Renal Function
Jacquelyn L. Banasik
Key Questions
• How does the structure of the glomerulus determine the composition of the tubular filtrate?
• What factors determine glomerular filtration rate?
• How do individual nephrons regulate their glomerular filtration rates?
• How are solutes and water transported across the renal tubular epithelium?
• What is the role of the kidney in fluid, electrolyte, and acid-base balance?
• How does renal function change across the life span?
• How are laboratory and diagnostic tests used to evaluate renal function and disease?
http://evolve.elsevier.com/Copstead/
The kidneys are responsible for maintaining fluid and electrolyte homeostasis and ridding the body of water-soluble wastes. To accomplish these functions, the kidneys filter more than 7 L of fluid per hour, then reabsorb about 99%, producing a small amount of urine containing a high concentration of wastes.1 The kidneys can alter the amount and composition of urine to keep blood volume and electrolyte composition within normal limits. In addition, the kidneys perform two important endocrine functions: production of erythropoietin, which is a regulator of red blood cell quantity, and activation of vitamin D, which is a cofactor for intestinal calcium absorption.
Most individuals have two kidneys, each containing approximately one million nephrons, which provide a large renal reserve. The nephron is the functional unit of the kidney, performing all filtration, reabsorption, and secretory functions. Removal of 50% of a person’s nephrons, as occurs with kidney donation, results in no significant impairment of renal function, although renal reserve is reduced.2,3 Serious renal impairment generally does not occur until between 75% and 90% of the total nephrons have been damaged. Thus, clinical findings may not be evident until late in the course of chronic kidney disease. A number of laboratory and diagnostic tests are used to assess renal structure and function and to identify disease processes. These are briefly described at the end of this chapter. Kidney diseases, renal failure, and abnormalities of the bladder are discussed in Chapters 27, 28, and 29, respectively. A discussion of fluid and electrolyte imbalances and acid-base disturbances can be found in Chapters 24 and 25, respectively. The essentials of kidney structure and nephron function are presented in this chapter.
Renal Anatomy
The urinary system consists of the kidneys, ureters, urinary bladder, and urethra (Figure 26-1). The kidneys are located in the retroperitoneal space in the posterior abdomen. One kidney is on each side of the vertebral column between the level of the twelfth thoracic and third lumbar vertebrae. The costovertebral angle (CVA), the point at which the bottom of the rib cage meets the spine, is commonly used as an external landmark for finding kidney position during physical examination.4 The right kidney is located beneath the liver and is placed slightly lower than the left kidney.
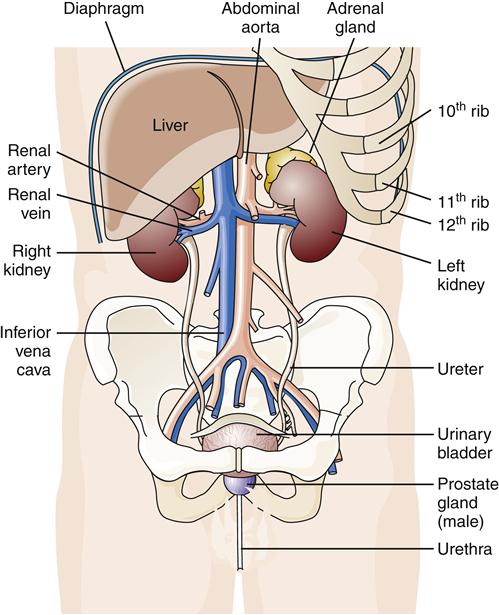
The kidneys are located in the retroperitoneal space in the posterior abdominal cavity, in contact with the diaphragm and covered on the upper portions by ribs.
The kidneys are protected and surrounded by strong back and flank muscles, fascia, and fat. The kidneys are somewhat mobile and can be injured by high-impact activities, such as bouncing along on horseback or on a mountain bike, or by direct trauma, as might occur from falls or blunt trauma. Kidney hemorrhage results in bleeding into the retroperitoneal space but not into the peritoneal cavity.
The kidneys drain urine into the ureters by gravity flow and the ureters provide peristaltic action to move urine along to the bladder where it is stored. The two principal parts of the bladder are the body and the neck. The body stores urine and is made up of smooth muscle known as detrusor muscle. Detrusor muscle extends in all directions throughout the bladder and contracts as a unit in response to initiation of action potentials. The urinary bladder collects 300 to 500 ml before stretch receptors signal a need for bladder emptying. Urine is drained from the bladder by the urethra when the internal and external sphincters are relaxed. Innervation and control of bladder function are discussed in detail in Chapter 29.
An adult kidney weighs approximately 115 to 170 g; is 11 cm long, 6 cm wide, and 3 cm thick; and is shaped like a red kidney bean, with the concave portion, termed the hilum, facing the vertebral column.5 A thin, fibrous capsule covers each kidney and encloses blood vessels, lymphatic vessels, and nerve fibers, including pain receptors. Lymphatic vessels, blood vessels, and nerves enter and exit the kidney through the hilum.
