CHAPTER 3 Signal Transduction, Membrane Receptors, Second Messengers, and Regulation of Gene Expression
The human body is composed of billions of cells, each with a distinct function. However, the function of cells is tightly coordinated and integrated by external chemical signals, including hormones, neurotransmitters, growth factors, odorants, and products of cellular metabolism that serve as chemical messengers and provide cell-to-cell communication. Light and mechanical and thermal stimuli are physical external signals that also coordinate cellular function. Chemical and physical messengers interact with receptors located in the plasma membrane, cytoplasm, and nucleus. Interaction of these messengers with receptors initiates a cascade of signaling events that mediate the response to each stimulus. These signaling pathways ensure that the cellular response to external messengers is specific, amplified, tightly regulated, and coordinated. This chapter provides an overview of how cells communicate via external messengers and discusses the receptors and intracellular signaling pathways that process external information into a highly coordinated cellular response. In subsequent chapters, details on signaling pathways in the nervous system, muscle, cardiovascular system, respiratory system, gastrointestinal system, kidneys, and endocrine system will be discussed in greater detail.
CELL-TO-CELL COMMUNICATION
An overview of how cells communicate with each other is presented in Figure 3-1. Cells communicate by releasing extracellular signaling molecules (e.g., hormones and neurotransmitters) that bind to receptor proteins located in the plasma membrane, cytoplasm, or nucleus. This signal is transduced into the activation, or inactivation, of one or more intracellular messengers by interacting with receptors. Receptors interact with a variety of intracellular signaling proteins, including kinases, phosphatases, and GTP-binding proteins (G proteins). These signaling proteins interact with and regulate the activity of target proteins and thereby modulate cellular function. Target proteins include, but are not limited to, ion channels and other transport proteins, metabolic enzymes, cytoskeletal proteins, gene regulatory proteins, and cell cycle proteins that regulate cell growth and division. Signaling pathways are characterized by (1) multiple, hierarchical steps; (2) amplification of the hormone-receptor binding event, which magnifies the response; (3) activation of multiple pathways and regulation of multiple cellular functions; and (4) antagonism by constitutive and regulated feedback mechanisms, which minimize the response and provide tight regulatory control over these signaling pathways. A brief description of how cells communicate follows. Readers who desire a more in-depth presentation of this material are encouraged to consult one of the many cellular and molecular biology textbooks currently available.
Cells in higher animals release hundreds of signaling molecules, including peptides and proteins (e.g., insulin), catecholamines (e.g., epinephrine and norepinephrine), steroid hormones (e.g., aldosterone, estrogen), iodothyronines (e.g., thyroid hormones, including thyroxine [T4] and triiodothyronine [T3]), eicosanoids (e.g., prostaglandins, leukotrienes, thromboxanes, and prostacyclins), and other small molecules, including amino acids, nucleotides, ions (e.g., Ca++), and gases, such as nitric oxide (NO) and carbon dioxide (CO2), into the extracellular space by the processes of exocytosis and diffusion. Secretion of signaling molecules is cell type specific. For example, beta cells in the pancreas release insulin, which regulates glucose uptake into cells. The ability of a cell to respond to a specific signaling molecule depends on the expression of receptors that bind the signaling molecule with high affinity and specificity. Receptors are located in the plasma membrane, the cytosol, and the nucleus (Fig. 3-2).
Signaling molecules can act over long or short distances and can require cell-to-cell contact or very close cellular proximity (Fig. 3-3). Contact-dependent signaling is important during development and in immune responses. Molecules that are released and act locally are called paracrine or autocrine hormones. Paracrine signals are released by one type of cell and act on another type; they are usually taken up by target cells or rapidly degraded (within minutes) by enzymes. Autocrine signaling involves the release of a molecule that affects the same cell or other cells of the same type. Synaptic signaling occurs when neurons transmit electrical signals along their axons and release neurotransmitters at synapses that affect the function of other neurons or cells that are distant from the neuron cell body. The physical relationship between the nerve terminal and the target cell ensures that the neurotransmitter is delivered to a specific cell. Details on synaptic signaling are discussed in Chapter 6. Endocrine signals are hormones that are secreted into the blood and are widely dispersed in the body. Details on endocrine signaling are discussed in Chapter 37.
