pain is unpleasant. Itching can be produced not only by repeated local mechanical stimulation of the skin but also by a variety of chemical agents, including histamine and kinins such as bradykinin, that are released in the skin in response to tissue damage. Kinins exert their effects by activation of two types of G-protein–coupled receptors, B1 and B2. Activation of bradykinin B2 receptors is a downstream event in protease-activated receptor-2 (PAR-2) activation, which induces both a nociceptive and a pruritogenic response.
THERAPEUTIC HIGHLIGHTSSimple scratching relieves itching because it activates large, fast-conducting afferents that gate transmission in the dorsal horn in a manner analogous to the inhibition of pain by stimulation of similar afferents. Antihistamines are primarily effective in reducing pruritus associated with an allergic reaction. In a mouse model exhibiting scratching behavior in response to activation of PAR-2, treatment with a B2 receptor antagonist reduced the scratching behavior. B2 receptor antagonists may be a useful therapy for treating pruriginous conditions.
Nerve endings also have a variety of receptors that respond to immune mediators that are released in response to tissue injury. These include B1 and B2 receptors (bradykinin), prostanoid receptors (prostaglandins), and cytokine receptors (interleukins). These receptors mediate inflammatory pain.
Innocuous cold receptors or cool receptors are on dendritic endings of Aδ and C fibers, whereas innocuous warmth receptors are on C fibers. Mapping experiments show that the skin has discrete cold and heat-sensitive spots. There are 4–10 times as many cold-sensitive as heat-sensitive spots.
The threshold for activation of warmth receptors is 30°C, and they increase their firing rate as the skin temperature increases to 46°C. Cold receptors are inactive at temperatures of 40°C, but then steadily increase their firing rate as skin temperature falls to about 24°C. As skin temperature further decreases, the firing rate of cold receptors decreases until the temperature reaches 10°C. Below that temperature, they are inactive and cold becomes an effective local anesthetic.
The receptor that is activated by moderate cold is TRPM8. The M refers to menthol, the ingredient in mint that gives it its “cool” taste. TRPV4 receptors are activated by warm temperatures up to 34°C; TRPV3 receptors respond to slightly higher temperatures of 35–39°C.
GENERATION OF IMPULSES IN CUTANEOUS RECEPTORS
The way that sensory receptors generate action potentials in the nerves that innervate them varies based on the complexity of the sense organ. In the skin, the Pacinian corpuscle has been studied in some detail. The myelin sheath of the sensory nerve begins inside the corpuscle (Figure 8–3). The first node of Ranvier is also located inside; the second is usually near the point at which the nerve fiber leaves the corpuscle.
FIGURE 8–3
Demonstration that the generator potential in a Pacinian corpuscle originates in the unmyelinated nerve terminal. The electrical responses to pressures (black arrow) of 1, 2×, 3×, and 4× are shown. The strongest stimulus produced an action potential in the sensory nerve, originating in the center of the corpuscle. (Reproduced with permission from Waxman SG: Clinical Neuroanatomy, 26th ed. New York, NY: McGraw-Hill; 2010.)
When a small amount of pressure is applied to the Pacinian corpuscle, a nonpropagated depolarizing potential resembling an excitatory postsynaptic potential (EPSP) is recorded. This is called the generator potential or receptor potential (Figure 8–3). As the pressure is increased, the magnitude of the receptor potential is increased. The receptor therefore converts mechanical energy into an electrical response, the magnitude of which is proportional to the intensity of the stimulus. Thus, the responses are described as graded potentials rather than all-or-none as is the case for an action potential. When the magnitude of the generator potential reaches about 10 mV, an action potential is produced at the first node of Ranvier. The nerve then repolarizes. If the generator potential is great enough, the neuron fires again as soon as it repolarizes, and it continues to fire as long as the generator potential is large enough to bring the membrane potential of the node to the firing level. Thus, the node converts the graded response of the receptor into action potentials, the frequency of which is proportional to the magnitude of the applied stimulus.
