OBJECTIVES
After studying this chapter, you should be able to:
Describe the major functions of the liver with respect to metabolism, detoxification, and excretion of hydrophobic substances.
Understand the functional anatomy of the liver and the relative arrangements of hepatocytes, cholangiocytes, endothelial cells, and Kupffer cells.
Define the characteristics of the hepatic circulation and its role in subserving the liver’s functions.
Identify the plasma proteins that are synthesized by the liver.
Describe the formation of bile, its constituents, and its role in the excretion of cholesterol and bilirubin.
Outline the mechanisms by which the liver contributes to whole body ammonia homeostasis and the consequences of the failure of these mechanisms, particularly for brain function.
Identify the mechanisms that permit normal functioning of the gallbladder and the basis of gallstone disease.
INTRODUCTION
The liver is the largest gland in the body. It is essential for life because it conducts a vast array of biochemical and metabolic functions, including ridding the body of substances that would otherwise be injurious if allowed to accumulate, and excreting drug metabolites. It is also the first port of call for most nutrients absorbed across the gut wall, supplies most of the plasma proteins, and synthesizes the bile that optimizes the absorption of fats as well as serving as an excretory fluid. The liver and associated biliary system have therefore evolved an array of structural and physiologic features that underpin this broad range of critical functions.
THE LIVER
An important function of the liver is to serve as a filter between the blood coming from the gastrointestinal tract and the blood in the rest of the body. Blood from the intestines and other viscera reach the liver via the portal vein. This blood percolates in sinusoids between plates of hepatic cells and eventually drains into the hepatic veins, which enter the inferior vena cava. During its passage through the hepatic plates, it is extensively modified chemically. Bile is formed on the other side at each plate. The bile passes to the intestine via the hepatic duct (Figure 28–1).
FIGURE 28–1
Schematic anatomy of the liver. Hepatocytes are arranged radially in plates surrounding a central vein. Blood is supplied to the liver by branches of the portal vein (PV) and hepatic artery (HA), which empty into sinusoids (S) surrounding the hepatocytes. The direction of blood flow is indicated with black arrows. The endothelial cells that line the sinusoids are fenestrated and thus provide little hindrance to the transfer of substances from the sinusoids to the space of Disse, which abuts the basolateral membrane of the hepatocytes. The apical membranes of adjacent hepatocytes form bile canaliculi, which transfer bile to the bile ducts lined by cholangiocytes. Bile flows in the opposite direction to blood (green arrows). The bile duct, portal vein, and hepatic artery comprise the “portal triad.” (Adapted with permission from Paulsen DF: Histology and Cell Biology: Examination and Board Review. 5th edition. New York, NY: McGraw-Hill; 2010.)
In each hepatic lobule, the plates of hepatic cells are usually only one cell thick. Large gaps occur between the endothelial cells, and plasma is in intimate contact with the cells (Figure 28–2). Hepatic artery blood also enters the sinusoids. The central veins coalesce to form the hepatic veins, which drain into the inferior vena cava. The average transit time for blood across the liver lobule from the portal venule to the central hepatic vein is about 8.4 s. Additional details of the features of the hepatic microcirculation and macrocirculation, which are critical to organ function, are provided below. Numerous macrophages (Kupffer cells) are anchored to the endothelium of the sinusoids and project into the lumen. The functions of these phagocytic cells are discussed in Chapter 3.
Each liver cell is also opposed to several bile canaliculi (Figure 28–2). The canaliculi drain into intralobular bile ducts, and these coalesce via interlobular bile ducts to form the right and left hepatic ducts. These ducts join outside the liver to form the common hepatic duct. The cystic duct drains the gallbladder. The hepatic duct unites with the cystic duct to form the common bile duct (Figure 28–1). The common bile duct enters the duodenum at the duodenal papilla. Its orifice is surrounded by the sphincter of Oddi, and it usually unites with the main pancreatic duct just before entering the duodenum. The sphincter is usually closed, but when the gastric contents enter the duodenum, cholecystokinin (CCK) is released and the gastrointestinal hormone relaxes the sphincter and makes the gallbladder contract.
The walls of the extrahepatic biliary ducts and the gallbladder contain fibrous tissue and smooth muscle. They are lined by a layer of columnar cells with scattered mucous glands. In the gallbladder, the surface is extensively folded; this increases its surface area and gives the interior of the gallbladder a honeycombed appearance. The cystic duct is also folded to form the so-called spiral valves. This arrangement is believed to increase the turbulence of bile as it flows out of the gallbladder, thereby reducing the risk that it will precipitate and form gallstones.