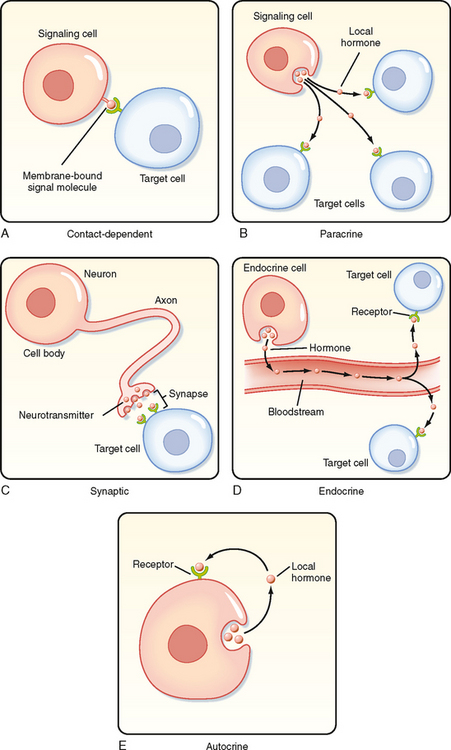
Figure 3-3 Cell-to-cell communication is mediated by five basic mechanisms, described in the text.
(Redrawn from Alberts B et al: Molecular Biology of the Cell, 4th ed. New York, Garland Science, 2002.)
In addition to paracrine, autocrine, endocrine, and synaptic signaling, cell-to-cell communication also occurs via the gap junctions that form between adjacent cells (see Chapter 2). Gap junctions are specialized junctions that allow intracellular signaling molecules, generally less than 1200 Da in size, to diffuse from the cytoplasm of one cell to an adjacent cell. The permeability of gap junctions is regulated by cytosolic [Ca++], [H+], and cAMP and by the membrane potential. Gap junctions also allow cells to be electrically coupled, which is vitally important for the coordinated activity of cardiac and smooth muscle cells (see Chapters 13 and 14).
The speed of a response to an extracellular signal depends on the mechanism of delivery. Endocrine signals are relatively slow (seconds to minutes) because time is required for diffusion and blood flow to the target cell, whereas synaptic signaling is extremely fast (milliseconds). If the response involves changes in the activity of proteins in the cell, the response may occur in milliseconds to seconds. However, if the response involves changes in gene expression and the de novo synthesis of proteins, the response may take hours to occur, with days required to achieve a maximal response. For example, the stimulatory effect of aldosterone on sodium transport by the kidney requires days to fully develop (see Chapter 34).
The response to a particular signaling molecule also depends on the ability of the molecule to reach a particular cell, on expression of the cognate receptor (i.e., receptors that recognize a particular signaling molecule or ligand with a high degree of specificity), and on the cytoplasmic signaling molecules that interact with the receptor. Thus, signaling molecules frequently have many different effects that are dependent on the cell type. For example, the neurotransmitter acetylcholine stimulates contraction of skeletal muscle but decreases the force of contraction in heart muscle. This is due to the fact that skeletal muscle and heart cells express different acetylcholine receptors.*
RECEPTORS
All signaling molecules bind to specific receptors that act as signal transducers, thereby converting a ligand-receptor binding event into intracellular signals that affect cellular function. Receptors can be divided into two basic classes based on their structure and mechanism of action: membrane receptors and nuclear receptors (Table 3-1 and Figs. 3-4 and 3-5).
Table 3-1 Classes of Membrane Receptors
Receptor Class | Ligand | Signal Transduction Pathway/Target |
---|---|---|
A. Ion channel | Extracellular ligand: | Membrane currents: |
GABA | Cl− | |
ACh | Na+, K+, Ca++ | |
ATP | Ca++, Na+ | |
K+ | ||
Intracellular ligand: | ||
cAMP | Na+, K+ | |
cGMP | Na+, K+ | |
InsP3 | Ca++ | |
Ca++ | Ca++ | |
B. G protein coupled | Neurotransmitters Peptides, Odorants, Cytokines, Lipids | |
C. Catalytic | ANP | Receptor guanylyl cyclase |
Insulin, EGF | Receptor tyrosine kinase | |
Bind to regulatory sequences in DNA and increase or decrease gene transcription | ||
Bind to regulatory sequences in DNA and increase or decrease gene transcription |
ACh, acetylcholine; ANP, atrial natriuretic peptide; cAMP, cyclic adenosine monophosphate; cGMP, cyclic guanosine monophosphate; EGF, epidermal growth factor; GABA, γ-aminobutyric acid; InsP3, inositol 1,4,5-triphosphate.
