Figure 9.1. Schematic depicting the size of various substances. Proteins and other biomolecules such as DNA fall within nanometre range.2
Translational Medicine
Translational medicine is an up and coming branch of science, which looks at medical practice and interventional epidemiology from evidence-based medicine. It integrates inputs from various research advances of basic science in a socio-political and economic environment to optimise patient care to develop preventive medicine, which sometimes falls out of the periphery of conventional health care. In translational medicine, biomedical engineers and scientists take an alternative point of view to convert novel basic research discoveries from bench to bedside for the diagnosis and treatment of various critical diseases.4 They use and provide opportunities to develop cutting edge technologies; qualify, validate and identify genes and gene products and measure levels of transcription of all known genes, protein products in the blood and tissue for clinical use in terms of personalised medicine. The following processes underlie translational medicine5:
This line of research represents the science of tomorrow as this is bringing a paradigm shift in bioresearch. Historically, medicine, biology, drug development and clinical practice were treated as separate entities.5 However, with the advent of translational medicine all these areas converge into a single platform. In this context, recent advances in bioinformatics and genomics provide a solid rationale for bringing translational medicine to the forefront of drug development and sustainability of existing drugs. The disease signatures encrypted at the molecular level are now being used to develop drugs targeting the cause of the disease instead of the symptoms. This has catalysed into pharmaceutical intervention in drug development by creating compounds from a patient-centric point of view.
Nanotechnology in Medicine and Health care
Nanotechnology has facilitated development of novel medical devices, improved patient care in hospitals and outpatient services by opening up opportunities for personalised point of care. Most importantly, it has advanced our capability to diagnose diseases and deliver targeted drugs whether by using nanotechnology platforms for the identification of biomarkers or by advancing our diagnostic imaging modalities or nanodelivery fabrications.
Applications of nanotechnology in medicine have been vigorously researched for the past 10 years. However, this nuanced form of engineering is often complicated because it requires great expertise to integrate different forms of science and engineering to culminate in medical use. On the other hand, it is rewarding to note that progress has been made in integrating those disciplines to advance the field of medicine in the following areas6:
Nanoparticle-Based DDS
This field of study is highly specific and complex. It deals with an intervention of engineering at a molecular plane for medical applications to control disease and tissue damage. There are several advantages of using nanoparticle-based DDS. It increases the half-life of the drug in systemic circulation, allows the drug to be released at a constant rate, reduces the number of administrations, reduces the adverse effects by delivering the drug only to the target site at desired levels and allows simultaneous delivery of many drugs.7,8 Even though there are many commercial products available in the market, there is a lot of room for improving DDS and apply those improvements to various classes of drugs not yet researched.
The scope of this chapter is to familiarise the reader with nanofabrications associated with polymer-, liposome- and magnetic-nanoparticle-based DDS.
Polymer-Based DDS
Often complexes and conjugates are linked with polymers to make advanced drugs with improved efficacy and tolerability. Some of the current research initiatives deal with multiplexing polymers with DNA complexes, polymer–drug conjugates and hydrophobic drugs.9 The amazing potential of these tiny particulates is due to their small dimension and biocompatibility. As they are miniscule, they can circulate freely in the blood stream for extended periods of time allowing the drug to reach the target site.
For example, chemotherapy is currently being used extensively to treat cancer. The primary problem with this form of therapy is its non-specificity to cancer cells. Most of the patients experience undesirable side effects, perhaps because of repeated treatments, which lead to a poor quality of life.9 The encapsulation of the chemotherapy drugs to polymer-based nanosized delivery agents can improve the efficacy of the drug and reduce the adverse effects. These novel formulations are designed to prolong the circulation time of the drug in vivo from few minutes to several hours.
