(1)
Canberra, Aust Capital Terr, Australia
Scientific Name
Echinacea purpurea (L.) Moench
Synonyms
Brauneria purpurea (L.) Britton, Echinacea intermedia Lindl. ex Paxton, Echinacea purpurea var. arkansana Steyerm., Echinacea purpurea f. liggettii Steyerm., Echinacea purpurea var. serotina (Nutt.) L.H. Bailey, Echinacea serotina (Sweet) D. Don ex G. Don f., Echinacea speciosa (Wender.) Paxton, Helichroa purpurea (L.) Raf. Rudbeckia purpurea L.
Family
Asteraceae
Common/English Names
Black Sampson, Comb Flower, Coneflower, Eastern Purple Coneflower, Echinacea, Indian Head, Missouri Snakeroot, Purple Coneflower, Red Sunflower.
Vernacular Names
Brazil: Equinácea, Equinocea, Flor De Cone (Portuguese)
Chinese: Zi Hua Song Guo Ju, Zi Zhui Ju
Czech: Třapatka Nachová
Danish: Have-Purpursolhat, Purpur Solhat, Solhat
Dutch: Kogelbloemsoort, Purperen Rudbeckia, Rode Zonnehoed
Eastonian: Purpur-Siilkübar
Finnish: Auringonhattu, Kaunopunahattu, Punahattu
French: Echinacée, Echinacée Pourpre, Rudbeckie Pourpre
German: Echinacea, Echinacin, Echter Sonnenhut, Kegelblume, Purpurroter Sonnenhut, roter Scheinsonnenhut, Roter Sonnenhut, Rudbeckie, Sonnenhut
Hungarian: Bíbor Kasvirág, Bíbor Kúpvirág, Lángvörös Kasvirág, Piros Kasvirág
Icelandic: Echinacea, Sólhattur
Italian: Echinacea Purpurea
Norwegian: Echinacea, Purpursolhatt, Rød Solhatt
Polish: Jeżówka Purpurowa, Jeżogłówka Purpurowa, Rudbekia Purpurowa
Portuguese: Equinácea Purpúrea
Russian: Echinacija purpurovaja
Slovašcina: Ameriški Slamnik, Purpurni Ameriški Slamnik, Škrlatni Ameriški Slamnik
Spanish: Echinacea
Swedish: Purpurrudbeckia, Röd Rudbeckia, Röd Solhatt
Turkish: Ekinazya, Güneş Çiçeği, Kipriciceği, Kipriotu
Origin/Distribution
Eastern Purple Coneflower is native to eastern North America. It is present to some extent in the wild in much of the eastern, southeastern and midwest United States.
Agroecology
In its native temperate range, it occurs in open woodlands, thickets, prairies, near waterways and roadsides. It is extensively grown as ornamentals in gardens and parks and is also cultivated commercially as an herbal remedy.
E. purpurea thrives best in full sun and is shade intolerant. It is not fastidious of soil pH but plant prefers loamy or sandy, well-drained soils. It is also quite drought tolerant.
Edible Plant Parts and Uses
The petals are edible. Some dishes include echinacea Pane bagno (bathed bread) decorated with fresh echinacea petals and a wedge of lemon, American Indian savoury echinacea spread, echinacea and melon fruit salad (Roberts 2000). Petals are fried with watercress, onion and mustard leaves and spread over sweet potatoes.
Botany
A herbaceous perennial 5–120 cm high with erect, branched brownish-green glabrous or hairy stems (Plate 1) and fibrous roots. Leaves alternate, simple and lower leaves broader ovate (Plate 2) and petiolate with weakly winged petioles, lengths decreasing in upper leaves, lamina ovate lanceolate to narrolyl lanceolate, 5–30 by 5–12 cm, margins serrate, apex acute, base rounded to acute. Flowering heads solitary on stout terminal peduncle, 8–25 cm (Plates 1, 3 and 4). Involucral bracts linear to lanceolate. Receptacle with 9–15 mm palea, red orange tipped, slightly curve and pointed. Discs conic to subglobose 1.4–45.5 by 2–4 cm, disc florets corolla greenish to pink or purple, 1.5–4.5 × 2–4 cm. Ray florets corolla pink to purple pink to purple, laminae spreading to recurved, 3–8 cm × 0.8–2 cm, two-toothed at apex, sparsely hairy abaxially (Plates 1, 3 and 4). Cypselae, 3–4 angled, glabrous, tan or bicoloured with a dark brown distal band, pappi persistent, 1.2 mm with 0–4 prominent teeth.





Plate 1
Echinacea in flowers and lanceolate upper leaves

Plate 2
Broadly ovate juvenile leaves

Plate 3
Close view of flowers with conic discs

Plate 4
Flowers with subglobose and flattish round discs
Nutritive/Medicinal Properties
Plant/Cell Culture Phytochemicals
Traces of pyrrolizidine alkaloids (0.006 %) tussilagine and isotussilagine were found in dried materials of Echinacea angustifolia and E. purpurea (Roder et al. 1984).