Renal Parenchyma
On cross-section, the kidney is seen to contain three principal areas: the pelvis, the medulla, and the cortex (Figure 26-2). The renal pelvis is a large collecting area for the urine that drains from the many collecting ducts of the nephrons. The minor (smaller) calices collect urine as it drains from the papilla of the renal pyramids. The normal kidney has 8 to 18 minor calices and 2 to 3 major calices.1 The major calices are large collecting spaces located between the minor calices and the upper part of the ureter.
The medulla contains 8 to 18 renal pyramids, the bases of which are adjacent to the outer cortex, whereas the apices open into the minor calices. The pyramids consist of collecting tubules, collecting ducts, long loops of Henle, and vasa recta. The papillae are the openings at the tips of the renal pyramids through which urine exits the collecting ducts.
The renal cortex, which is the outer rim of the kidney, is about 1 cm thick. The cortex contains all of the glomeruli as well as 85% of the nephron tubules. Fifteen percent of nephrons send their loops of Henle deep into the medulla and are called juxtamedullary nephrons. Columns of cortical tissue are found between the medullary pyramids and provide the passageway for the interlobar arteries.
Renal Lymphatics and Innervation
There are two lymphatic systems in the kidney. One system is composed of vessels that are located both in the renal capsule and immediately under the capsule in the outer cortex. The other lymphatic system is composed of vessels that accompany and wrap around the arterial blood vessels. All the lymphatic vessels, as well as blood vessels and nerves, exit the kidney through the hilum, and lymph drains into the paraaortic lymph nodes.
The kidneys are innervated by the sympathetic division of the autonomic nervous system. The lesser splanchnic nerves come from the renal plexuses, which are located next to the renal arteries. These nerve fibers travel with the renal arterial blood vessels and terminate in smooth muscle of the afferent and efferent arterioles, proximal and distal tubules, and the renin-secreting juxtaglomerular cells.1,5 Stimulation of the sympathetic nervous system results in renal vasoconstriction and renin release. The renal capsule and all structures between the renal pelvis and urinary meatus are innervated with pain receptors (see Chapter 27).
Renal Blood Supply
Approximately 25% of the cardiac output is delivered to the kidneys, the majority of which circulates through the cortex, while only 1% to 2% perfuses the medulla.5 Total renal blood flow in both kidneys is approximately 1250 ml/min. Blood flows to the kidneys from the abdominal aorta through the renal arteries, which then divide into several interlobar arteries.5 The interlobar arteries travel in the renal columns adjacent to the pyramids (see Figure 26-2). When the interlobar arteries reach the border of the medulla and the cortex, they branch into the arcuate arteries. The arcuate arteries then travel along the cortical medullary border parallel to the renal capsule. The arcuate arteries branch further to form small interlobular arteries, which penetrate the cortex and branch extensively to form the afferent arterioles. The afferent arterioles divide to form glomerular capillaries, which coalesce to form the efferent arterioles (Figure 26-3). The efferent arterioles branch again to form a second capillary bed. The peritubular capillaries wrap around the proximal and distal convoluted tubules (Figure 26-4). Some capillaries, called vasa recta, dip down into the medulla to surround the loops of Henle and collecting ducts. The vasa recta have a specialized loop structure that enables them to pick up interstitial fluid without excessive removal of interstitial solutes. Solutes and water move into and out of the vasa recta passively such that the descending limb gains solute as it dips into the highly concentrated medulla, but then most of the solute is lost as the ascending loop makes its way back up to the cortex.
The capillaries of the peritubular system and the vasa recta join together and drain into interlobular venules. The veins that drain blood from the kidney run parallel to the arteries and are similarly named (Figure 26-5).
Overview of Nephron Structure and Function
Most of the physiologic functioning of the kidney can be understood by examining the function of an individual nephron. Thus, the nephron is said to be the functional unit of the kidney. Nephrons are organized in parallel such that each must accomplish all the necessary processing before releasing urine into the collecting ducts. Complex autoregulatory mechanisms ensure that the workload is evenly distributed among the kidneys’ many nephrons.