There are numerous polymer-based nanoparticles that have been used as DDS, and a plethora of protocols that have been developed for synthesis or preparation of polymer-based nanoparticles. For example, one of the most widely used polymers for drug delivery is PLGA or poly(lactic-co-glycolic acid) and there are numerous methods available for the preparation of PLGA nanoparticles. One such technique is oil-in-water emulsion technique. In this technique, the biodegradable polymer (PLGA) and the drug to be encapsulated are first dissolved in a solvent, such as ethylene chloride, followed by the solvent emulsification in an aqueous phase like water, hence the name oil-in-water. This technique is also known as emulsion evaporation technique because at the end of particle preparation, the volatile solvent is removed by evaporation to a gaseous phase.10 Other methods of PLGA particle preparation include water–oil–water emulsion technique, phase separation methods and spray drying.11,12
Since the approval of PLGA by the US Food and Drug Administration (FDA) as a drug delivery vehicle, numerous polymer-based DDS have been approved for clinical therapeutics. Some examples of polymer-based vehicles have been presented in Table 9.1.6
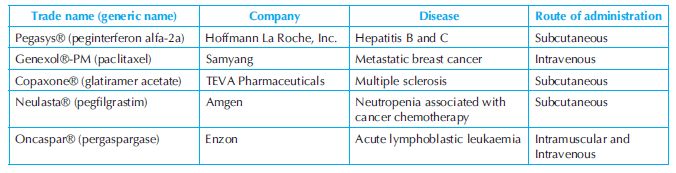
Liposome-Based DDS
Liposomes are lipid-based vesicles with one or more layers of phospholipids. Current applications of liposomes include pharmaceutical drug development.13 This is due to the unique favourable properties of liposomes as they can be used for the following:
Phospholipids are the primary components of liposomes used for their preparation. Phospholipids can be derived from natural sources or synthetic sources. Phosphatidylcholine (PC), phosphatidylenolamine (PE) and phosphatidylserine (PS) are commonly used phospholipids for liposome preparation.14 Cholesterol is usually added during the preparation of liposome to reduce the permeability of the bilayers.
A number of strategies are used for the preparation of liposomes. The simplest and the oldest method is a film-based method first introduced by Bangham et al.15 In this technique, liposomes are produced by hydrating thin lipid films deposited from an organic solution on a glass wall by shaking at temperatures above the transition temperature of the bilayer. Liposome particles of approximately 1 µm in diameter are produced by this method. Other methods include the following15: (i) ultrasonication, (ii) ether vaporisation, (iii) reverse phase evaporation, (iv) freeze-thaw extrusion and (v) dehydration–rehydration.
The size of liposomes is a critical factor that determines its potential applications. Hence, it is necessary to produce liposomes of known size. This is usually achieved by extrusion of liposomes through polycarbonate membranes or alumina membranes. After extrusion, dynamic light scattering is performed to determine the size of the liposome particles. In pharmaceutics, the size, shape and structure play an important role in how much drug gets encapsulated.15 Doxil® was the first liposome-based drug to be approved by the FDA. The FDA has approved a plethora of liposome-based drugs over the last decade. Some examples of drugs based on liposome delivery system are presented in Table 9.2.6
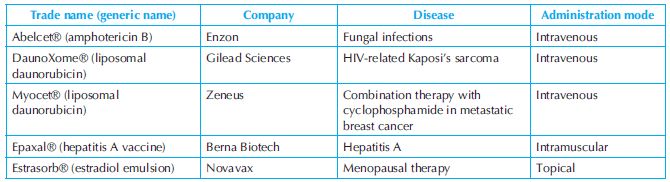
Magnetic Nanoparticles
Magnetic nanoparticles consist of a metal or a metallic oxide core. The magnetic properties of these particles enable them to be applied in numerous applications such as magnetic resonance imaging, thermal ablation or hyperthermia of tumours and site directed or target specific drug delivery.16 Magnetic nanoparticle-based DDS offer several advantages over other DDS: (i) it can be visualised, (ii) it can be guided to a specific site by using a magnetic field and (iii) it can be heated in presence of a magnetic field to trigger drug release.16 Unlike other systems like polymer DDS, magnetic nanoparticles do not have the drawback of high polydispersity. In case of magnetic nanoparticles, the drug is either covalently attached to the surface or encapsulated to the pores of the magnetic carrier. The most common magnetic carrier is mesoporous silica. There are several parameters that are critical for proper functionality of magnetic nanoparticles inside the human body. These parameters include surface, size and magnetic properties. In order to have favourable surface properties (to make them biocompatible) they are coated with hydrophilic compounds like PEG.17 These surface modifications allow covalent bonding of antibodies or drugs to the surface and also increase the half-life inside the body from few minutes to several days. In essence, it can be concluded that a functional magnetic nanoparticle consists of a magnetic core, a protective covering and surface functionality. Hence, appropriate steps in the synthesis of magnetic nanoparticles are critical for proper functionality and they can be synthesised by the following methods16:
Even though magnetic nanoparticles are a promising mode of drug delivery, the method comes with the following limitations: (i) tendency to accumulate at inappropriate places at times, (ii) magnetic field presence is always required to channel the delivery of the drug and (iii) limited penetration into the human body layers due to not very strong magnetic field.16
There are many magnetic nanoparticle-based DDS currently available in the market and some are listed in Table 9.3.16
Table 9.3. List of products based on magnetic nanoparticles
Company | Application |
MicromodPartikeltechnologie GmbH | Drug Delivery and Magnetic Separation |
Polymicrospheres | Drug Delivery and diagnostic assays |
Triton Biosystems | Hyperthermia |
Biophan Technologies | Drug Delivery |
Chemicell GmbH | Drug delivery (FluidMAG) |
In Vivo Imaging
Quantum Dots
One of the key applications of nanotechnology is the development of luminescent nanosized particles. The most popular fluorescent nanoparticles for biological application are quantum dots (QD).18 At nanometre scale, these charged carriers (electrons or holes) exhibit quantum confinement effect at room temperature and give rise to material properties that not only differ from their bulk form but can also be fine tuned by controlling their quantum yield.19 The size of the nanoparticle controls the emission spectrum, while ultraviolet (UV) light excites all QDs. Hence, by selecting the size of the particles, QDs with different emission spectra can be synthesised.18 QDs exhibit the following advantages over organic fluorophores: broad excitation spectra stretching into the UV region, narrow emission spectra, large Stokes shift, high resistance to photobleaching, higher photostability and stronger fluorescence.20
Multiplexing, an attractive way to detect multiple markers, presents challenges, especially for organic fluorophores, due to bleed through in the emission bands and the requirement of multiple excitation sources. Solutions include use of expensive hardware and software combinations such as those used in expensive flow cytometry instruments. In contrast, the broad excitation spectra in QDs allows multiplexing with a single excitation source and their narrow emission spectra addresses the issue of overlapping emission spectra, which is inherent in organic fluorophores.21
Extreme levels of resistance to photobleaching allow QDs to be employed in various systems where high-power excitation sources are used, for example confocal laser scanning microscopy. It has been reported that QDs exhibit 100-fold greater stability to photobleaching than their organic fluorophore counterparts.22
This higher photostability of QDs is crucial for experiments where continuous fluorescence measurements are required, for example in live cell imaging for monitoring particular cellular trafficking events.23 The stronger and brighter fluorescence properties of QDs help in overcoming the auto-fluorescence signal that is particularly high in the in vivo conditions.22,24
QDs are semiconductor nanoparticles, which comprise of a core shell structure. Two of the most widely used commercial QDs come with a core of CdSe or CdTe and a shell of ZnS and emissions from 405 to 805 nm.21,22 The shell stabilises the structure, helps to overcome quenching compared to a QD made only from a core and provides a large surface area available for further modification.
QD conjugation to biomolecules is achieved by different ways from electrostatic binding to noncovalent biotin–streptavidin bonding or covalent bonding. Table 9.4 shows the summary of properties and performance of the different QD-Ab conjugates evaluated for immunohistochemical staining.25
Applications of QDs encompass many areas including single-cell labelling and imaging, imaging of signalling pathways, deep tissue imaging, in vivo tumour and cancer imaging, immunosensor development, assay labelling as well as their use as FRET donors.26 Karwa et al.27 at Drexel University demonstrated the use of QDs in in vivo imaging by detecting the presence of myeloperoxidase (MPO) in the colitis tissue of mice, in vivo.