A 35-kDa water-soluble, acidic polysaccharide, 4-O-methyl-glucuronoarabinoxylan, was isolated from hemicellulosic fraction of E. purpurea and characterized by Wagner et al. (1984, 1985) and Proksch and Wagner (1987). The polysaccharide contained a (1 → 4)-linked β-d-xylan backbone with branching points at C-2 and C-3. Three homogeneous polysaccharides, two neutral fucogalactoxyloglucans with mean molecular weight of 10,000 and 25,000 and an acidic arabinogalactan with a mean molecular weight of 75,000 were isolated from the medium of Echinacea purpurea cell cultures (Wagner et al. 1988). An arabinogalactan protein (AGP) with molecular weight 1.2 × 106 Da, from pressed juice of Echinacea purpurea, was isolated from a high molecular weight fraction by precipitation with the β-glucosyl Yariv reagent (Classen et al. 2000). It comprised a high amount of polysaccharide (83 %) with a ratio of galactose to arabinose of 1.8:1, some uronic acids (4–5 %) and a low-protein content (7 %) with high levels of serine, alanine and hydroxyproline. The amino acid profile in the arabinogalactan protein comprised serine 16 %, alanine 12.5 %, hydroxyproline 11.5 %, asparagine/aspartic acid 10.6 %, threonine 10.5 %, glutamine/glutamic acid 9.4 %, arginine 7.5 %, glycine 4.6 %, valine 4.1 %, histidine 3.7 %, lysine 3.3 %, leucine 2.5 %, isoleucine 2.2 % and phenylalanine 1,6 %. The monosaccharide profile in the arabinogalactan protein comprised galactose 59.1, arabinose 33.2, glucosamine 4.0 %, mannose 2.6 % and rhamnose 1.1 %. The molecular weight arabinogalactan protein (AGP) from the pressed juice of Echinacea purpurea was found to have hydroxyproline (42.9 % w/w) as the dominant amino acid and the major amino acid responsible for the binding between the protein and the arabinogalactan subunits via an O-glycosidic linkage (Volk et al. 2007). Large amounts of glutamine/glutamic acid (24.5 % w/w) and asparagine/aspartic acid (17.3 % w/w) were also found. Another arabinogalactan protein was purified from E. purpurea suspension culture (Classen 2007). It comprised high amount of polysaccharide (90 % w/w) with the dominating monosaccharides galactose and arabinose and some glucuronic acid and a small protein moiety (10 % w/w) with the main amino acids alanine, hydroxyproline, serine, glutamine/glutamic acid, asparagines/aspartic acid and threonine. The polysaccharide part was composed of a branched core-polysaccharide of 3-, 6- and 3,6-linked Galp residues with terminal Araf, Arap, Galp and GlcAp residues. Compared to an arabinogalactan protein from pressed juice of the aerial parts of Echinacea purpurea, differences particularly in terminal arabinose mono- and oligosaccharides in arabinogalactan (AG) side branches could be detected.
Two groups of minerals (I, Fe, Cu, Mn, Li and, II, Ca, Mg, Zn, Ni) were identified in the aerial parts and roots of E. purpurea in Serbia (Razić et al. 2003). The trace element profiles in the roots, stem, leaves and flowers were found to differ significantly.
The aerial parts of Echinacea purpurea afforded, in addition to known compounds, five highly unsaturated acetylenic amides and a derivative of linolen (Bohlmann and Hoffmann 1983). The aerial parts of E. purpurea were found to contain different structural types of alkamides; the roots yielded similar alkamide pattern (Bauer and Reminger 1989). Alkamide levels differed significantly among roots, rhizomes, stems, leaves and flowers of E. purpurea (Perry et al. 1997). Roots were distinguished from other plant parts by higher levels of the C12 diene-diyne alkamides, whereas levels of the C12 tetraene alkamides and C11 diene-diynes were highest in vegetative stems. The ratio of the 2 stereoisomeric C12 tetraene alkamides differed between flowers and all other E. purpurea parts. The alkylamides and cichoric acid in dried roots and aerial parts of E. purpurea grown in eastern Australia were determined as follows: total alkylamide concentration in root samples was 6.2 mg/g (range 1.2–12.1 mg/g) and in aerial samples was 1.0 mg/g (range 0.2–3.9 mg/g) (Wills and Stuart 1999). The cichoric acid concentration in root samples was 13.2 mg/g (range 1.4–20.5 mg/g) and in aerial samples was 12.9 mg/g (range 4.9–21.4 mg/g). Stuart and Wills (2000) found that total alkamide concentration in E. purpurea root, stem and leaf decreased throughout the first growing season while the concentration in flowers increased. In mature plants, the root contained about 70 % of the total plant alkamides with approximately 20 % in flower, 10 % in stem and 1 % in leaf tissue. The relative proportion of individual alkamides in the root did not change during plant growth, but cichoric acid concentration in plant tissues did decrease during plant senescence. Similar concentrations of cichoric acid were measured in root, flower and leaf tissues, but stem levels were lower. In mature plants, the flower and leaf each contained about 35 % of the total plant cichoric acid, while the root and stem contained approximately 20 and 10 %, respectively. Cichoric acid was the main phenolic in E. purpurea roots (mean 2.27 % summer, 1.68 % autumn) and tops (2.02 % summer, 0.52 % autumn) followed by caftaric acid was the other main phenolic compound in the roots (0.40 % summer, 0.35 % autumn) and tops (0.82 % summer, 0.18 % autumn) (Perry et al. 2001). Autumn-grown Echinacea purpurea plants in Taiwan produced more caffeoyl phenols, particularly cichoric acid and caftaric acid, in leaf and flower tissues than spring-grown plants (Chen et al. 2008). Iranian cultivated E. purpurea tops was found to have a high content of cichoric acid (3.5–5.7 %), followed by caftaric acid (3.1–4.5 %) and caffeic acid (0.6–1.1 %) with total polyphenol content of 7.9–10.9 %, (Iranshahi and Amanzadeh 2008). After 2 hours of boiling water extraction, the content of cichoric acid was 5.7 %, whereas the content of this acid in 60:40 ethanol–water extraction did not exceed 3.9 %.
For total alkamides, concentrations (mg/g dry weight basis) among individual E. purpurea plants varied from 5.02 to 27.67 (mean = 14.4 %) in roots, from 0.62 to 3.42 (mean = 1.54) in nearly matured seed heads, and from 0.22 to 5.25 (mean = 0.77) in young tops (about ½ flower heads, ¼ leaves, and ¼ stems) (Qu et al. 2005). For cichoric acid, concentrations among individual plants varied from 2.65 to 37.52 (mean = 8.95), from 2.03 to 31.58 (mean = 10.9) and from 4.79 to 38.55 (mean = 18.88) in the roots, the seed heads and the tops, respectively. Dodeca-2E, 4E, 8Z, 10E-tetraenoic acid isobutylamide and dodeca-2E, 4E, 8Z, 10Z-tetraenoic acid isobutylamide (alkamides 8/9) accounted for only 9.5 % of the total alkamides in roots but comprised 87.9 % in the seed heads and 76.6 % in the young tops. Two cinnamic acids, 2-O-caffeoyl-3-O-isoferuloyltartaric (3) and 2, 3-di-O-isoferuloyltartaric acid (5), along with three known caffeic acids, cichoric acid (1), 2-O-caffeoyl-3-O-feruloyltartaric acid (2) and 2-O-caffeoyl-3-O–p-coumaroyltartaric acid (4), were isolated and purified from Echinacea purpurea (Lu et al. 2012). From 250 mg of crude extracts, 65.1 mg of 1, 8.3 mg of 2, 4.0 mg of 3, 4.5 mg of 4 and 4.3 mg of 5 were isolated, with purities of 98.5, 97.7, 94.6, 94.3 and 98.6 %, respectively.
