CHAPTER 14 Smooth Muscle
Nonstriated, or smooth, muscle cells are a major component of hollow organs such as the alimentary canal, airways, vasculature, and urogenital tract. Contraction of smooth muscle serves to alter the dimensions of the organ, which may result in either propelling the contents of the organ (as in peristalsis of the intestine) or increasing the resistance to flow (as in vasoconstriction). The basic mechanism underlying contraction of smooth muscle involves an interaction of myosin with actin (as in striated muscle), although there are some important differences. Specifically, contraction of smooth muscle is thick filament regulated and requires an alteration in myosin before it can interact with actin, whereas contraction of striated muscle is thin filament regulated and requires movement of the troponin-tropomyosin complex on the actin filament before myosin can bind to actin. Smooth muscle can contract in response to either electrical or hormonal signals and exhibits the ability to remain contracted for extended periods at low levels of energy consumption, which is important for functions such as maintaining vascular tone and hence blood pressure. An additional feature of smooth muscle (termed “length adaptation”) facilitates contraction of smooth muscle over a broad range of lengths, which may be important for emptying a hollow organ at various levels of filling. Thus, regulation of contraction of smooth muscle is complex, sometimes involving multiple intracellular signaling cascades. In the present chapter, effort is made to identify mechanisms underlying this diverse regulation of smooth muscle contraction and, when appropriate, compare these regulatory mechanisms with those observed in striated muscle. Alterations in smooth muscle function/regulation that have been implicated in various pathological conditions are also discussed.
OVERVIEW OF SMOOTH MUSCLE
Types of Smooth Muscle
A second consideration when discussing types of smooth muscle is the activity pattern (Fig. 14-1). In some organs, the smooth muscle cells contract rhythmically or intermittently, whereas in other organs, the smooth muscle cells are continuously active and maintain a level of “tone.” Smooth muscle exhibiting rhythmic or intermittent activity is termed phasic smooth muscle and includes smooth muscles in the walls of the gastrointestinal and urogenital tracts. Such phasic smooth muscle corresponds to the single-unit category described earlier because the smooth muscle cells contract in response to action potentials that propagate from cell to cell. Smooth muscle that is continuously active, on the other hand, is termed tonic smooth muscle. Vascular smooth muscle, respiratory smooth muscle, and some sphincters are continuously active. The continuous partial activation of tonic smooth muscle is not associated with action potentials, although it is proportional to membrane potential. Tonic smooth muscle would thus correspond to the multiunit smooth muscle described earlier. Phasic and tonic contractions of smooth muscle result from interactions of actin and myosin filaments, although as discussed later in this chapter, there is a change in cross-bridge cycling kinetics during tonic contraction such that the smooth muscle can maintain force at low energy cost.
STRUCTURE OF SMOOTH MUSCLE CELLS
Smooth muscle cells typically form layers around hollow organs (Fig. 14-2). Blood vessels and airways exhibit a simple tubular structure in which the smooth muscle cells are arranged circumferentially, so contraction reduces the diameter of the tube. This contraction increases resistance to the flow of blood or air but has little effect on the length of the organ. Smooth muscle cell organization is more complex in the gastrointestinal tract. Layers of smooth muscle in both circumferential and longitudinal orientations provide the mechanical action for mixing food and also propelling the luminal contents from the mouth to the anus. Coordination between these layers depends on a complex system of autonomic nerves linked by plexuses. These plexuses are located between the two muscle layers. The smooth muscle in the walls of saccular structures, such as the urinary bladder or rectum, allows the organ to increase in size with the accumulation of urine or feces. The varied arrangement of cells in the walls of these organs contributes to their ability to reduce internal volume to almost zero during urination or defecation. Smooth muscle cells in hollow organs occur in a spectrum of forms, depending on their function and mechanical loads.