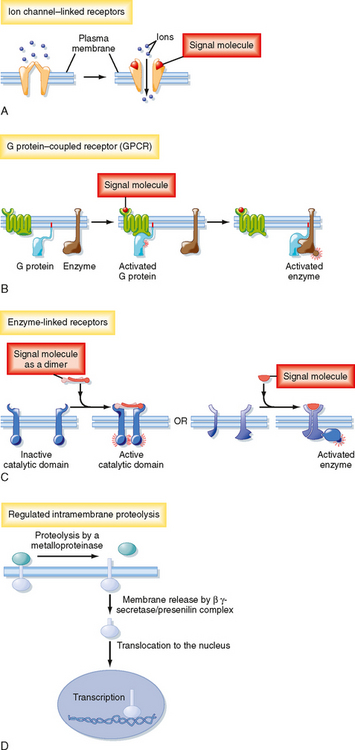
Figure 3-4 Classes of plasma membrane receptors. See text for details.
(Redrawn from Alberts B et al: Molecular Biology of the Cell, 4th ed. New York, Garland Science, 2002.)
Plasma Membrane Receptors
There are four major types of plasma membrane receptors defined by the intracellular signaling pathways that they use: ion channel–linked receptors, G protein–coupled receptors (GPCRs), catalytic receptors, and a fourth class of transmembrane receptors that when activated, release transcription factors that undergo proteolytic cleavage and liberate a cytosolic fragment that enters the nucleus and modulates gene expression (Fig. 3-4).
Ion channel–linked receptors, also known as ligand-gated ion channels, mediate direct and rapid synaptic signaling between electrically excitable cells (Fig. 3-4, A). Neurotransmitters bind to the receptors and either open or close the ion channel, thereby changing the ionic permeability of the plasma membrane and altering the membrane potential. For examples and more details, see Chapter 2.
GPCRs regulate the activity of other proteins, such as enzymes and ion channels (Fig. 3-4, B). In this type of receptor the interaction between the receptor and the target protein is mediated by heterotrimeric G proteins, which are composed of α, β, and γ subunits. Stimulation of G proteins by ligand-bound receptors activates or inhibits downstream target proteins that regulate signaling pathways if the target protein is an enzyme or change membrane ion permeability if the target protein is an ion channel.
Catalytic receptors either function as enzymes or are associated with and regulate enzymes (Fig. 3-4, C). Most enzyme-linked receptors are protein kinases or are associated with protein kinases, and ligand binding causes the kinases to phosphorylate a specific subset of proteins on specific amino acids, which in turn activates or inhibits protein activity.
Some membrane proteins do not fit the classic definition of receptors, yet they subserve a receptor-like function in that they recognize extracellular signals and transduce the signals into an intracellular second messenger that has a biological effect. For example, on activation by a ligand, some membrane proteins undergo regulated intramembrane proteolysis (RIP), which elaborates a cytosolic peptide fragment that enters the nucleus and regulates gene expression (Fig. 3-4, D). In this signaling pathway, binding of ligand to a plasma membrane receptor leads to ectodomain shedding, facilitated by members of the metalloproteinase-disintegrin family, and produces a carboxy-terminal fragment that is the substrate for γ-secretase. γ-Secretase induces RIP, thereby releasing an intracellular domain of the protein that enters the nucleus and regulates transcription (Fig. 3-4, D). The most well characterized example of RIP is the sterol regulatory element–binding protein (SREB), a transmembrane protein expressed in the membrane of the endoplasmic reticulum. When cellular cholesterol levels are low, SREB undergoes RIP and the proteolytically cleaved fragment is translocated into the nucleus, where it transcriptionally activates genes that promote cholesterol biosynthesis.