Nineteen phenolics were identified in the medium of E. purpurea cell cultures after elicitation with biotic elicitors (Li and Barz 2005, 2006). The medium contained lignan, neolignan and acetophenone derivatives as the main elicitor-enhanced products. E. purpurea cells mainly contained phenolic glycosides including a new compound α-O-β-d-glucopyranosyl-acetovanillone. Constitutive compounds identified in the cell culture medium of E. purpurea included α-hydroxy-acetovanillone (1), coniferyl alcohol (4), methyl (E)-p-coumarate (7), and 1-hydroxypinoresinol (8). After elicitation of E. purpurea cell culture with yeast, the compounds found in the medium included α-hydroxyacetosyringone (2); 4-hydroxy-acetophenone (3); 4,7,9,8′-tetrahydroxy-3,3′-5′-trimethoxy-8,4′-oxy-9′-norneolignan-7′-one (5); 1-(4-hydroxy-3-methoxyphenyl)-2-(2-methoxy-4-[1-(E)-propen-3-ol]-phenoxy]-propane-1,3-diol (6a,b); 4,7,9-trihydroxy-3,3′,5′-trimethoxy-8,4′-oxy-9′-norneolignan-7′-one (9); and buddlenol B (10). Phenolics found in E. purpurea cells were 4-hydroxyacetophenone (3); scopoletin (11); 4-hydroxybenzoic acid glucose ester (12); 4-O-β-glucopyranosylacetophenone (13); α-O-β-d-glucopyranosyl-acetovanillone (14); 4-O-β-glucopyranosylconferyl alcohol (15); scopoline (16); 1-(4-O-B-glucopyranosyl-3-methoxyphenyl)-2-(2-methoxy-4[1-(E)-propen-3-ol]-phenoxy]-propane-1,3-diol (17a,b); 4(O-β-d-glucopyranosyl)-7,9-dihydroxy-3,3′,5′-trimethoxy-8,4′-oxy-9′-norneolignan-7′-one (18); 1-(4-hydroxy-3-methoxyphenyl)-2-(2-methoxy-4-[1-(E)-propen-3-ol]-phenoxy]-propane-1,3-diol (6a,b); and longiloroside (19). The optima accumulation of Echinacea purpurea suspension culture biomass (73.6 g/l FW and 10.03 g/l DW), phenolics (61.14 mg/g DW) and flavonoids (38.30 mg/g DW) was achieved in 0.5 MS (Murashige and Skoog) medium (Wu et al. 2007b). High adventitious root biomasses (83.1 g/l FW and 15.30 g/l DW) were achieved with feeding of the 0.5 MS medium at the end of 2nd week. This led to slight decreases in the total production of phenolics and flavonoids; however, this feeding was responsible for increases in the accumulation of caftaric acid (5.76 mg/g DW) and cichoric acid (26.12 mg/g DW).
Beside the above, many other studies had isolated and identified alkamides and caffeic acid derivatives in E. purpurea plant, various plant parts and products (Bergeron et al. 2000; Laasonen et al. 2002; Mølgaard et al. 2003). Ultrasonic extraction of dried samples of E. purpurea roots and aerial parts with methanol–water (7:3) or ethanol–water (7:3) gave good yields of cichoric acid, echinacoside and the alkamides, undeca-2E,4Z-diene-8,10-diynoic acid isobutylamide and a mixture of dodeca-2E,4E,8Z,10E/Z-tetraenoic acid isobutylamides (recoveries of 89, 85, 80 and 90 %, respectively) (Bergeron et al. 2000). The HPLC separation of the phenolic compounds cichoric acid, chlorogenic acid and echinacoside was also improved by careful attention to the pH of the mobile phase. The profile of phenolic compounds (mean concentration μg/ml) in E. purpurea herb was determined as 0–767 μg caftaric acid, 0–45 μg chlorogenic acid, 0–220 μg caffeic acid, 0 μg cynarin, 0–2,879 μg cichoric acid, 0 μg echinacoside and 0–25 μg tet 8/9 (dodeca-2E,4E,8Z,10E/Z-tetraenoic acid isobutylamide) (Vimalanathan et al. 2005).
Studies showed that the content of caffeic acid derivatives in E. purpurea aerial parts and roots reached its highest in the middle stage of full blossoming (Liu et al. 2007). The content of caffeic acid derivatives in fresh raw material was generally higher than that in dried raw material. The developmental pattern of total phenolics in E. purpurea was the same as that of caffeic acid derivatives. The stage of mid-bloom was the optimal harvesting period for both caffeic acid derivatives and total phenolics. There was no significant difference in the content of caffeic acid derivatives among three Chinese geographical populations of E. purpurea.
E. purpurea extracts stored for 18 months were found to contain caftaric acid, cichoric acid and undeca-2Z,4E-diene-8,10-diynoic acid isobutylamide at concentrations of 0.7, 0.71 and 2.0 mg/ml, respectively (Cech et al. 2006). Using HPLC/electrospray ionization mass spectrometry, isomeric isobutylamides and 2-methylbutylamides could be distinguished. The cichoric acid was the main phenolic compound detected in dried E. purpurea materials (flowers, leaves, stems and roots), followed by caftaric acid (Lin et al. 2011). The bioactive constituent contents in different plant parts were in the descending order: flowers > leaves > stems > roots. Both caffeic acid derivatives and total phenolics contents were affected by drying method and storage/packing condition. Cool wind-dried materials retained more bioactive constituents content (>85 %) compared to vacuum freeze-dried materials. The storability results indicated that the freeze-dried E. purpurea materials sealed in polyethylene terephthalate/aluminium foil/polyethylene or nylon/polyethylene bags and stored under 10–20 °C and 40–60 % relative humidity without light conditions retained the highest content of bioactive compounds.