Cell-to-Cell Contact
A variety of specialized contact exists between smooth muscle cells. Such contact allows mechanical linkage and communication between the cells (Fig. 14-3). In contrast to skeletal muscle cells, which are normally attached at either end to a tendon, smooth (and cardiac) muscle cells are connected to each other. Because smooth muscle cells are anatomically arranged in series, they not only must be mechanically linked but must also be activated simultaneously and to the same degree. This mechanical and functional linkage is crucial to smooth muscle function. If such linkage did not exist, contraction in one region would simply stretch another region without a substantial decrease in radius or increase in pressure. The mechanical connections are provided by attachments to sheaths of connective tissue and by specific junctions between muscle cells.
Several types of junctions are found in smooth muscle (Fig. 14-4). Functional linkage of the cells is provided by gap junctions. Gap junctions form low-resistance pathways between cells (see Chapter 2). They also allow chemical communication by diffusion of low-molecular-weight compounds. In certain tissues, such as the outer longitudinal layer of smooth muscle in the intestine, large numbers of such junctions exist. Action potentials are readily propagated from cell to cell through such tissues.
Adherens junctions (also called dense plaques or attachment plaques) provide mechanical linkage between smooth muscle cells. As depicted in Figure 14-4, the adherens junction appears as thickened regions of opposing cell membranes that are separated by a small gap (≈60 nm) containing dense granular material. Thin filaments extend into the adherens junction to allow the contractile force generated in one smooth muscle cell to be transmitted to adjacent smooth muscle cells.
Figure 14-3 also shows the presence of caveolae, which represent invaginations of the smooth muscle membrane (analogous to T tubules in striated muscle). The sarcoplasmic reticulum (SR) extends throughout the smooth muscle cell, although as depicted in Figure 14-3, there are junctional regions of the SR where it abuts regions of the sarcolemma or caveolae, or both. As discussed in a subsequent section, these subsarcolemmal regions of the SR play an important role in the regulation of intracellular [Ca++] and hence smooth muscle tone.
Cells and Membranes
Embryonic smooth muscle cells do not fuse, and each differentiated cell has a single, centrally located nucleus (Fig. 14-5). Though dwarfed by skeletal muscle cells, smooth muscle cells are nevertheless quite large (typically 40 to 600 μm long). These cells are 2 to 10 μm in diameter in the region of the nucleus, and most taper toward their ends. Contracting cells become quite distorted as a result of the force exerted on the cell by attachments to other cells or to the extracellular matrix, and cross sections of these cells are often very irregular.
Smooth muscle cells lack T tubules, the invaginations of the skeletal muscle sarcolemma that provide electrical links to the SR. However, the sarcolemma of smooth muscle has longitudinal rows of tiny sac-like in-pocketings called caveolae (Figs. 14-3 and 14-5). Caveolae increase the surface-to-volume ratio of the cells and are often closely opposed to the underlying SR. A gap of approximately 15 nm has been observed between the caveolae and the underlying SR, comparable to the gap between the T tubules and terminal SR in skeletal muscle. Moreover, “Ca++ sparks” and a variety of Ca++-handling proteins have been observed in the vicinity of caveolae, thus raising the possibility that the caveolae and the underlying SR may contribute to the regulation of intracellular [Ca++] in smooth muscle. The voltage-gated L-type Ca++ channel and the 3Na+-1Ca++ antiporter, for example, are associated with caveolae. The proteins caveolin and cholesterol are both critical for the formation of caveolae, and it is hypothesized that the caveolae reflect a specialized region of the sarcolemma that may also contain various signaling molecules in addition to the Ca++ signaling mentioned earlier.
Smooth muscle also has an intracellular membrane network of SR that serves as an intracellular reservoir for Ca++ (Figs. 14-3 and 14-5). Calcium can be released from the SR into the myoplasm when stimulatory neurotransmitters, hormones, or drugs bind to receptors on the sarcolemma. Importantly, intracellular Ca++ channels in the SR of smooth muscle include the ryanodine receptor (RYR), which is similar to that found in skeletal muscle SR, and the inositol 1,4,5trisphosphate (InsP3)-gated Ca++ channel. The RYR is typically activated by a rise in intracellular [Ca++] (i.e., Ca++-induced release of Ca++ in response to an influx of Ca++ through the sarcolemma). The InsP3-gated Ca++ channel is activated by InsP3, which is produced when a hormone or hormones bind to various Ca++-mobilizing receptors on the sarcolemma. Intracellular [Ca++] is lowered through the action of an SR Ca++–ATPase (SERCA) and extrusion of Ca++ from the cell via a 3Na+-1Ca++ antiporter and a sarcolemmal Ca++-ATPase. The amount of SR in smooth muscle cells varies from 2% to 6% of cell volume and approximates that of skeletal muscle. As mentioned earlier, chemical signals such as InsP3 or a localized increase in intracellular [Ca++] (e.g., within the gap between the caveolae and SR) functionally link the sarcolemma and the SR.
