Chapter 5 Respiratory Physiology
I. Overview
A. Because it is essential for metabolism, oxygen must be provided in relatively large amounts to most cells.
II. Functional Anatomy of the Respiratory System
A. Overview
1. The respiratory system is composed of large conducting airways, which conduct air to the smaller respiratory airways.
B. Conducting airways
2. Despite their larger size, airway resistance is greater than in the respiratory airways because the conducting airways are arranged in series and airflow resistance in series is additive.
3. Bronchi (Table 5-1)
• The bronchi are large airways (>1 mm in diameter) that contain supportive cartilage rings.
TABLE 5-1 Comparison of Bronchi and Bronchioles
Parameter | Bronchi | Conducting Bronchioles |
---|---|---|
Smooth muscle | Present (many layers) | Present (1-3 layers) |
Cartilage | Yes | No |
Epithelium | Pseudostratified columnar | Simple cuboidal |
Ciliated | Yes | Yes (less) |
Diameter | Independent of lung volume | Depends on lung volume |
Location | Intraparenchymal and extraparenchymal | Embedded directly within connective tissue of lung |
4. Mucociliary tract
• Bronchial epithelium comprises pseudostratified columnar cells, many of which are ciliated, interspersed with mucus-secreting goblet cells.
• The mucus traps inhaled foreign particles before they reach the alveoli.
a. It is then transported by the beating cilia proximally toward the mouth, so that it can be swallowed or expectorated.
C. Respiratory airways (Table 5-2)
1. These include respiratory bronchioles, alveolar ducts, and alveoli, where gas exchange occurs.
TABLE 5-2 Comparison of Conducting and Respiratory Airways
Parameter | Conducting Airways | Respiratory Airways |
---|---|---|
Histology | Ciliated columnar tissue | Nonciliated cuboidal tissue |
Goblet cells (mucociliary tract) | No goblet cells | |
Lacks smooth muscle | ||
Presence of cartilage | Yes | No |
Resistance | Large diameter | Small diameter |
Arranged in series | Arranged in parallel | |
High resistance | Low resistance |
2. Despite their smaller size, airway resistance is less than in conducting airways, because the respiratory airways are arranged in parallel, and airflow resistances in parallel are added reciprocally.
D. Pulmonary membrane: the “air-blood” barrier (Fig. 5-1)
1. This is a thin barrier that separates the alveolar air from the pulmonary capillary blood, through which gas exchange must occur.
2. It comprises multiple layers, including, from the alveolar space “inward”:
• Epithelial and capillary basement membranes, separated by a thin interstitial space (fused in areas)
III. Mechanics of Breathing
B. Inspiration
1. Overview
• Inspiration is an active process that requires substantial expansion of the thoracic cavity to accommodate the inspired air (Fig. 5-2).
2. Driving force for inspiration
• A negative intrapleural pressure is created by movement of the diaphragm downward and the chest wall outward.
• The relationship between intrapleural pressure and lung volume is expressed by Boyle’s law:
where
P1 = intrapleural pressure at start of inspiration
P2 = intrapleural pressure at end of inspiration
V1 = lung volume at start of inspiration
V2 = lung volume at end of inspiration
a. Boyle’s law shows that as lung volume increases during inspiration, the intrapleural pressure must decrease (become more negative).
C. Expiration
1. Overview
• Usually a passive process in which relaxation of the diaphragm, combined with elastic recoil of the lungs and chest wall, forces air from the lungs
2. Driving forces for expiration
• An increase in intrapleural pressure is created by movement of the diaphragm upward and the chest wall inward.
a. This increase is then transmitted to the terminal air spaces (alveolar ducts and alveoli) and compresses them, causing air to leave the lungs.
3. Sources of resistance during expiration
• As the volume of the thoracic cavity decreases during expiration, the intrathoracic pressure increases (recall Boyle’s law—the inverse relationship of pressure and volume).
• The increased pressure compresses the airways and reduces airway diameter.
a. This reduction in airway diameter is the primary source of resistance to airflow during expiration.
• Figure 5-4 shows a flow-volume curve recorded during inspiration and expiration in a normal subject.
D. Work of breathing
2. Airway resistance
• As inspired air courses along the airways, friction, and therefore airway resistance, is generated between air molecules and the walls of conducting airways.
• Because air is essentially a fluid of low viscosity, airflow resistance can be equated to the resistance encountered by a fluid traveling through a rigid tube.
a. Poiseuille’s equation relates airflow resistance (R), air viscosity (η), airway length (l), and airway radius (r), assuming laminar rather than turbulent airflow:
b. In the lung, air viscosity and airway length are basically unchanging constants, whereas airway radius can change dramatically.