Flower Phytochemicals
Volatiles in the headspace of E. purpurea flowers were acetaldehyde, dimethyl sulphide, 2-methylpropanal and acetone, 2-butanone, 2-methylbutanal, 3-methylbutanal, unknown, α-pinene, camphene, hexanal, β-pinene, sabinene/β-thujene, unknown, 2-methyl-4-pentenal, β-myrcene, α-terpinene, limonene, 2-hexenal (trans), ocimene, γ-terpinene, trans-ocimene, p-cymene, α-terpinolene, unknown, 1-hexanol, allo-ocimene, 3-hexen-1-ol (cis), 2-hexen-1-ol (trans) and α-cubebene/α-copaene (Mazza and Cottrell 1999). Major volatiles in headspace of flower tissues were myrcene 43 %, β-pinene 7.4 %, α-pinene 22.6 % and dimethyl sulphide 1.0 %.
The essential oil of E. purpurea flower heads was found to contain 89 components of which 63 (monoterpene hydrocarbons, oxygenated monoterpenes, sesquiterpenes, oxygenated sesquiterpenes) were identified comprising 92.8 % of the total oil yield (Diraz et al. 2012). The major components were germacrene D (11.3 %), caryophyllene oxide (8.7 %), β-caryophyllene (7.2 %) and α-cadinol (6.2 %). The other components higher than 1 % included β-pinene (1.3 %), α-phellandrene (2.9 %), p-cymene (2.65), β-elemene (2.1 %), a-cadinene (1.0 %), naphthalene, 1,2,3,5,6,8a-hexahydro-4,7-dimethyl-1-(1-ethylethyl)-,(1S–cis)- (3.3 %), α-farnesene (1.0 %), 1,5 epoxysalvia-4(14)ene (3.3 %), naphthalene, 1,2,3,4,4a,7-hexahydro-1,6-dimethyl-4-1(1-methylethyl) – (1.6 %), α-bisabolene (2.3 %), bicylo[4.4.0]dec-1-ene,2-isopropyl-5-methyl-9-methylene- (1.8 %), α-cadinene (2.0 %), decatone (1.5 %), ethyl oleate (1.6 %0, vulgarol B (1.9), aromadendrene oxide (1.1 %), 3,4,-difloro-4-methoxybiphenyl (2.7 %), isoaromadendiene epoxide (1.9 %), trans (Z)-α-bisabolene epoxide (2.3 %), benzenepropanoic acid, octadecyl ester (1.2 %) and diepi-α-cedrene epoxide (1.2 %). Earlier, 36 compounds comprising 70.9 % of sesquiterpenes and 6.4 % monoterpene hydrocarbons were identified in the E. purpurea flower-head essential oil in Iran (Mirjalili et al. 2006). Germacrene D (57 %) was the major component.
Rutin and nicotiflorin (3-O-rutinoside campherol) were isolated from the flowers, the latter flavonoid being dominant (Kurkin et al. 2011). The major anthocyanins of Echinacea purpurea and E. pallida were identified as cyanidin 3-O-(β-d-glucopyranoside) and cyanidin 3-O-(6-O-malonyl-β-d-glucopyranoside) (Cheminat et al. 1989). Cyanidin 3-O-(β-d-glucopyranoside) (9.8 mg) and cyanidin 3-O-(6″-O-β-d-glucopyranoside) (14.3 mg) were obtained from 160 mg crude flower extract with a purity of 95.1 and 98.2 %, respectively (Li et al. 2012).
The profile of phenolic compounds (mean concentration μg/ml) in E. purpurea flower was determined as 19–1,212 μg caftaric acid, 0–208 μg chlorogenic acid, 11–179 μg caffeic acid, 0–13 cynarin, 9–734 μg cichoric acid, 0 μg echinacoside, and 0–39 μg tet 8/9 (dodeca-2E,4E,8Z,10E/Z-tetraenoic acid isobutylamide) (Vimalanathan et al. 2005). In Echinacea purpurea cultivars Magnus and White Swan and line CLS-P2, harvested dry flower heads contained highest cichoric acid content and followed by caftaric acid and chlorogenic acid (Chen et al. 2009). The contents of cynarin and echinacoside in flower heads were relatively low as compared to cichoric acid, caftaric acid or chlorogenic acid. Total caffeoyl derivatives content were 125.3, 116.7 and 145.5 mg/g dry weight for line CLS-P2, Magnus and White Swan, respectively. The alkamides dodeca-2E,4E,8Z,10E-tetraenoic acid isobutylamide (alkamide 8) and dodeca-2E,4E,8Z,10Z-tetraenoic acid isobutylamide (alkamide 9) were also found in the flower heads, but their content was much lower than in the roots. No statistically significant differences in flower-head alkamides 8 and 9 content were found among the tested E. purpurea cultivars and lines.
The extraction yields of phenolics of freeze-dried E. purpurea flowers were significantly affected by the ethanol concentrations at 25 °C with water extraction giving the highest extraction yield (39.8 %) (Tsai et al. 2011). When the ethanol volume percentage in the solvent was increased from 25 % up to 95 %, the extraction yields were decreased from 36.9 to 3.3 %. Water extraction yielded 1,656 mg/g extract of caftaric acid, 0.14 mg chlorogenic acid, 0.53 mg echinoside, 37.23 mg cichoric acid, total caffeic acid derivatives 54.45 mg and total phenolics 97.07 mg. For caftaric acid, ethanol extraction (50 %) yielded the highest 67.07 mg down to 16.35 mg (ethanol 95 %); for chlorogenic acid, ethanol (75 %) gave 2.84 mg down to 0.53 mg at ethanol 95 %; for echinacoside, ethanol (75 %) gave 1.07 mg down to 0.54 mg at ethanol 95 %; for cichoric acid, ethanol extraction (50 %) yielded the highest 180.29 mg down to 42.54 mg (ethanol 95 %). For total caffeic acid derivatives, ethanol extraction (50 %) yielded the highest 250.79 mg down to 59.96 mg (ethanol 95 %). For total phenolics, ethanol extraction (50 %) yielded the highest 441.33 mg down to 104.47 mg (ethanol 95 %). The total phenols, individual and total caffeic acid derivatives contents of extracts were enhanced by elevated extraction temperatures ranged from 25 to 65 °C.