SENSORY CODING
Converting a receptor stimulus to a recognizable sensation is termed sensory coding. All sensory systems code for four elementary attributes of a stimulus: modality, location, intensity, and duration. Modality is the type of energy transmitted by the stimulus. Location is the site on the body or space where the stimulus originated. Intensity is signaled by the response amplitude or frequency of action potential generation. Duration refers to the time from start to end of a response in the receptor. These attributes of sensory coding are shown for the modality of touch in Figure 8–1.
When the nerve from a particular sensory receptor is stimulated, the sensation evoked is that for which the receptor is specialized no matter how or where along the nerve the activity is initiated. This principle, first enunciated by Johannes Müller in 1835, has been called the law of specific nerve energies. For example, if the sensory nerve from a Pacinian corpuscle in the hand is stimulated by pressure at the elbow or by irritation from a tumor in the brachial plexus, the sensation evoked is touch. The general principle of specific nerve energies remains one of the cornerstones of sensory physiology.
Humans have four basic classes of receptors based on their sensitivity to one predominant form of energy: mechanical, thermal, electromagnetic, or chemical. The particular form of energy to which a receptor is most sensitive is called its adequate stimulus. The adequate stimulus for the rods and cones in the eye, for example, is light (an example of electromagnetic energy). Receptors do respond to forms of energy other than their adequate stimuli, but the threshold for these nonspecific responses is much higher. Pressure on the eyeball will stimulate the rods and cones, for example, but the threshold of these receptors to pressure is much higher than the threshold of the pressure receptors in the skin.
The term sensory unit refers to a single sensory axon and all of its peripheral branches. These branches vary in number but may be numerous, especially in the cutaneous senses. The receptive field of a sensory unit is the spatial distribution from which a stimulus produces a response in that unit (Figure 8–1). Representation of the senses in the skin is punctate. If the skin is carefully mapped, millimeter by millimeter, with a fine hair, a sensation of touch is evoked from spots overlying these touch receptors. None is evoked from the intervening areas. Similarly, temperature sensations and pain are produced by stimulation of the skin only over the spots where the receptors for these modalities are located. In the cornea and adjacent sclera of the eye, the surface area supplied by a single sensory unit is 50–200 mm2. The area supplied by one sensory unit usually overlaps and interdigitates with the areas supplied by others.
One of the most important mechanisms that enable localization of a stimulus site is lateral inhibition. Information from sensory neurons whose receptors are at the peripheral edge of the stimulus is inhibited compared to information from the sensory neurons at the center of the stimulus. Thus, lateral inhibition enhances the contrast between the center and periphery of a stimulated area and increases the ability of the brain to localize a sensory input. Lateral inhibition underlies two-point discrimination (see Clinical Box 8–2).
The intensity of sensation is determined by the amplitude of the stimulus applied to the receptor. This is illustrated in Figure 8–4. As a greater pressure is applied to the skin, the receptor potential in the mechanoreceptor increases (not shown), and the frequency of the action potentials in a single axon transmitting information to the CNS is also increased. In addition to increasing the firing rate in a single axon, the greater intensity of stimulation also will recruit more receptors into the receptive field.
FIGURE 8–4
Relationship between stimulus and impulse frequency in an afferent fiber. Action potentials in an afferent fiber from a mechanoreceptor of a single sensory unit increase in frequency as branches of the afferent neuron are stimulated by pressure of increasing magnitude. (Reproduced with permission from Widmaier EP, Raff H, Strang KT: Vanders Human Physiology. 11th edition. New York, NY: McGraw-Hill; 2008.)
CLINICAL BOX 8–2 Neurologic Exam
The size of the receptive fields for light touch can be measured by the two-point threshold test. In this procedure, the two points on a pair of calipers are simultaneously positioned on the skin and one determines the minimum distance between the two caliper points that can be perceived as separate points of stimulation. This is called the two-point discrimination threshold.