• Contribution of large and small airways to resistance
a. Under normal conditions, most of the total airway resistance actually comes from the large conducting airways.
b. By contrast, the small airways (terminal bronchioles, respiratory bronchioles, and alveolar ducts) provide relatively little resistance.
c. Resistance is low in smaller-diameter airways despite the fact that Poiseuille’s equation states that resistance is inversely proportional to the fourth power of airway radius.
• This is because the branches of the small airways have a total cross-sectional area that is greater than that of the larger airways from which they branch.
3. Compliance resistance (work)
• As the lungs inflate, work must be performed to overcome the intrinsic elastic recoil of the lungs.
4. Tissue resistance
• As the pleural surfaces slide over each other during the respiratory cycle, friction and therefore resistance is generated.
• A small amount of pleural fluid in the pleural space acts to lubricate these surfaces, thereby minimizing the friction.
E. Pulmonary compliance (C)
2. Defined as the change in volume (ΔV) required for a fractional change of pulmonary pressure (ΔP):
3. Compliance of the lungs (Fig. 5-6)
• In the schematic, note that the inspiratory curve has a different shape than the expiratory curve.
• The lagging of an effect behind its cause, in which the value of one variable depends on whether the other has been increasing or decreasing, is referred to as hysteresis.
• Hysteresis is an intrinsic property of all elastic substances, and the compliance curve of the lungs represents the difference between the inspiratory and expiratory curves.
4. Compliance of the combined lung–chest wall system (Fig. 5-7)
• In the schematic, note that at functional residual capacity (FRC), the lung–chest wall system is at equilibrium.
• In other words, at FRC, the collapsing pressure from the elastic recoil of the lungs is equal to the outward pressure exerted from the chest wall.
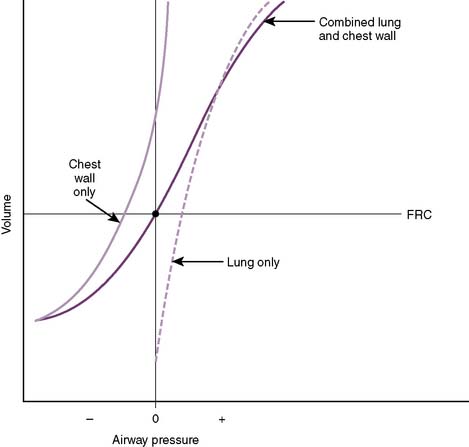
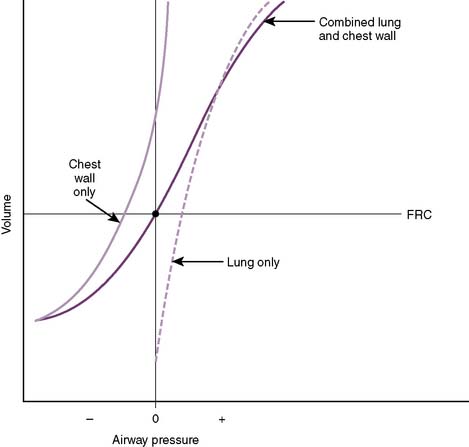
5-7 Compliance of the lungs and chest wall separately and together. FRC, Functional residual capacity.
(From West JB: Respiratory Physiology: The Essentials, 8th ed. Philadelphia, Lippincott Williams & Wilkins, 2008, Fig. 7-11.)
F. Pulmonary elastance
2. Pulmonary elastance (E) is the pressure (P) required for a fractional change of lung volume (ΔV):
G. Surface tension
2. The water molecules are attracted to each other through noncovalent hydrogen bonds and are repelled by the hydrophobic alveolar air.
3. The attractive forces between water molecules generate surface tension (T), which in turn produces a collapsing pressure, which acts to collapse the alveoli.
4. Laplace’s law states that collapsing pressure is inversely proportional to the alveolar radius, such that smaller alveoli experience a larger collapsing pressure:
where
5. Figure 5-8 demonstrates that saline-inflated lungs are more compliant that air-inflated lungs because of reduced surface tension and collapsing pressures.
H. Role of surfactant
1. Surfactant is a complex phospholipid secreted onto the alveolar membrane by type 2 epithelial cells.
• It minimizes the interaction between alveolar fluid and alveolar air (Fig. 5-9), which reduces surface tension.
2. Surfactant reduces compliance resistance (work) of the lungs.
• A moderate amount of surface tension is beneficial because it generates a collapsing pressure that contributes to the elastic recoil of the lungs during expiration.
• However, if collapsing pressure were to become pathologically elevated, lung inflation during inspiration would become impaired.