The content of cichoric acid and caftaric acids in dried E. purpurea flowers were found to be significantly affected by the drying methods used (Kim et al. 2000b). Although significant loss of cichoric acid was observed when flowers were stored at high moisture, vacuum microwave-dried flowers with a low-moisture content retained the highest levels of cichoric acid and caftaric acid similar to freeze-dried flowers. Flowers that were air-dried at 25 °C retained about 50 %, while those air-dried D at 70 °C had lowest retention of these acids. Although flowers air-dried at 40 °C retained relatively high amounts of cichoric acid and caftaric acid, the time (55 hours) required to reach optimal drying was considerably longer than that (47 minutes) for vacuum microwave drying. They also found that individual alkamide concentrations in roots and leaves were affected by the drying methods used (Kim et al. 2000a). To preserve higher levels of total alkamides, freeze-drying was found to be the best method, vacuum microwave drying was a superior method for drying roots than air-drying at 70 °C, while air-drying at 50 °C was the preferred method for drying leaves of E. purpurea.
Fruit (Achene)/Seed Phytochemicals
Two major alkamide peaks were identified in E. purpurea achenes as undeca-2E,4Z-diene-8, 10-diynoic acid isobutylamide and dodeca-2E,4E,8E,10E/Z tetraenoic acid isobutylamide (8/9) (He et al. 1998). The isomer pair, tetraene 8/9, was purified as a standard for quantification of alkamide content in E. purpurea achenes and roots.
Seed oil yields of E. angustifolia, E. pallida and E. purpurea, harvested in 1998 and 1999, ranged from 13 to 23 % (Oomah et al. 2006). Vitamin E content of the oils ranged from 29 to 85 mg/100 g oil, with α-tocopherol constituting 83 % of the total tocopherol. The oil was highly polyunsaturated and abundant in linoleic, oleic and palmitic acids, together comprising 95 % of the total fatty acids. E. purpurea seed oils contained 66.5 % linoleic, 21.4 %, oleic and 8 % palmitic acids in the 1998 year harvest and 75.6 % linoleic, 12.2 % oleic and 7 % palmitic acids in the 1999 harvest. Fruit (achenes) of E. purpurea was found to contain 33.6 % light yellow fatty oil comprising 78.4 % unsaturated fatty acids and 21.6 % saturated fatty acids; the major components were linoleic acid (58.2 %) and oleic (20.2 %) acid, and the equivalent iodine number was 121 (Vandyshev et al. 2009). Eighteen components were identified in E. purpurea seed oil, comprising 90.4 % of total area with petroleum ether solvent and 96 % with solvent n-hexane solvent (Diraz et al. 2012). The most abundant fatty acids were palmitic acid, stearic acid, oleic acid, and linoleic acid. The fatty acid profile using petroleum ether and n-hexane solvents were, respectively, as follows: oleic acid C18:1 (48 %, 29 %), palmitic acid C16:0 (16.6 %, 9.2 %), linoleic acid C18:2 (13.3 %; 51 %), oxocol C13 (0.1 %, 0 %), myristic acid C14:0 (0.3 %, 0.2 %), pentadecanoic acid C15:0 (0.1 %, 0.1 %), palmitoleic acid C16:1 (1 %, 0.4 %), carbonic acid C17:0 (0.2 %, 0.1 %), heptadecanoic acid C17:1 (0.2 %, 0 %), stearic acid C18:0 (5 %, 2.5 %) elaidic acid C18:1 (0.7 %, 0 %), linolelaidic acid C18:2 (0.8 %, 0 %), arachidic acid C20:0 (0.5 %, 0.6 %), gamma-linolenic acid C18:3 (0 %, 0.5 %), eicosenoic acid C20:1 (0.3 %, 0.5 %), behenic acid C22:0 (1.3 %, 0.7 %), tricosanoic acid C23:0 (0.1 %, 0.1 %), lignoceric acid C24:0 (0.6 %, 0.3 %) and oxiraneoctanoic acid C26 (0.8 %, 0.8 %). Oxocol, heptadecenoic acid, elaidic acid and linolelaidic acid could not be defined with solvent n-hexane.
Leaf Phytochemicals
Volatiles in the headspace of E. purpurea leaf tissues were acetaldehyde, dimethyl sulphide, propanal, 2-methylbutanal, 3-methylbutanal, 2-ethylfuran, pentanl, α-pinene, α-thujene, camphene, hexanal, β-pinene, sabinene/ β-thujene, pentanal, 2-methyl-4-pentenal, β-myrcene, α-terpinene, limonene, 2-hexanal (cis), 2-hexenal (trans), ocimene, γ-terpinene, trans-ocimene, p-cymene, hexyl acetate, α-terpinolene, 3-hexen-1-ol-acetate, 1-hexanol, allo-ocimene 3-hexen-1-ol (cis), α-ylangene, γ-cadinene, trans-caryophyllene, calarene/α-copaene, germacrene D/α-cubebene, 5-ethyl-2(5H)-furanone, δ-cadinene, βα-cubebine/γ-cadinene and 2,2,3,3-tetramethyl hexane (Mazza and Cottrell 1999). Major volatiles in headspace of leaf tissues were myrcene 27 %, β-pinene 1.9 %, α-pinene 12.1 %, dimethyl sulphide <1.0 %
Total phenolic contents reported for E. purpurea (leaf) were 15.15 mg GAE/100 g DW (Wojdyło et al. 2007). Major phenolic compounds (mg/100 g DW) found were phenolic acids, 620 mg caffeic acid, 115 mg neochlorogenic acid, 19.5 mg p-coumaric acid and 17.9 mg ferulic acid, and flavonoids, 12.4 mg quercetin.
In Echinacea purpurea cultivars Magnus and White Swan and line CLS-P2, harvested dry leaves contained highest cichoric acid content and followed by caftaric acid and echinacoside (Chen et al. 2009). The contents of chlorogenic acid and cynarin in leaves were relatively low as compared to cichoric acid, caftaric acid and chlorogenic acid. Among the five caffeoyl derivatives examined, CLS-P2 leaves had greater cichoric acid, caftaric acid, cynarin and echinacoside levels than Magnus and White Swan. Total caffeoyl derivatives content were 61.73, 31.75 and 20.02 mg/g dry weight for line CLS-P2, cultivar Magnus and cultivar White Swan, respectively. Line CLS-P2 had the highest dodeca-2E,4E,8Z,10E-tetraenoic acid isobutylamide (alkamide 8) and dodeca-2E,4E,8Z,10Z-tetraenoic acid isobutylamide (alkamide 9) content in dry leaves among the tested cultivars and line. The profile of phenolic compounds (mean concentration μg/ml) in E. purpurea leaf and stem was determined as 0–1,174 μg caftaric acid, 0–63 μg chlorogenic acid, 0–421 μg caffeic acid, 0–5 cynarin, 0–6,001 μg cichoric acid, 0 μg echinacoside and 0.19 μg tet 8/9 (dodeca-2E,4E,8Z,10E/Z-tetraenoic acid isobutylamide) (Vimalanathan et al. 2005).