As the unit of kidney function, a nephron must accomplish three major functions: (1) filtration of water-soluble substances from the blood; (2) reabsorption of filtered nutrients, water, and electrolytes; and (3) secretion of wastes or excess substances into the filtrate. Different segments of the nephron are specialized to accomplish each of these processes. Each nephron is composed of a glomerulus, which includes the capillary tuft and Bowman capsule, and a tubule, which includes the proximal convoluted tubule, loop of Henle, distal convoluted tubule, and collecting tubule (Figure 26-6). The nephron tubule is composed of a single layer of epithelial cells with an apical side facing the lumen and a basolateral side facing the interstitial space and capillaries (Figure 26-7). The epithelial cells in each segment of the tubule are specialized for certain functions (Table 26-1). Nearly all cells in the nephron have a single cilium that protrudes from the apical surface into the lumen of the tubule. These cilia are mechanoreceptors and chemoreceptors that sense flow rate and composition of the tubular filtrate. Stimulation of the cilium triggers signaling cascades within the tubule cells that regulate cell proliferation, differentiation, and apoptosis. Abnormalities in cilia signaling function have been found in patients with polycystic kidney disease, associated with mutations in genes (polycystin, PKD1, PKD2) that code for cilia membrane proteins (see Chapter 27).
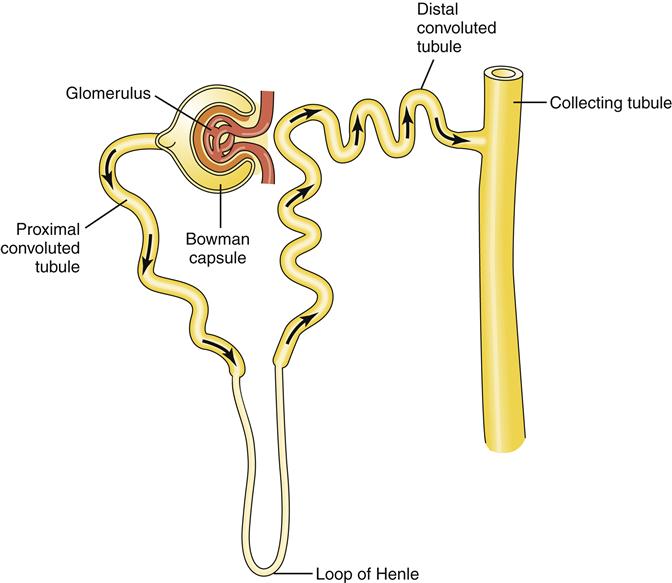
Filtration occurs at the glomerulus, and the remaining tubule segments perform reabsorption and secretion functions.
TABLE 26-1
FUNCTIONS OF THE NEPHRON SEGMENTS
NEPHRON SEGMENT | FUNCTIONS |
Glomerulus | Filters fluid from blood into Bowman capsule; prevents passage of blood cells and proteins |
Proximal convoluted tubule | Transports two thirds of filtered water and electrolytes and all of the filtered bicarbonate, glucose, amino acids, and vitamins from filtrate to interstitium |
Descending loop of Henle | Transports water; delivers a concentrated filtrate to ascending loop of Henle |
Ascending loop of Henle | Actively transports Na+, K+, Cl− to produce a hypoosmotic filtrate and a high interstitial osmolality |
Distal convoluted tubule | Transports Na+, Cl−, water, and urea; responsive to aldosterone; site of macula densa regulation of GFR; secretes H+ and K+ |
Collecting tubule | Passively transports water under influence of ADH; secretes H+ and K+ |
Glomerulus
The glomerulus is the site of fluid filtration from the blood to the nephron tubule. It is formed by a capillary tuft, which lies between the afferent and efferent arterioles, and by the surrounding epithelial cells of Bowman capsule. The outer layer of the glomerular capsule is called the parietal layer and consists of a single thickness of epithelial cells resting on a layer of basement membrane (Figure 26-8). The inner (visceral) layer of the capsule is composed of specialized epithelial cells called podocytes. Podocytes have foot processes (pedicels) that surround the glomerular capillary walls (Figure 26-9). Between the podocyte and the capillary endothelium is a layer of extracellular matrix called the basement membrane (see Figure 26-8).
