OBJECTIVES
After studying this chapter, you should be able to:
Describe the basic features of the neural elements in the olfactory epithelium and olfactory bulb.
Describe signal transduction in odorant receptors.
Outline the pathway by which impulses generated in the olfactory epithelium reach the olfactory cortex.
Describe the location and cellular composition of taste buds.
Name the five major taste receptors and signal transduction mechanisms in these receptors.
Outline the pathways by which impulses generated in taste receptors reach the insular cortex.
INTRODUCTION
Smell (olfaction) and taste (gustation) are generally classified as visceral senses because of their close association with gastrointestinal function. Physiologically, they are related to each other. The flavors of various foods are in large part a combination of their taste and smell. Consequently, food may taste “different” if one has a cold that depresses the sense of smell. Both smell and taste receptors are chemoreceptors that are stimulated by molecules in solution in mucus in the nose and saliva in the mouth. Because stimuli arrive from an external source, they are also classified as exteroceptors. The sensations of smell and taste allow individuals to distinguish between estimates of up to 30 million compounds that are present in food, predators, and mates and to convert the information received into appropriate behaviors.
SMELL
Olfactory sensory neurons are located in a specialized portion of the nasal mucosa, the yellowish pigmented olfactory epithelium. In dogs and other animals in which the sense of smell is highly developed (macrosmatic animals), the area covered by this membrane is large; in microsmatic animals, such as humans, it is small. In humans, it covers an area of 10 cm2 in the roof of the nasal cavity near the septum (Figure 11–1). The olfactory epithelium is said to be the place in the body where the nervous system is closest to the external world.
FIGURE 11–1
Structure of the olfactory epithelium. There are three cell types: olfactory sensory neurons, supporting (sustentacular) cells, and basal stem cells at the base of the epithelium. Each olfactory sensory neuron has a dendrite that projects to the epithelial surface. Numerous cilia protrude into the mucus layer lining the nasal lumen. Odorants bind to specific odorant receptors on the cilia and initiate a cascade of events leading to generation of action potentials in the sensory axon. Each olfactory sensory neuron has a single axon that projects to the olfactory bulb, a small ovoid structure that rests on the cribriform plate of the ethmoid bone. (Reproduced with permission from Kandel ER, Schwartz JH, Jessell TM [editors]: Principles of Neural Science, 4th ed. New York, NY: McGraw-Hill; 2000.)
The human olfactory epithelium contains about 50 million bipolar olfactory sensory neurons interspersed with glia-like supporting (sustentacular) cells and basal stem cells. New olfactory sensory neurons are generated by basal stem cells as needed to replace those damaged by exposure to the environment. The olfactory epithelium is covered by a thin layer of mucus secreted by the supporting cells and Bowman glands, which lie beneath the epithelium.
Each olfactory sensory neuron has a short, thick dendrite that projects into the nasal cavity where it terminates in a knob containing 6–12 cilia (Figure 11–1). In humans, the cilia are unmyelinated processes, about 5–10 μm long and 0.1–2 μm in diameter that protrude into the mucus overlying the epithelium. Odorant molecules (chemicals) dissolve in the mucus and bind to odorant receptors on the cilia of olfactory sensory neurons. The mucus provides the appropriate molecular and ionic environment for odor detection.
The axons of the olfactory sensory neurons (first cranial nerve) pass through the cribriform plate of the ethmoid bone and enter the olfactory bulbs (Figure 11–1). In the olfactory bulbs, the axons of the olfactory sensory neurons contact the primary dendrites of the mitral cells and tufted cells (Figure 11–2) to form anatomically discrete synaptic units called olfactory glomeruli. The olfactory bulbs also contain periglomerular cells, which are inhibitory neurons connecting one glomerulus to another, and granule cells, which have no axons and make reciprocal synapses with the lateral dendrites of the mitral and tufted cells (Figure 11–2). At these synapses, the mitral or tufted cells excite the granule cell by releasing glutamate, and the granule cells in turn inhibit the mitral or tufted cell by releasing GABA.
FIGURE 11–2
Basic neural circuits in the olfactory bulb. Note that olfactory receptor cells with one type of odorant receptor project to one olfactory glomerulus (OG) and olfactory receptor cells with another type of receptor project to a different OG. Solid black arrows signify inhibition via GABA release, and white arrows signify excitatory connections via glutamate release. CP, cribriform plate; Gr, granule cell; M, mitral cell; PG, periglomerular cell; T, tufted cell. (Adapted with permission from Mori K, et al: The olfactory bulb: coding and processing of odor molecular information, Science 1999 Oct 22; 286(5440):711–715.)