Stem Phytochemicals
Volatiles in the headspace of E. purpurea stem tissues were acetaldehyde, dimethyl sulphide, 2-methylpropanal and acetone, 2-methylbutanal, 3-methylbutanal, α-pinene, geranyl acetate, camphene, hexanal, β-pinene, sabinene/ β-thujene, unknown, 2-methyl-4-pentenal, β-myrcene, α-terpinene, limonene, 2-hexenal (trans), ocimene, γ-terpinene, trans-ocimene, α-terpinolene, 3-hexen-1-ol-acetate, 1-hexanol, allo-ocimene, 3-hexen-1-ol (cis), 2-hexen-1-ol (trans), α-cubebene/α-copaene, α-ylangene, α-ylangene, germacrene D/α-cubebene, δ-cadinene and βα-cubebine/γ-cadinene (Mazza and Cottrell 1999). Major volatiles in headspace of stem tissues were myrcene 45 %, β-pinene 4.4 %, α-pinene 33.7 % and dimethyl sulphide <1.0 %.
Root Phytochemicals
Volatiles in the headspace of E. purpurea roots were acetaldehyde, dimethyl sulphide, 2-methylpropanal and acetone, 2-propenal, 2-methylbutanal, 3-methylbutanal, ethanol, 1-methylpropyl acetate, trichloroacetic acid, α-pinene, camphene, hexanal, β-pinene, 2-methyl-1-propanol, sabinene/ β-thujene, α-phellandrene, α-terpinene, heptanal, limonene, 2-methyl-1-butanol, 3-methyl-1-butanol, ocimene, p-cymene, hexyl acetate, unknown, 6-methyl-5-hepten-2-one, 1-hexanol and 1-octen-3-ol, benzaldehyde (Mazza and Cottrell 1999). Major volatiles in headspace of root tissues were 0%, β-pinene 0.2 %, α-pinene 0.6 %, α-phellandrene 16.7 % and dimethyl sulphide 14.7 %. The seventeen lipophilic, volatile to semivolatile components, including the 11 alkamides known to E. purpurea roots, were identified (Hudaib et al. 2002). Cucumber mosaic cucumovirus infection was found to be responsible for significant variations in the relative compositions of the major constituents, in particular germacrene D, dodeca-2E, 4E, 8Z, 10Z(E)-tetraenoic acid isobutylamide cis/trans isomers, undeca-2Z, 4E-diene-8, 10-diynoic acid isobutylamide and dodeca-2E, 4Z-diene-8, 10-diynoic acid isobutylamide.
Root extracts of E. purpurea root were found to contain (μg/ml) chlorogenic acid (0.0157 μg), caftaric acid (0.1568 μg), cafeic acid (trace), cichoric acid (1.0147 μg) alkamides 1 undeca-2E-4Z-diene-8,10-diynoic acid isobutylamide (0.056 μg), undeca-2Z-4E-diene-8,10-diynoic acid isobutylamide (0.0159 μg), doceca-2E,4Z-diene-8,10-diynoic acid isobutylamide (0.0130 μg), undeca-2E,4Z-diene-8,10-diynoic acid 2-methylbutylamide (trace), dodeca-2E,4E,10E-trien-8-ynoic acid isobutylamide (0.0156 μg), dodeca-2E,4E-diene-8,10-diynoic acid 2-methylbutylamide (0.0092 μg), dodeca-2E,4E,8Z,10E-tetraenoic acid isobutylamide (0.2912 μg), dodeca-2E,4E,8Z,10Z-tetraenoic acid isobutylamide (0.0396 μg), dodeca-2E,4E,8Z, trienoic acid isobutylamide (0.0102 μg) and undeca-2E-ene-8,10-diynoic acid isobutylamide (0.0106 μg) (Bauer et al. 1988; Bauer and Reminger 1989; Binns et al. 2002b; Perry et al. 2001; Hall 2003; Senchina et al. 2006; Pietta et al. 1998; Gotti et al. 2002; Pomponio et al. 2002; Solco 2007). No cynarin and echinacoside were detected in the roots. Five alkylamides, undeca-2E,4Z-dien-8,10-diynoic acid isobutylamide, dodeca-2E,4Z-dien-8,10-diynoic acid isobutylamide, dodeca-2E,4E,10E-trien-8-ynoic acid isobutylamide, dodeca-2E,4E,8Z-trienoic acid isobutylamide and dodeca-2E,4Z-dien-8,10-diynoic acid 2-methylbutylamide, were isolated from the roots (Bauer et al. 1988). Nine alkamides were identified in the root of E. purpurea (He et al. 1998) similar to the fingerprint reported by Bauer and Remiger (1989). Several minor alkamides were also tentatively identified.