Large gaps occur between endothelial cells in the walls of hepatic sinusoids, and the sinusoids are highly permeable. The way the intrahepatic branches of the hepatic artery and portal vein converge on the sinusoids and drain into the central lobular veins of the liver is shown in Figure 28–1. The functional unit of the liver is the acinus. Each acinus is at the end of a vascular stalk containing terminal branches of portal veins, hepatic arteries, and bile ducts. Blood flows from the center of this functional unit to the terminal branches of the hepatic veins at the periphery (Figure 28–3). This is why the central portion of the acinus, sometimes called zone 1, is well oxygenated, the intermediate zone (zone 2) is moderately well oxygenated, and the peripheral zone (zone 3) is least well oxygenated and most susceptible to anoxic injury. The hepatic veins drain into the inferior vena cava. The acini have been likened to grapes or berries, each on a vascular stem. The human liver contains about 100,000 acini.
FIGURE 28–3
Concept of the acinus as the functional unit of the liver. In each acinus, blood in the portal venule and hepatic arteriole enters the center of the acinus and flows outward to the hepatic venule. (Adapted with permission from Rappaport AM: The microcirculatory hepatic unit, Microvasc Res 1973 Sep; 6(2):212–228.)
Portal venous pressure is normally about 10 mm Hg in humans, and hepatic venous pressure is approximately 5 mm Hg. The mean pressure in the hepatic artery branches that converge on the sinusoids is about 90 mm Hg, but the pressure in the sinusoids is lower than the portal venous pressure, so a marked pressure drop occurs along the hepatic arterioles. This pressure drop is adjusted so that there is an inverse relationship between hepatic arterial and portal venous blood flow. This inverse relationship may be maintained in part by the rate at which adenosine is removed from the region around the arterioles. According to this hypothesis, adenosine is produced by metabolism at a constant rate. When portal flow is reduced, it is washed away more slowly, and the local accumulation of adenosine dilates the terminal arterioles. In the period between meals, moreover, many of the sinusoids are collapsed. Following a meal, on the other hand, when portal flow to the liver from the intestine increases considerably, these “reserve” sinusoids are recruited. This arrangement means that portal pressures do not increase linearly with portal flow until all sinusoids have been recruited. This may be important to prevent fluid loss from the highly permeable liver under normal conditions. Indeed, if hepatic pressures are increased in disease states (such as the hardening of the liver that is seen in cirrhosis), many liters of fluid can accumulate in the peritoneal cavity as ascites.
The intrahepatic portal vein radicles have smooth muscle in their walls that is innervated by noradrenergic vasoconstrictor nerve fibers reaching the liver via the 3rd to 11th thoracic ventral roots and the splanchnic nerves. The vasoconstrictor innervation of the hepatic artery comes from the hepatic sympathetic plexus. No known vasodilator fibers reach the liver. When systemic venous pressure rises, the portal vein radicles are dilated passively and the amount of blood in the liver increases. In heart failure, this hepatic venous congestion may be extreme. Conversely, when diffuse noradrenergic discharge occurs in response to a drop in systemic blood pressure, the intrahepatic portal radicles constrict, portal pressure rises, and blood flow through the liver is brisk, bypassing most of the organ. Most of the blood in the liver enters the systemic circulation. Constriction of the hepatic arterioles diverts blood from the liver, and constriction of the mesenteric arterioles reduces portal inflow. In severe shock, hepatic blood flow may be reduced to such a degree that patchy necrosis of the liver takes place.
FUNCTIONS OF THE LIVER
The liver has many complex functions that are summarized in Table 28–1. Several will be touched upon briefly here.