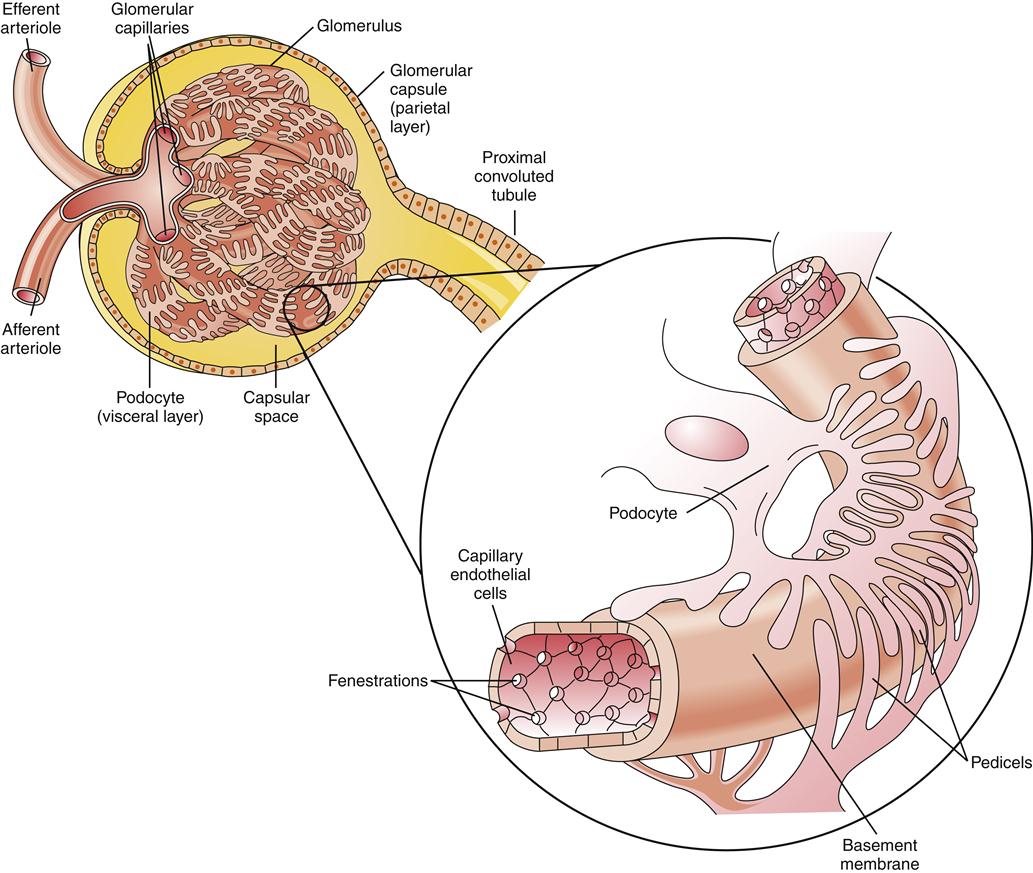
The enlargement shows the glomerular membrane to be composed of the endothelial cells of the capillary, the podocytes of Bowman capsule, and the basement membrane between them.
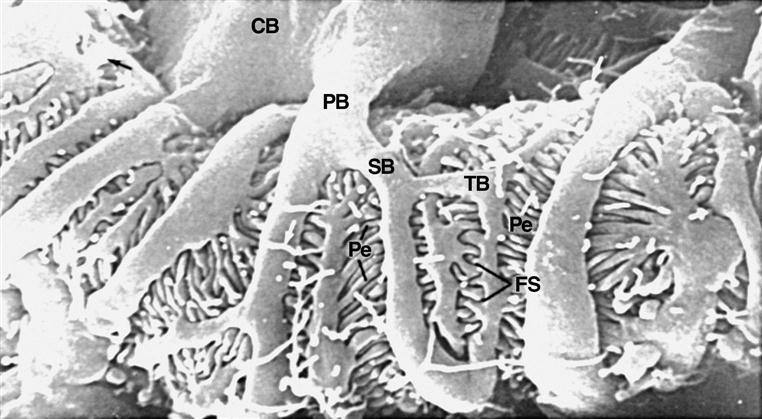
Note the spaces between the podocyte foot processes that contribute to a highly permeable glomerular membrane. CB, Podocyte cell body; PB, primary branch; SB, secondary branch; TB, tertiary branch; Pe, pedicle; FS, filtration slits (slit pores). (From Kessel RG, Kardon RH: Tissues and organs: a text-atlas of scanning electron microscopy, San Francisco, 1979, WH Freeman.)
Spaces between the endothelial cells are called fenestra, and spaces between the podocyte foot processes are called slit pores. These intercellular spaces provide the surface area for glomerular filtration and make the glomeruli considerably more permeable than other capillaries in the body (Figure 26-10). The basement membrane is an important selectivity barrier of the glomerulus, preventing plasma proteins, erythrocytes, leukocytes, and platelets from passing through. Cells are too large to pass through pores, and plasma proteins are negatively charged and repelled to some extent by the basement membrane. Slit pores have a thin diaphragm of extracellular proteins that restricts the filtration of plasma proteins that make it through the basement membrane.5 Nephrin, podocin, NEPH1, CD2AP, and others are important proteins in the slit pores as demonstrated by the proteinuria (protein in urine) that occurs when they are genetically mutated (Figure 26-11).5 Proteins and blood cells are not usually present in the urine. If the glomerulus is injured, blood cells and proteins may filter through and be found in urine. Proteinuria is an important sign of basement membrane dysfunction. Except for the lack of proteins and cells, the glomerular filtrate is very similar in composition to plasma.