Smooth muscle cells contain a prominent rough endoplasmic reticulum and Golgi apparatus, which are located centrally at each end of the nucleus. These structures reflect significant protein synthetic and secretory functions. The scattered mitochondria (Fig. 14-5) are sufficient for oxidative phosphorylation to generate the increased ATP consumed during contraction.
Contractile Apparatus
The thick and thin filaments of smooth muscle cells are about 10,000 times longer than their diameter and are tightly packed. Therefore, the probability of observing an intact filament by electron microscopy is extremely low. In contrast to skeletal muscle, which contains a transverse alignment of thick and thin filaments that results in striations, the contractile filaments in smooth muscle are not in uniform transverse alignment, and thus smooth muscle has no striations. The lack of striations in smooth muscle does not imply a lack of order. The thick and thin filaments are organized in contractile units that are analogous to sarcomeres.
The thin filaments of smooth muscle have an actin and tropomyosin composition and structure similar to that in skeletal muscle. However, the cellular content of actin and tropomyosin in smooth muscle is about twice that of striated muscle. Smooth muscle lacks troponin and nebulin but contains two proteins not found in striated muscle: caldesmon and calponin. The precise roles of these proteins are unknown, but they do not appear to be fundamental to cross-bridge cycling. It has been suggested that calponin may inhibit the binding of unphosphorylated myosin to actin. Most of the myoplasm is filled with thin filaments that are roughly aligned along the long axis of the cell. The myosin content of smooth muscle is only a fourth that of striated muscle. Small groups of three to five thick filaments are aligned and surrounded by many thin filaments. These groups of thick filaments with interdigitating thin filaments are connected to dense bodies or areas (Figs. 14-4 and 14-5) and represent the equivalent of the sarcomere. To maintain alignment of the contractile apparatus along the long axis of the cell, the thick and thin filaments of some smooth muscles do not appear to circumvent the centrally located nucleus but instead may connect to (or near) the nucleus. The contractile apparatus of adjacent cells is mechanically coupled by the links between membrane-dense areas (Fig. 14-4).
Cytoskeleton
The cytoskeleton in smooth muscle cells serves as an attachment point for the thin filaments and permits transmission of force to the ends of the cell. In contrast to skeletal muscle, the contractile apparatus in smooth muscle is not organized into myofibrils, and Z lines are lacking. The functional equivalents of the Z lines in smooth muscle cells are ellipsoidal dense bodies in the myoplasm and dense areas that form bands along the sarcolemma (Figs. 14-3 to 14-5). These structures serve as attachment points for the thin filaments and contain α-actinin, a protein also found in the Z lines of striated muscle. Intermediate filaments with diameters between those of thin filaments (7 nm) and thick filaments (15 nm) are prominent in smooth muscle. These filaments link the dense bodies and areas into a cytoskeletal network (Fig. 14-4). The intermediate filaments consist of protein polymers of desmin or vimentin.
CONTROL OF SMOOTH MUSCLE ACTIVITY
The contractile activity of smooth muscle can be controlled by numerous factors, including hormones, autonomic nerves, pacemaker activity, and a variety of drugs. Like skeletal or cardiac muscle, contraction of smooth muscle is dependent on Ca++, and the agents just listed induce smooth muscle contraction by increasing intracellular [Ca++]. However, in contrast to skeletal or cardiac muscle, action potentials in smooth muscle are highly variable and not always needed to initiate contraction. Moreover, several agents can increase intracellular [Ca++] and hence contract smooth muscle without changing the membrane potential. Figure 14-6 shows various types of action potentials in smooth muscle and the corresponding changes in force. An action potential in smooth muscle can be associated with a slow twitch-like response, and the twitch forces can summate during periods of repetitive action potentials (i.e., similar to tetany in skeletal muscle). Such a pattern of activity is characteristic of single-unit smooth muscle in many viscera.