< div class='tao-gold-member'>
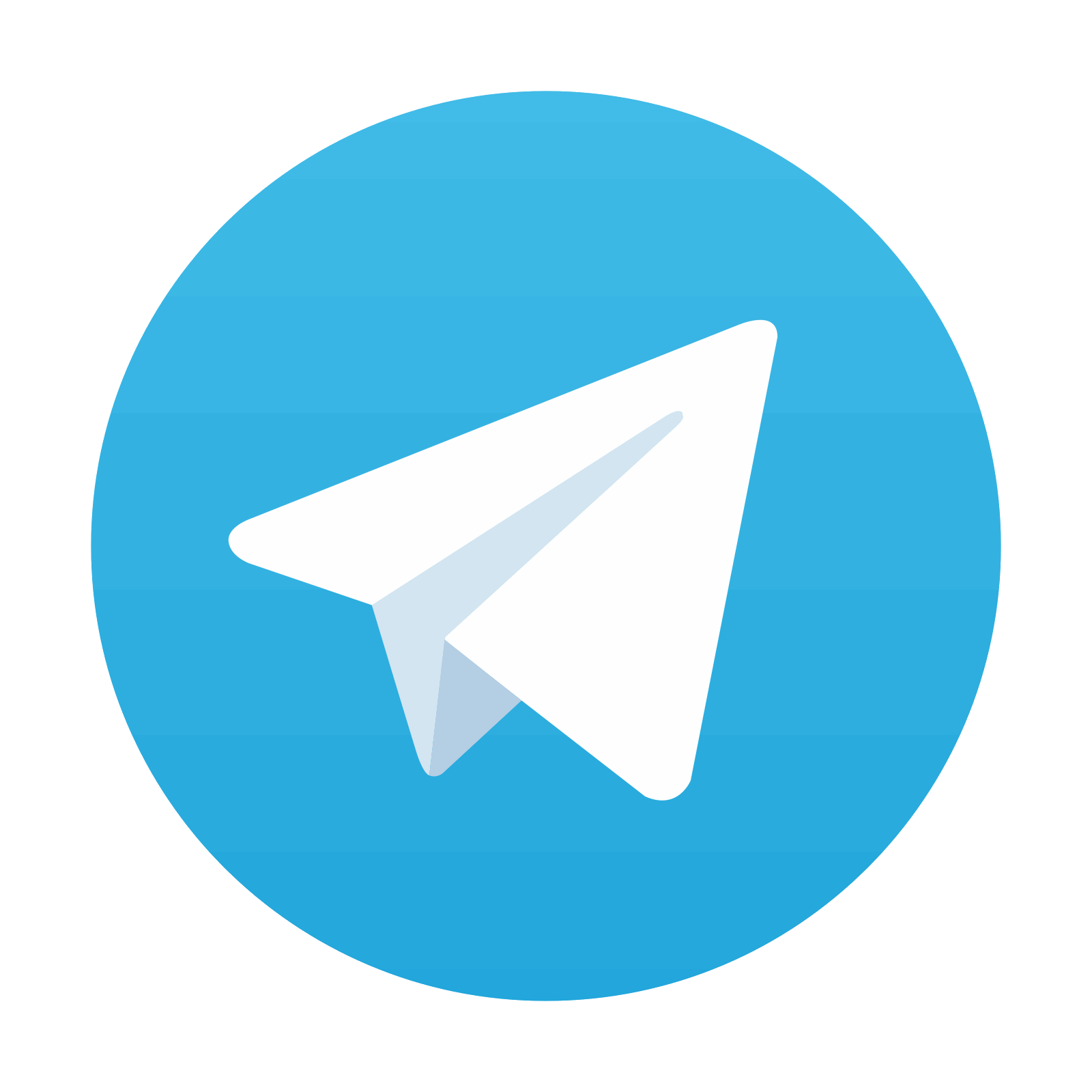
Stay updated, free articles. Join our Telegram channel

Full access? Get Clinical Tree
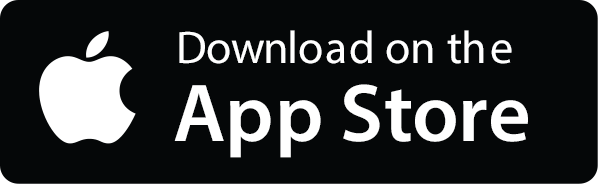
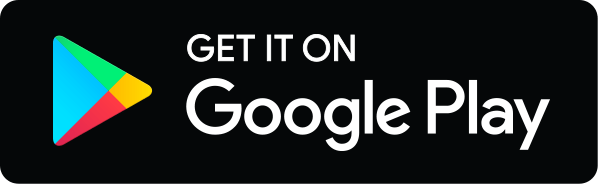