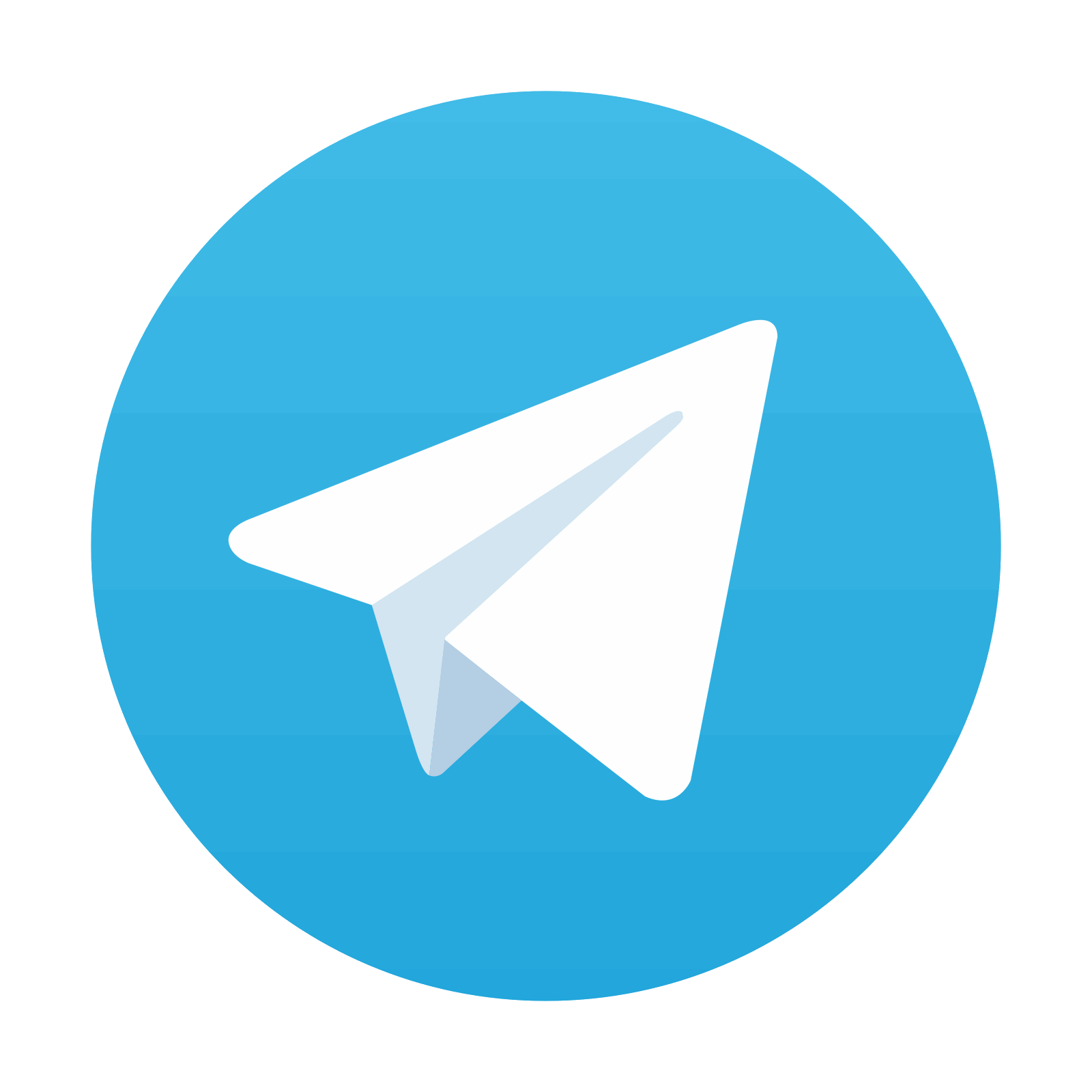
Stay updated, free articles. Join our Telegram channel

Full access? Get Clinical Tree
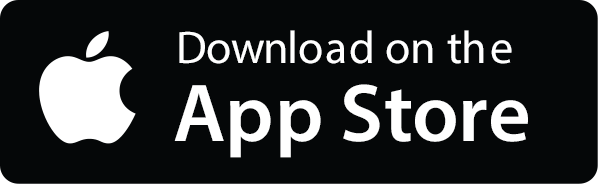
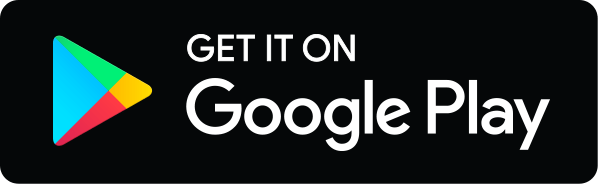