The following alkamides were identified in fresh and dry root extracts of E. purpurea (Spelman et al. 2009): undeca-2E,4Z-diene-8,10-diynoic acid isobutylamide (A); undeca-2Z,4E-diene-8,10-diynoic acid isobutylamide (B); undeca-2E-ene-8,10-diynoic acid isobutylamide (C); undeca-2E,4Z-diene-8,10-diynoic acid 2-methylbutylamide (D); undeca-2Z,4E-diene-8,10-diynoic acid 2-methylbutylamide (E, new); dodeca-2Z,4E-diene-8,10-diynoic acid isobutylamide (F); dodeca-2E,4Z-diene-8,10-diynoic acid isobutylamide (G); dodeca-2E,4E,10E-triene-8-ynoic acid isobutylamide (H); dodeca-2E,4E,8Z,10E-tetraenoic acid isobutylamide (I); dodeca-2E-ene-8,10-diynoic acid isobutylamide (J, used as standard); dodeca-2E,4E, 8E,10Z-tetraenoic acid isobutylamide (K); dodeca-2E,4E, 8Z,10Z-tetraenoic acid isobutylamide (L, used as standard); dodeca-2E,4E,8Z-trienoic acid isobutylamide (M); dodeca-2E,4E-dienoic acid isobutylamide (N); dodeca-2E,4E-dienoic acid isobutylamide (O); trideca-2E,7Z-diene-8,10-diynoic acid isobutylamide (P); dodeca-2E,4Z-diene-8,10-diynoic acid 2-methylbutylamide (Q); and dodeca-2,4,8,10-tetraenoic acid 2-methylbutylamide (R). Quantities of the isomeric alkylamides K, L and M and alkylamide J in ethanol extracts of E. purpurea root were determined in terms of concentrations of the isomeric tetraenes (compounds K, L and M) and dodeca-2E-ene-8,10-diynoic acid isobutylamide (compound J) per ml of solvent. The three different Echinacea root extracts, fresh 1:2, dry 1:11 and dry 1:5, all contained these alkylamides. However, the dry 1:5 extract contained the greatest amount of these compounds. All three extraction techniques investigated here (fresh 1:2, dry 1:5 and dry 1:11) resulted in very similar alkylamide profile and gave similar yields of alkylamides and of total dissolved solids. The similarity in alkylamide content in fresh 1:2 and dry 1:11 extracts indicated that drying of root material at 50 °C did not result in a loss of alkylamides. It was concluded that either fresh or dried roots could be used to prepare extracts with high alkylamide content, although the overall yield was slightly lower for fresh extracts.
Caffeic acid derivatives, caftaric acid, chlorogenic acid, caffeic acid, cichoric acid and alkamides: undeca-2E,4Z-diene-8,10-diynoic acid isobutylamide; undeca-2Z,4E-diene-8,10-diynoic acid isobutylamide; dodeca-2Z,4E-diene-8,10-diynoic acid isobutylamide; undeca-2E,4Z-diene-8,10-diynoic acid 2-methylbutylamide; dodeca-2E,4E,10E-triene-8-ynoic acid isobutylamide; trideca-2E,7Z-diene-10,12-diynoic acid isobutylamide; dodeca-2Z, 4E-diene-8,10-diynoic acid isobutylamide; dodeca-2E,4Z-diene-8,10-diynoic acid 2-methylbutylamide; dodeca-2E,4E, 8E,10Z-tetraenoic acid isobutylamide, dodeca-2E, 4E,8Z-trienoic acid isobutylamide; and dodeca-2E,4E-dienoic acid isobutylamide, were detected in the roots and extracts (Luo et al. 2003). Three alkamides and nitidanin diisovalerianate were identified, together with 14 known alkamides and one sesquiterpene from the roots of Echinacea purpurea (Hohmann et al. 2011). Cichoric acid and verbascoside predominated in extracts of E. purpurea roots (Sloley et al. 2001). A total of 16 alkamides, three ketoalkenes, two ketoalkynes and four phenolic acids (echinacoside, cichoric acid, caftaric acid and chlorogenic acid) were identified in aqueous ethanol (70 %) root extracts of Echinacea purpurea and Echinacea pallida (Thomsen et al. 2012). The major alkamides in the roots of E. purpurea were at their lowest concentration in the middle of autumn and early winter, while all of the major phenolic acids were at their highest concentrations in spring. In E. purpurea root extract, the major alkamide, dodeca-2E, 4E, 8Z, 10E/Z-tetraenoic acid isobutyl amide, was not significantly affected by storage at any of the temperatures (−20, 25 and 40 °C), but cichoric acid content declined significantly at both 25 and 40 °C as compared to low-temperature storage (Livesey et al. 1999). In the root powder, the major alkamide showed a significantly reduced level at 25 and 40 °C, while cichoric acid did not decline significantly.
Biomass accumulation and production of caffeic acid derivatives (caftaric acid, chlorogenic acid and cichoric acid) in Echinacea purpurea adventitious root cultures was optimal under incubation temperature of 20 °C among the different incubation temperatures tested (10, 15, 20, 25 and 30 °C) (Wu et al. 2007a). Biomass of adventitious roots was highest in cultures grown under dark, while accumulation of caffeic acid derivatives was optimum in the cultures grown under 3/21 hours light and dark cultural regimes. Studies found that 15-day-old hairy root culture stimulated every 5 days by ultrasound for 6 minutes produced the highest amount of caffeic acid derivatives (CADs) after 30 days of culture among all ultrasound treatment experiments (Liu et al. 2012). The obvious increase of CADs production in E. purpurea hairy roots stimulated by ultrasound was related to the increase of both rolB-regulated endogenous indole-3-acetic acid biosynthesis and phenylalanine ammonium lyase (PAL) activity.
In Echinacea purpurea cultivars Magnus and White Swan and line CLS-P2, harvested dry roots contained highest cichoric acid content and then followed by caftaric acid, cynarin, echinacoside and chlorogenic acid (Chen et al. 2009). The caffeoyl derivatives were 16.53, 19.71 and 27.40 mg/g dry weight for CLS-P2, Magnus and White Swan, respectively. Compared to flowers and leaves, roots contained the highest content of alkamides, dodeca-2E,4E,8Z,10E-tetraenoic acid isobutylamide (alkamide 8) and dodeca-2E,4E,8Z,10Z-tetraenoic acid isobutylamide (alkamide 9). White Swan accumulated considerably higher alkamides 8 and 9 content in dry roots than Magnum and line CLS-P2.
Highly water-soluble fructans were isolated from E. purpurea roots (Wack and Blaschek 2006). The fructans represented linear inulin-type fructans with almost exclusively β-(2 → 1)-linked fructosyl units, terminal glucose and terminal fructose. Small proportions of β-(2 → 1,2 → 6)-linked branch point residues were detected. 80 % ethanol-insoluble fructan from E. purpurea showed an average mean degree of polymerization of 35, 60 % ethanol-insoluble fructan of 44, and 40 % ethanol-insoluble fructan of 55.