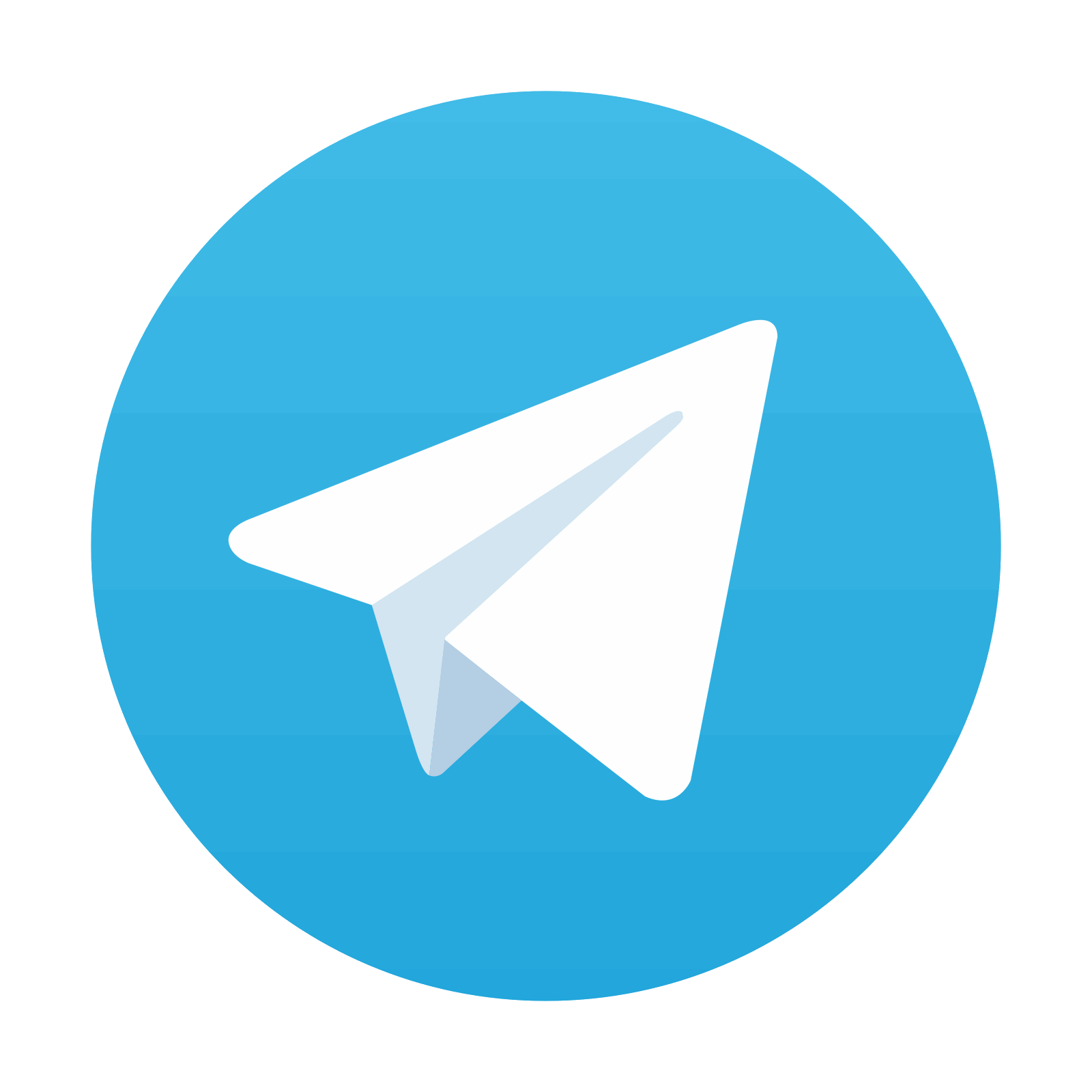
Stay updated, free articles. Join our Telegram channel

Full access? Get Clinical Tree
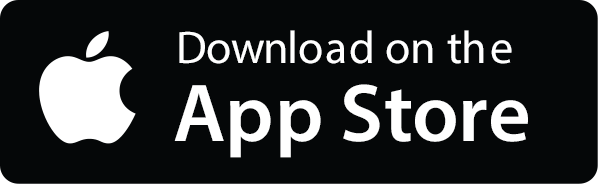
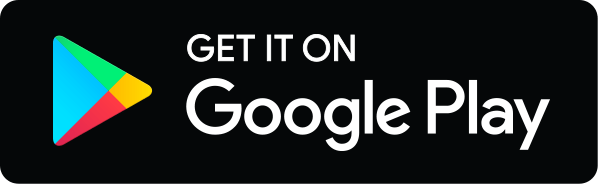