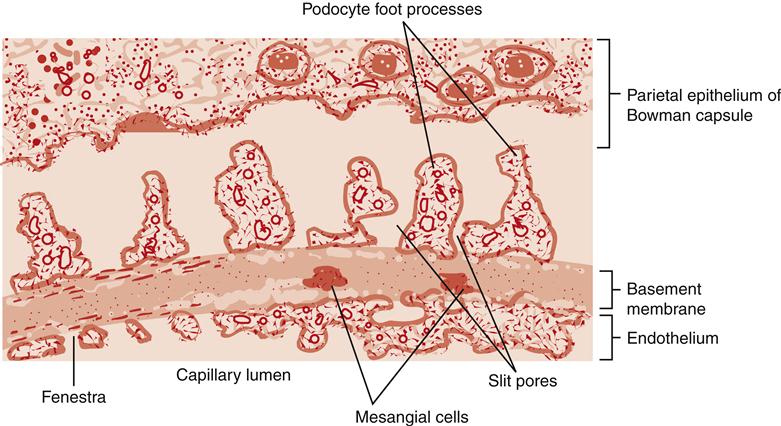
Filtration occurs through the fenestra and slit pores. The basement membrane provides the principal selectivity barrier of the glomerulus.
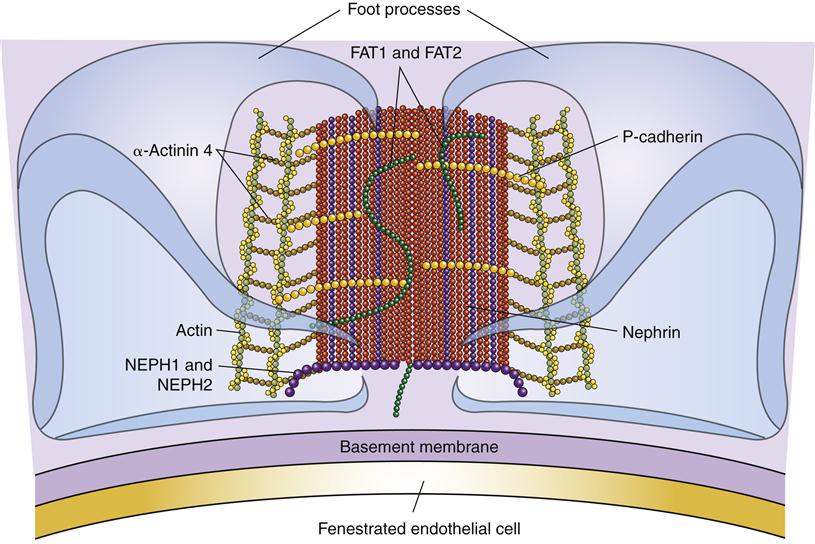
Another important component of the glomerulus is the mesangium, which includes mesangial cells and mesangial matrix. Mesangial cells have a number of functions including provision of structural support for glomerular capillaries, secretion of matrix proteins, phagocytosis, and regulation of the glomerular filtration rate (GFR). By contracting and relaxing, mesangial cells can alter the available surface area for filtration and affect GFR. The GFR averages about 125 ml/min.
Proximal Convoluted Tubule
The Bowman capsule drains the glomerular filtrate directly into the proximal tubule segment, where two thirds of the water and electrolytes are rapidly transported from the filtrate to the interstitium for reabsorption by peritubular capillaries6 (Figure 26-12). Nutrients, vitamins, and small proteins normally are reabsorbed completely in the early proximal tubule. The early proximal tubule is the site of most bicarbonate ion reabsorption, whereas chloride ion is reabsorbed in the late proximal tubule. The proximal tubule consists of cuboidal epithelium that is convoluted to provide a greater surface area for reabsorption. The epithelial cells in this segment have microvilli that form a brush border next to the filtrate and substantially increase the apical surface area. Proximal tubule cells have high adenosine triphosphate (ATP) requirements because most reabsorption utilizes active transport mechanisms that are dependent on Na+-K+ ion pumps in the basolateral membrane. Details of some of these transport mechanisms are discussed in subsequent sections. Water is reabsorbed passively through paracellular transport between the tubular cells and through water channels in the tubule cell membranes made of proteins called aquaporin 1. Reabsorption of solutes creates the osmotic force for passive water reabsorption.
Loop of Henle
The loop of Henle is divided into the descending and ascending limbs, which differ significantly in structure and function. The descending limb receives filtrate from the proximal convoluted tubule and delivers it to the ascending limb. The thin descending limb is permeable to water, but the thin and thick ascending part of the loop is not.6 The thick ascending segment contains powerful membrane pumps that cotransport ions (Na+, K+, 2Cl−) from the filtrate and deposit them in the interstitial fluid surrounding the loops of Henle and collecting ducts (Figure 26-13). About 15% of nephrons have extra-long loops of Henle that dip down into the medulla (juxtamedullary nephrons). These nephrons are vital for creating concentrated urine.