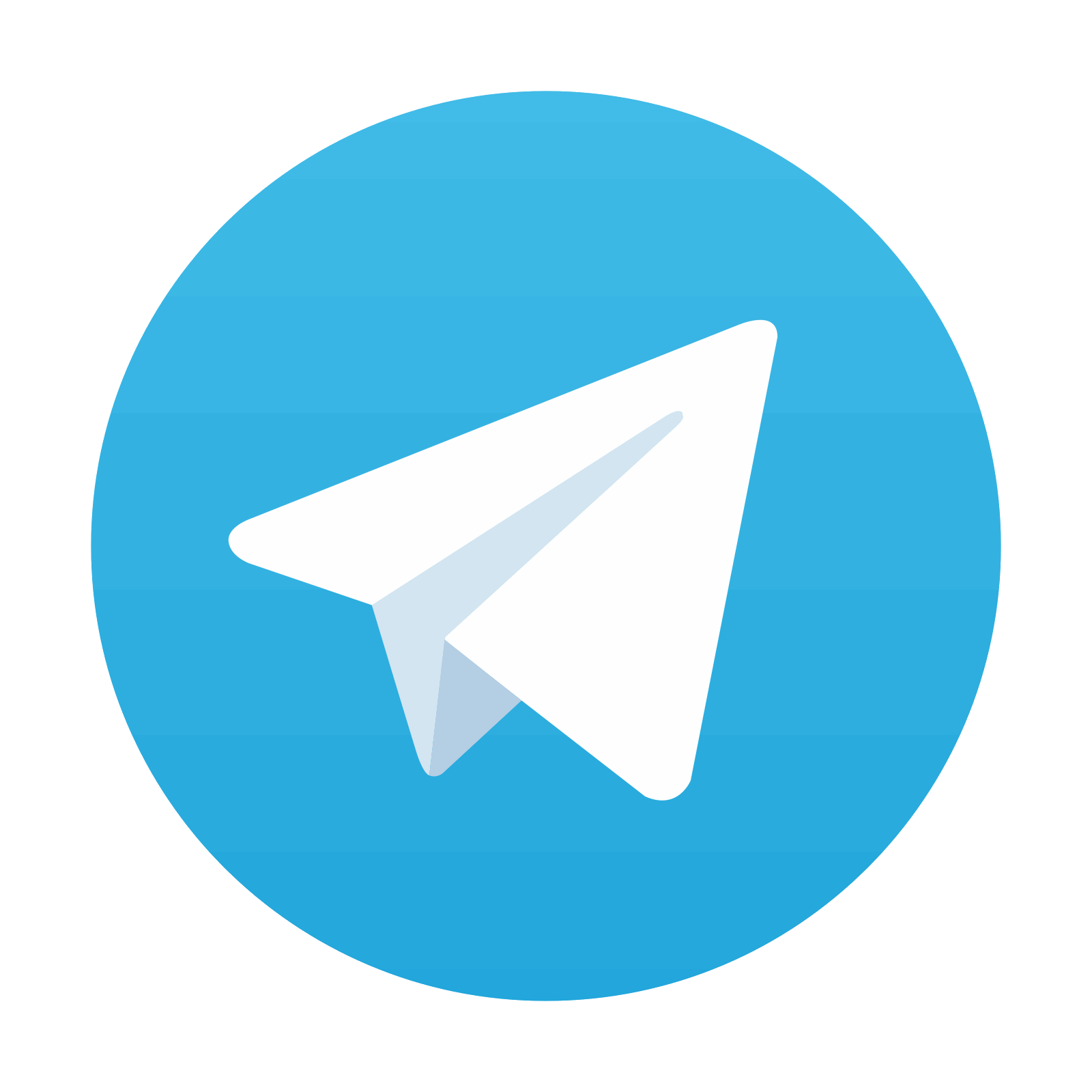