IV. Gas Exchange
B. Partial pressure of gases
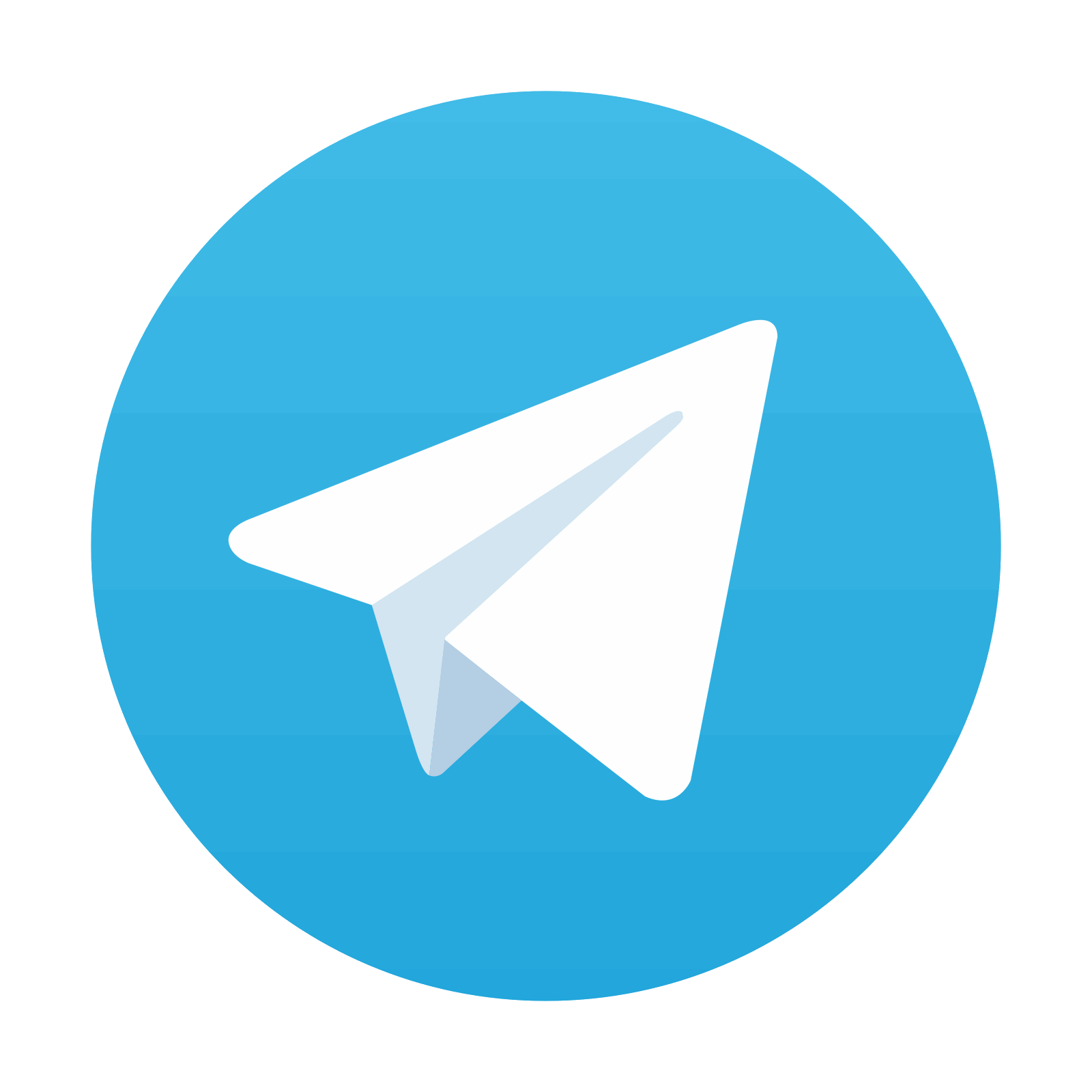
1. According to Dalton’s law, the partial pressure exerted by a gas in a mixture of gases is proportional to the fractional concentration of that gas:
where
Px = partial pressure of the gas (mm Hg)
PB = barometric pressure (mm Hg)
F = fractional concentration of the gas
2. The partial pressure of O2 in the atmosphere (Po2) at sea level, which has a fractional concentration of 21%, is calculated as follows:
3. The partial pressure of O2 in humidified tracheal air is calculated as follows:
• Note that the addition of H2O vapor decreases the percent concentration of O2 in alveolar air and hence decreases its partial pressure (Table 5-3).