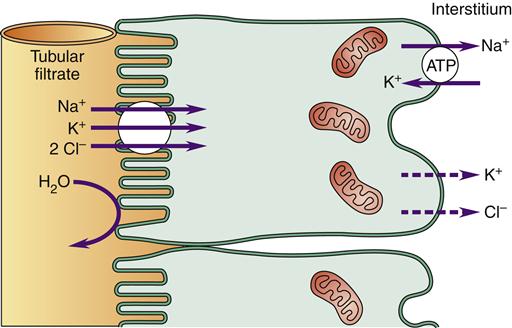
The Na+ is then pumped out of the basolateral membrane and into the interstitium. The loop of Henle ion cotransporter is responsible for creating a highly concentrated medullary interstitium.
The loop formation of the loop of Henle creates a countercurrent mechanism, which allows the ascending loop of Henle to create a high interstitial gradient in the medulla of the kidney (Figure 26-14). Because the ascending loop is impermeable to water, water cannot follow the Na+, K+, and Cl− ions that are pumped into the interstitium. The descending loop is permeable to water, and water will be drawn out by the extra ions that were pumped into the interstitium by the ascending limb. Thus, the filtrate that reaches the ascending limb will be more concentrated than the original filtrate. Delivery of a more concentrated filtrate to the ascending limb allows the Na+-K+-2Cl− cotransporter to pump out a greater number of ions and reach an even higher interstitial gradient (Figure 26-15). This countercurrent mechanism creates a maximal osmolarity of about 600 mOsm/L at the tip of the loop of Henle as compared with the usual extracellular osmolarity of 280 to 300 mOsm/L at the cortex. Another 600 mOsm/L is contributed by the accumulation of urea particles in the interstitium.6 Urea moves passively from the filtrate into the interstitium down its concentration gradient. Urea becomes concentrated in the tubule filtrate when electrolytes and water are removed in the proximal tubule and loop of Henle. An overall interstitial osmolarity is generated that begins in the cortex at about 300 mOsm/L and increases progressively to about 1200 mOsm/L at a point deep in the medulla. This high interstitial osmolarity provides a gradient for water reabsorption from the collecting ducts as they pass through the medulla on their way to the renal pelvis. The maximal interstitial gradient attained is dependent on the length of the loops of Henle. In some animals that survive in dry climates, such as the desert mouse, very long loops of Henle create a much higher interstitial osmolarity, which allows formation of extremely concentrated urine. Fluid that is reabsorbed from collecting tubules into the medullary interstitium is picked up by the specialized capillary network called the vasa recta and returned to the venous circulation. Like other capillaries, the vasa recta passively exchange ions according to concentration gradients and passively reabsorb fluid by filtration forces. The loop structure of the vasa recta allows the capillary to passively leak accumulated solute back into the interstitium as the capillary makes its way back to the cortex from the medulla. This process minimizes the washout of the interstitial osmolality and has been called the countercurrent exchange mechanism.
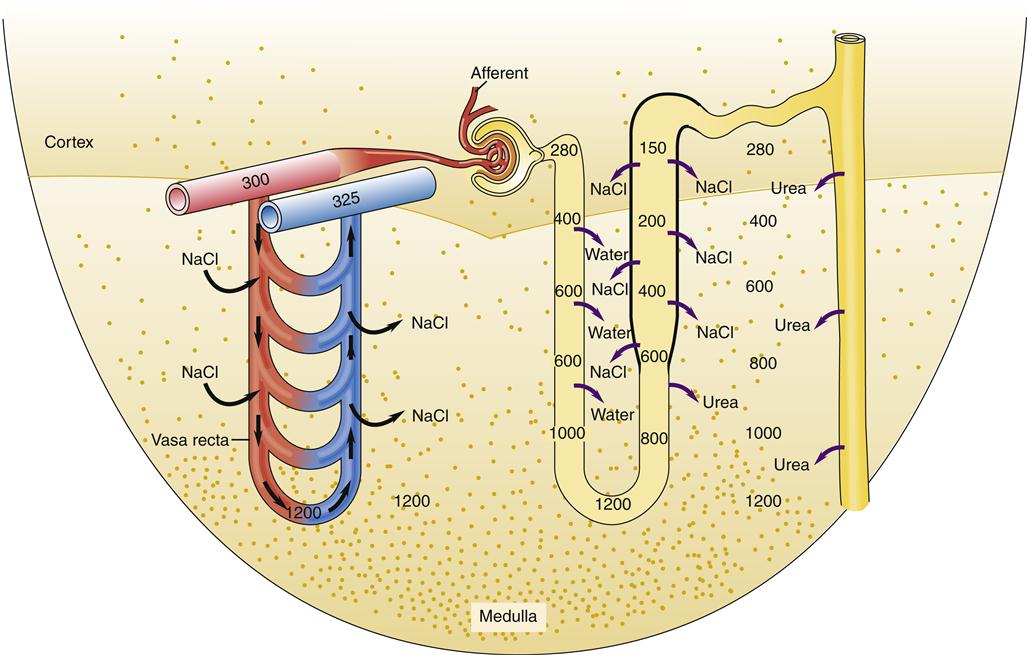
The separation of solute from water in the ascending loop of Henle also produces a dilute tubular fluid allowing the excretion of excess water by making a dilute urine in the absence of antidiuretic hormone (ADH). NaCl accumulation in the interstitium contributes about half of the total osmolality. Urea particles in the interstitium contribute the other half of the particles that produce the normal interstitial gradient in the medulla. As water is removed from the collecting duct (in the presence of ADH), urea becomes more concentrated and moves passively down its gradient into the interstitium. The specialized loop structure of the vasa recta allows it to pick up interstitial water from the medulla without significant solute removal. Although solutes are acquired in the descending segment of the vasa recta, they passively diffuse back out as the ascending segment reaches the cortex. This process is called countercurrent exchange.