Free endings of many trigeminal pain fibers are found in the olfactory epithelium. They are stimulated by irritating substances, which leads to the characteristic “odor” of such substances as peppermint, menthol, and chlorine. Activation of these endings by nasal irritants also initiates sneezing, lacrimation, respiratory inhibition, and other reflexes.
The tufted cells are smaller than the mitral cells and they have thinner axons, but they are similar from a functional point of view. The axons of the mitral and tufted cells pass posteriorly through the lateral olfactory stria to terminate on apical dendrites of pyramidal cells in five regions of the olfactory cortex: anterior olfactory nucleus, olfactory tubercle, piriform cortex, amygdala, and entorhinal cortex (Figure 11–3). From these regions, information travels directly to the frontal cortex or via the thalamus to the orbitofrontal cortex. Conscious discrimination of odors is dependent on the pathway to the orbitofrontal cortex. The orbitofrontal activation is generally greater on the right side than on the left; thus, cortical representation of olfaction is asymmetric. The pathway to the amygdala is probably involved with the emotional responses to olfactory stimuli, and the pathway to the entorhinal cortex is concerned with olfactory memories.
FIGURE 11–3
Diagram of the olfactory pathway. Information is transmitted from the olfactory bulb by axons of mitral and tufted relay neurons in the lateral olfactory tract. Mitral cells project to five regions of the olfactory cortex: anterior olfactory nucleus, olfactory tubercle, piriform cortex, and parts of the amygdala and entorhinal cortex. Tufted cells project to anterior olfactory nucleus and olfactory tubercle; mitral cells in the accessory olfactory bulb project only to the amygdala. Conscious discrimination of odor depends on the neocortex (orbitofrontal and frontal cortices). Emotive aspects of olfaction derive from limbic projections (amygdala and hypothalamus). (Reproduced with permission from Kandel ER, Schwartz JH, Jessell TM [editors]: Principles of Neural Science, 4th ed. New York, NY: McGraw-Hill; 2000.)
In rodents and various other mammals, the nasal cavity contains another patch of olfactory epithelium located along the nasal septum in a well-developed vomeronasal organ. This structure is concerned with the perception of odors that act as pheromones. Vomeronasal sensory neurons project to the accessory olfactory bulb (Figure 11–3) and from there to the amygdala and hypothalamus that are concerned with reproduction and ingestive behavior. Vomeronasal input has major effects on these functions. An example is pregnancy block in mice; the pheromones of a male from a different strain prevent pregnancy as a result of mating with that male, but mating with a mouse of the same strain does not produce blockade. The vomeronasal organ has about 100 G-protein-coupled odorant receptors that differ in structure from those in the rest of the olfactory epithelium.
The organ is not well developed in humans, but an anatomically separate and biochemically unique area of olfactory epithelium occurs in a pit in the anterior third of the nasal septum, which appears to be a homologous structure. There is evidence for the existence of pheromones in humans, and there is a close relationship between smell and sexual function. Perfume advertisements bear witness to this. The sense of smell is said to be more acute in women than in men, and in women it is most acute at the time of ovulation. Smell and, to a lesser extent, taste have a unique ability to trigger long-term memories, a fact noted by novelists and documented by experimental psychologists.
The olfactory system has received considerable attention in recent years because of the intriguing biologic question of how a simple sense organ such as the olfactory epithelium and its brain representation, which apparently lacks a high degree of complexity, can mediate discrimination of more than 10,000 different odors. One part of the answer to this question is that there are many different odorant receptors.
There are approximately 500 functional olfactory genes in humans, accounting for about 2% of the human genome. The amino acid sequences of odorant receptors are very diverse, but all the odorant receptors are G-protein-coupled receptors (GPCRs). When an odorant molecule binds to its receptor, the G-protein subunits (α, β, γ) dissociate (Figure 11–4). The α-subunit activates adenylyl cyclase to catalyze the production of cAMP, which acts as a second messenger to open cation channels, increasing the permeability to Na+, K–, and Ca2+. The net effect is an inward-directed Ca2+ current which produces the graded receptor potential. This then opens Ca2+-activated Cl– channels, further depolarizing the cell due to the high intracellular Cl– levels in olfactory sensory neurons. If the stimulus is sufficient for the receptor potential to exceed its threshold, an action potential in the olfactory nerve (first cranial nerve) is triggered.