< div class='tao-gold-member'>
Only gold members can continue reading. Log In or Register a > to continue
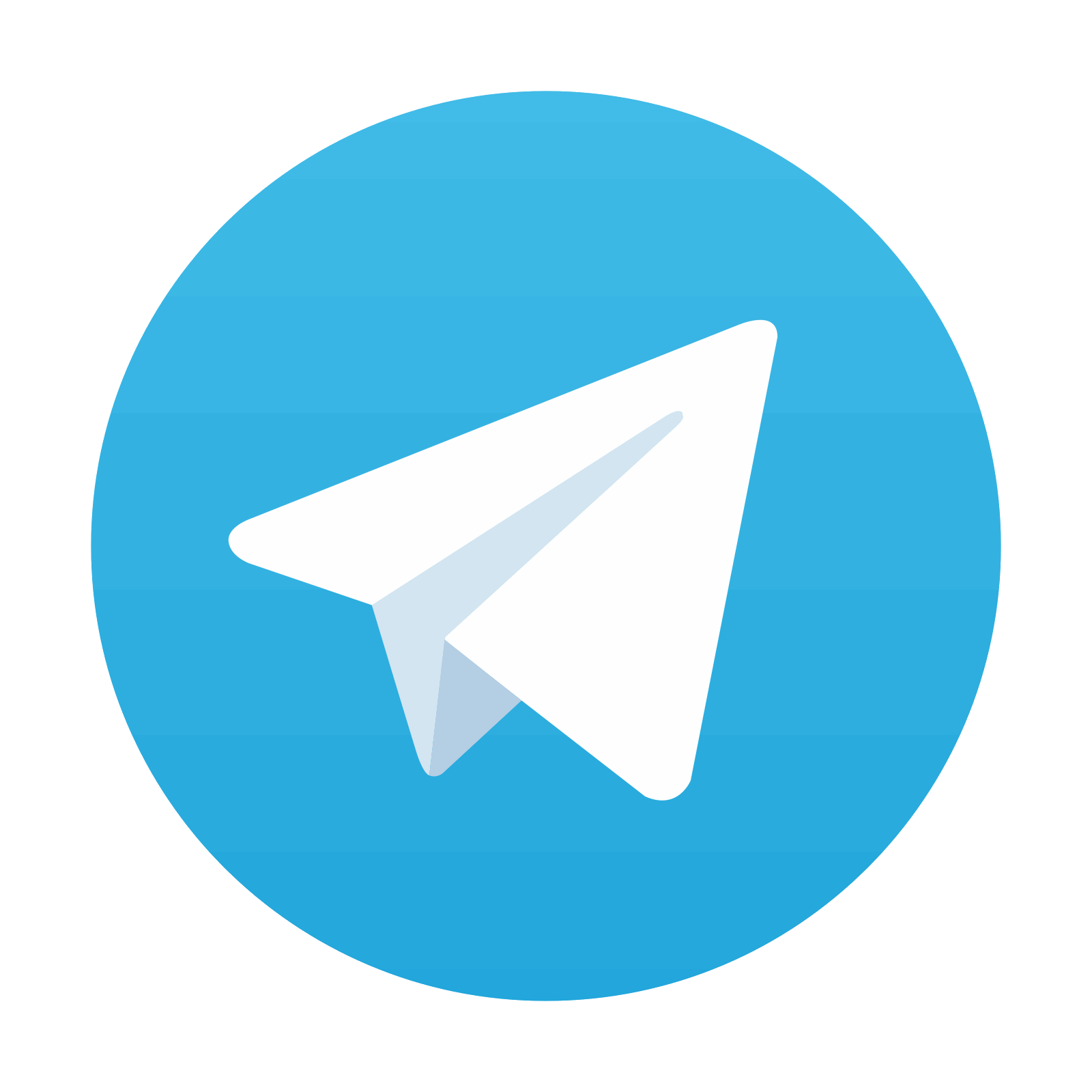
Stay updated, free articles. Join our Telegram channel

Full access? Get Clinical Tree
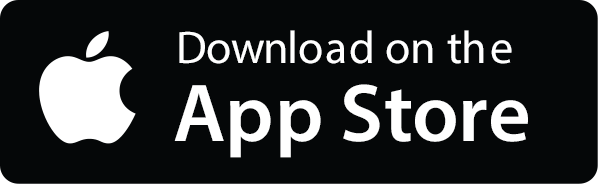
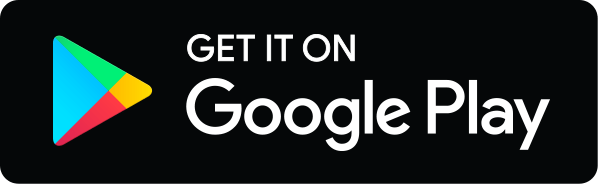