Formation and secretion of bile |
Nutrient and vitamin metabolism |
Glucose and other sugars Amino acids Lipids Fatty acids Cholesterol Lipoproteins Fat-soluble vitamins Water-soluble vitamins |
Inactivation of various substances |
Toxins Steroids Other hormones |
Synthesis of plasma proteins |
Acute-phase proteins Albumin Clotting factors Steroid-binding and other hormone-binding proteins |
Immunity Kupffer cells |
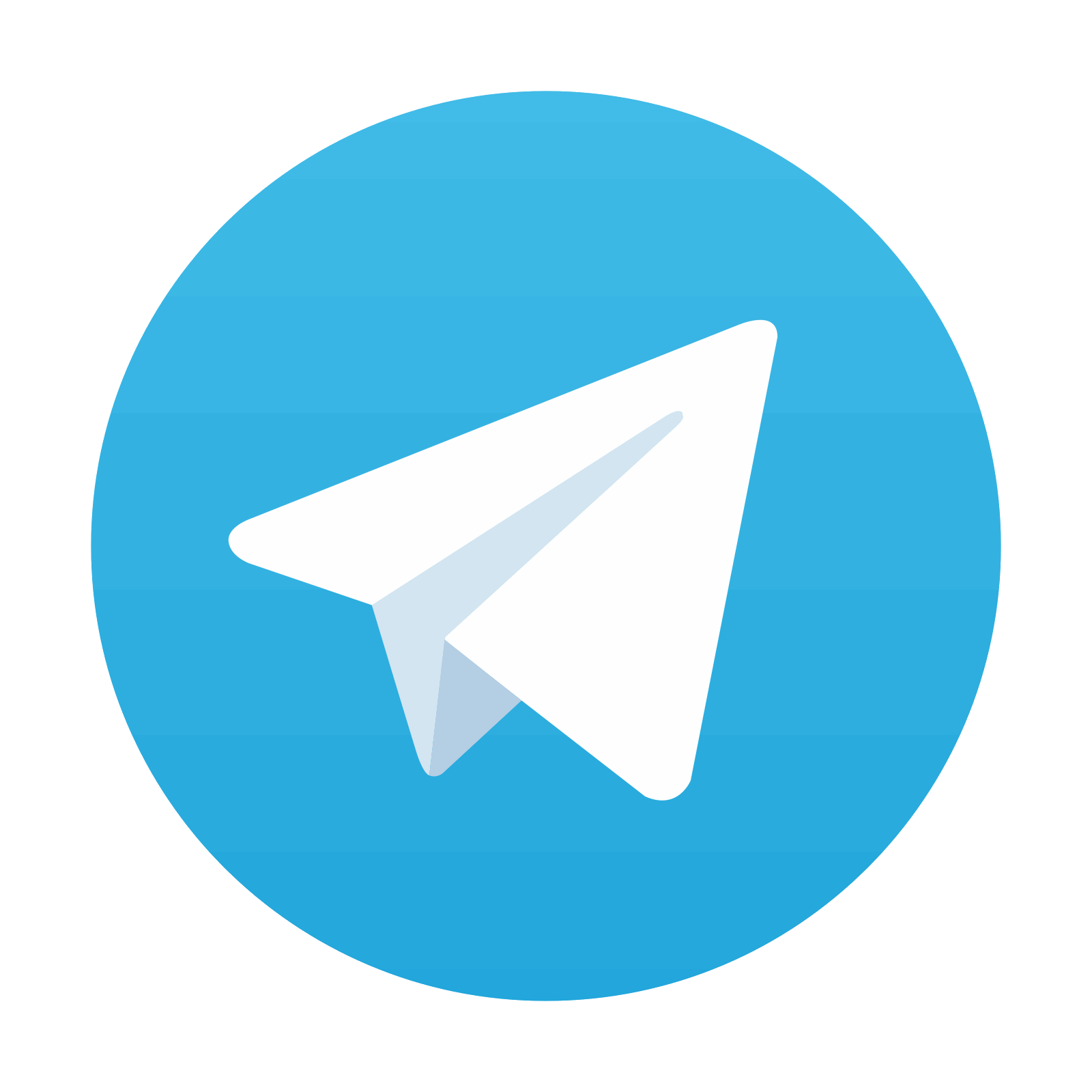
Stay updated, free articles. Join our Telegram channel

Full access? Get Clinical Tree
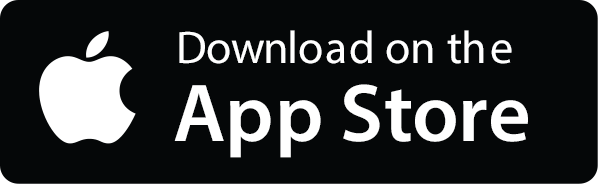
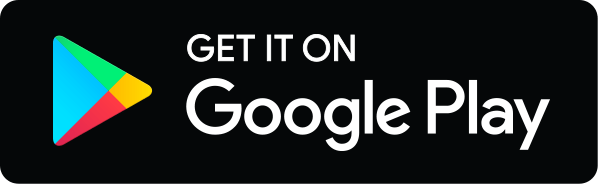