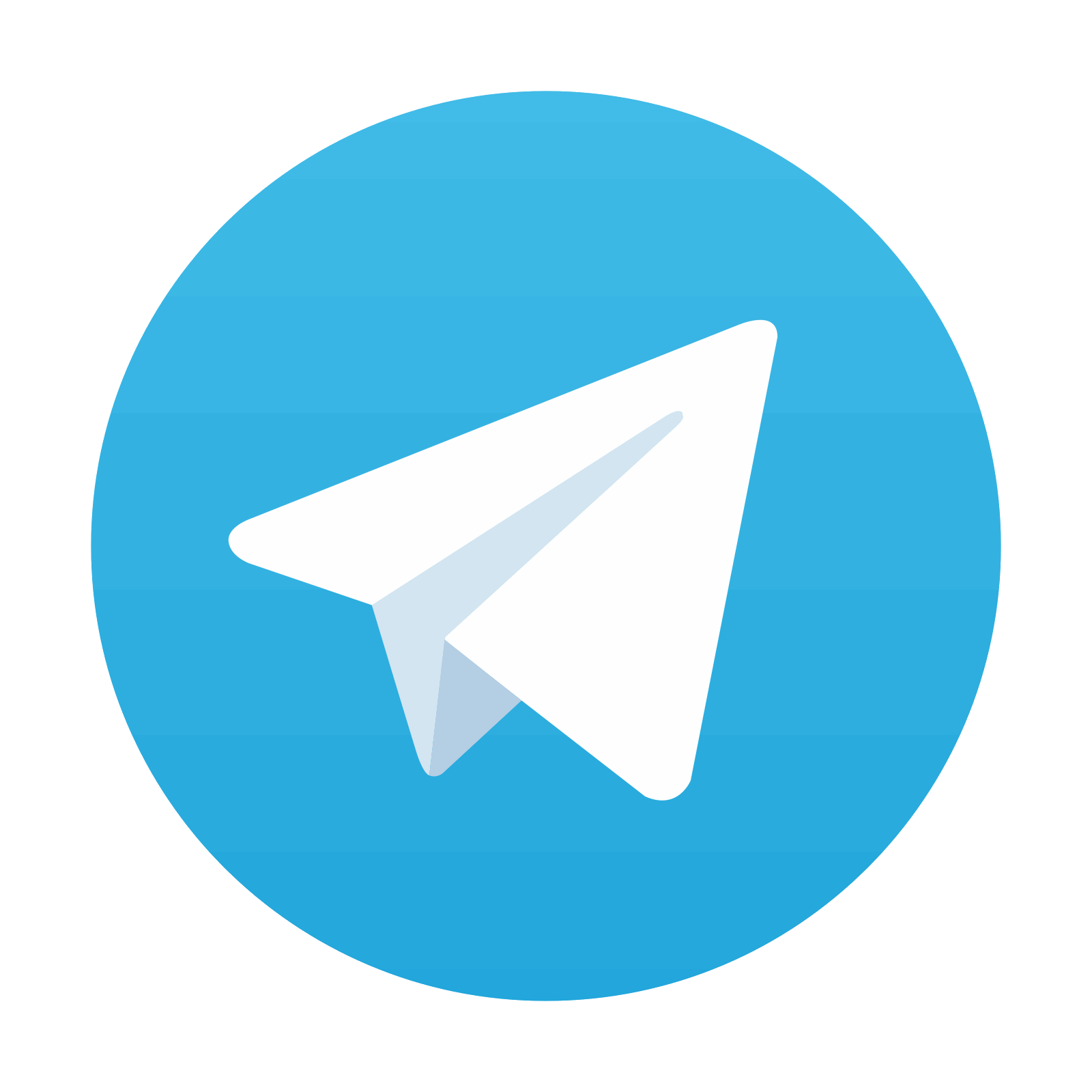
Stay updated, free articles. Join our Telegram channel

Full access? Get Clinical Tree
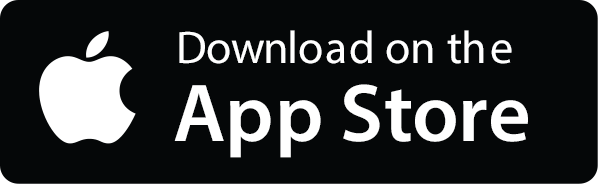
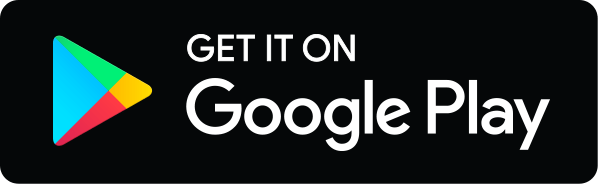