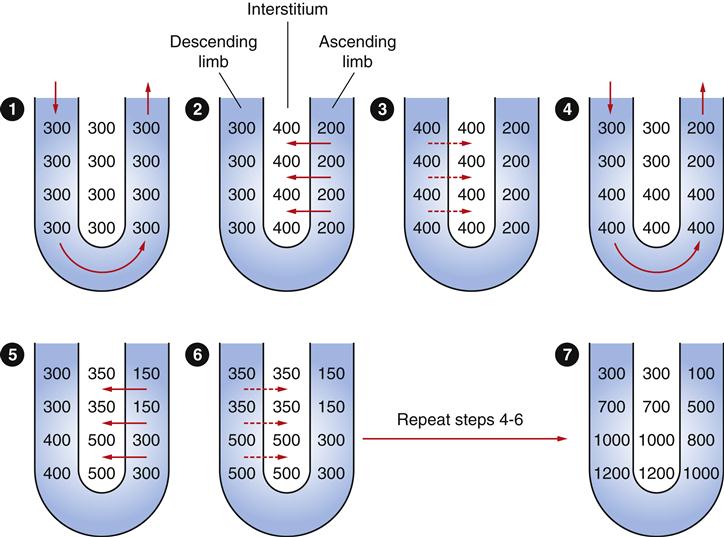
The Na+-K+-2Cl− cotransporters in the thick ascending loop of Henle can produce a gradient across the tubule wall of about 200 mOsm/L. The overall interstitial solute gradient is higher than these pumps could accomplish without the loop structure of the tubule. Countercurrent multiplication occurs because the descending loop is permeable to water and equilibrates with the rising interstitial solute concentration. Thus the filtrate reaching the ascending loop is increasingly concentrated with each step (1-7), allowing the ascending loop to further increase the osmolality of the interstitial fluid.
Distal Convoluted Tubule
The filtrate that reaches the distal tubule is normally hypoosmotic (100 mOsm/L) in comparison with plasma (280 mOsm/L) because electrolytes have been removed by the pumps in the ascending loop of Henle.7 At this point in the nephron, only 10% of the original glomerular filtrate volume remains, and further reabsorption in the distal tubule is largely under hormonal control. Aldosterone and angiotensin II (AII) stimulate the tubule cells to reabsorb sodium and water, whereas atrial natriuretic peptide (ANP) and urodilatin inhibit reabsorption.
Collecting Duct
The distal tubules of several nephrons empty into a single collecting tubule, which then merges into progressively larger and fewer collecting ducts that run parallel to the loops of Henle. Eventually the collecting ducts form the medullary pyramids, which empty into the minor calices through the papilla. The collecting ducts travel through the high interstitial gradient of the medulla on their way to the renal pelvis. The collecting ducts have two cell types called principle cells (P cells) and intercalated cells (I cells). The majority of cells are the P type that responds to antidiuretic hormone. In the presence of antidiuretic hormone (ADH), more than 99% of the original filtrate is reabsorbed by the time it reaches the renal pelvis, creating 30 to 60 ml of concentrated urine per hour. The I cells participate in acid-base balance by regulating the secretion of acid.
Regulation of Glomerular Filtration
The GFR is determined by the filtration pressure in the glomeruli and by the permeable surface area of the glomerular membrane (Kf). Filtration pressure varies considerably from the afferent end of the glomerulus to the efferent end and is difficult to measure directly. The average net filtration pressure for the capillary as a whole is about 10 mm Hg, and the permeability constant Kf is about 12.5 ml/min per mm Hg. GFR is the product of filtration pressure and Kf (10 mm Hg × 12.5 ml/min per mm Hg = 125 ml/min).1,5 The GFR is determined by the physical principles of filtration across a capillary membrane (Figure 26-16). These values are not easily measured in patients, so a global assessment of GFR is used clinically to assess renal function (see Tests of Renal Structure and Function); however, understanding the principles that underlie the global clinical measurement is essential to anticipation and prevention of impaired GFR.
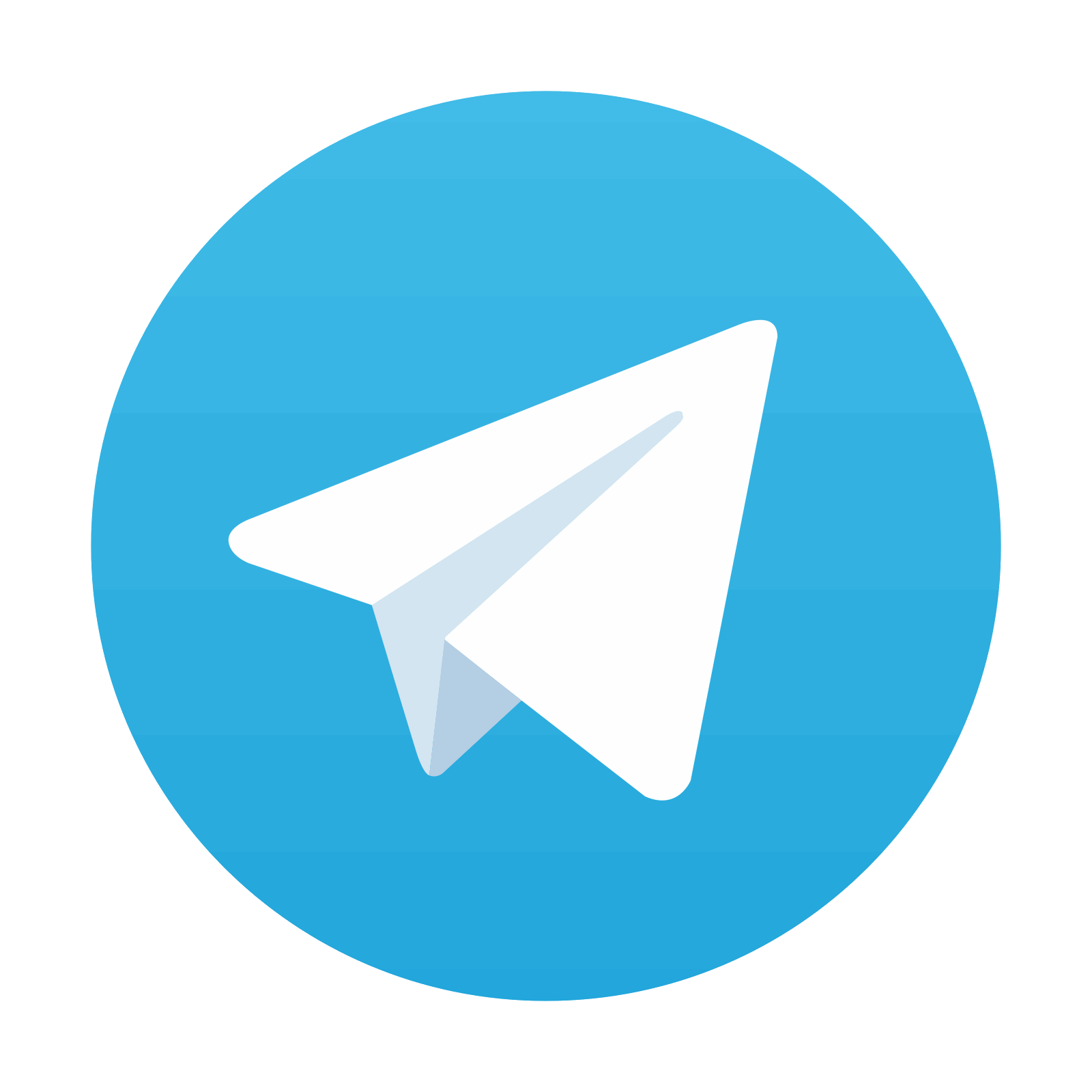
Stay updated, free articles. Join our Telegram channel

Full access? Get Clinical Tree
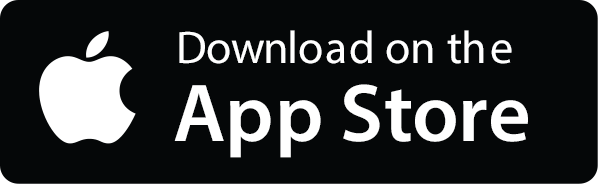
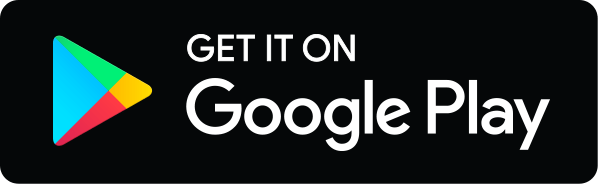