Commercial Echinacea extracts are manufactured primarily from three Echinacea species, namely, Echinacea purpurea (herb, roots or seeds), E. angustifolia (roots) and E. pallida (roots) (Mahady et al. 2001). Current recommendations for use of these products include oral administration for the prophylaxis and treatment of the common cold, bronchitis, influenza and bacterial and viral infections of the respiratory tract. However, based on existing data products containing pressed juice or hydroalcoholic extracts, Echinacea purpurea (leaf juice and roots) and E. pallida (roots) had the most convincing data supporting their use. According to Spelman et al. (2009), Echinacea purpurea, a top-selling botanical medicine, is currently of considerable interest due to immunomodulatory, antiinflammatory, antiviral and cannabinoid receptor 2 (CB2)-binding activities of its alkylamide constituents. It is an immunostimulating drug, containing multiple bioactive substances such as polysaccharides, caffeic acid derivatives (caffeic acid, cichoric acid, caftaric acid, chlorogenic acid), alkamides and glycoproteins (Manček and Kreft 2005). Among the many pharmacological properties reported for E. purpurea extracts, immunomodulation of macrophages had been demonstrated most convincingly (Barrett 2003). Several dozen clinical studies—including a number of blind randomized trials—had reported health benefits. The most robust data were from studies testing E. purpurea extracts in the treatment for acute upper respiratory infection. Although indicative of modest benefit, these studies were limited both in size and in methodological quality. Although a great deal of moderately good-quality scientific data regarding E. purpurea, effectiveness in treating illness or in enhancing human health exist, much has not yet been proven beyond a reasonable doubt. Numerous studies had shown that E. purpurea exhibited immunostimulating, antimicrobial, antiinflammatory, antioxidant, cytochrome enzyme inhibitory, antiandrogenic, cannabinoidomimetic, radioprotective and antitumorous activities (Gupta et al. 2012).
Antioxidant Activity
The mechanisms of antioxidant activity of extracts derived from E. angustifolia, E. pallida and E. purpurea roots included free radical scavenging and transition metal chelating (Hu and Kitts 2000). Root extracts of these Echinacea spp. were capable of scavenging hydroxyl, DPPH and ABTS radicals. These root extracts delayed the formation of conjugated diene hydroperoxide induced by the thermal decomposition of 2, 2′-azobis(2-amidinopropane) dihydrochloride and protracted the lag phase of peroxidation of soybean liposomes. These root extracts also suppressed the oxidation of human low-density lipoprotein, as evaluated by reduced agarose electrophoretic mobility following oxidative modification by Cu2+.
Studies showed that E. purpurea extracts had antioxidant activity similar to that of ascorbic acid but had no serious effect on inhibiting chicken’s peripheral blood mononuclear cells viability (Lee et al. 2009), In 2,2-diphenyl-1-picril hydrazyl radical (DPPH) scavenging capacity, the ED50 for the extract was measured at 0.23 mg/ml. The superoxide anions scavenging capacity of the extract was nearly equivalent to ascorbic acid (91.1 % vs. 93.0 %) at the same concentration of 1.6 mg/ml, and ED50 was 0.32 and 0.13 mg/ml, respectively. Reducing power of the extract increased linearly with its concentration, and the concentration at 2.0 mg/ml reached about 65 % of ascorbic acid at 0.3 mg/ml. The chelating capacity of ferrous iron (Fe2+) was 70 % as good as that of the synthetic metal chelater EDTA when added to 5.0 mg/ml of E. purpurea extract. The polysaccharides content of the extract was 159.8 mg/g dry weight (DW), and total phenolic compound was 11.0 mg gallic acid equivalent/g DW. Microculture tetrazolium assays showed extracts had 92 % cell viability at 1.6 mg/ml for chicken’s peripheral blood mononuclear cells (PBMCs) and 84 % for RAW 264.7 macrophages, neither reaching the IC50 level.
Extracts of the roots and leaves of E. purpurea were found to have antioxidant properties in a free-radical scavenging assay and in a lipid peroxidation assay (Sloley et al. 2001). The methanol root extract of E. purpurea, E. pallida and E. angustifolia exhibited DPPH antioxidant activity with EC50 values of 134, 167 and 231 μg/ml, respectively (Pellati et al. 2004). The radical scavenging activity of Echinacea root extracts reflected their phenolic content. The total phenolic content was 23.23 mg/g for E. purpurea, 17.83 mg/g for E. pallida and 10.49 mg/g for E. angustifolia. Caftaric acid, chlorogenic acid, caffeic acid, cynarin, echinacoside and cichoric acid were identified and quantified in Echinacea roots and derivatives. Pure echinacoside had the highest capacity to quench DPPH radicals (EC50 = 6.6 μM), while caftaric acid had the lowest (EC50 = 20.5 μM).
The antioxidant activity of three extracts, one alkamide fraction, four polysaccharide-containing fractions, and three caffeic acid derivatives from Echinacea purpurea root was evaluated by measuring their inhibition of in-vitro Cu(II)-catalyzed oxidation of human low-density lipoprotein (LDL) (Dalby-Brown et al. 2005). Among the extracts the 80 % aqueous ethanol extract exhibited ten times longer lag phase prolongation (LPP) than the 50 % ethanol extract, which in turn exhibited a longer LPP than the water extract. The antioxidant activity of the tested Echinacea extracts, fractions and isolated compounds was dose dependent. Synergistic antioxidant effects of Echinacea constituents were found when cichoric acid (major caffeic acid derivative in E. purpurea) or echinacoside (major caffeic acid derivative in Echinacea pallida and Echinacea angustifolia) was combined with a natural mixture of alkamides and/or a water extract containing the high molecular weight compounds.
The extracts of the stems, leaves and roots of Echinacea purpurea and their constituent cichoric acid were found to be efficient scavengers of DPPH radicals with an activity comparable to that of rosmarinic acid, a well-characterized antioxidant (Thygesen et al. 2007). The efficacy of the extracts in the reaction with DPPH correlated well with the amount of cichoric acid present in the various extracts. The alkamides alone showed no antioxidant activity in any of the tests. Alkamides present in the extract increased, however, the antioxidative effect of cichoric acid in the peroxidation lipid emulsion assay. Antioxidant activity in terms of TEAC (μM trolox/100 g DW) of E. purpurea leaves reported was 12.3 μM for ABTS, 75 μM for DPPH and 94.6 μM for FRAP (ferric reducing antioxidant power) assays (Wojdyło et al. 2007). Total phenolic contents reported were 15.15 mg GAE/100 g DW. The DPPH scavenging reached 93.6 %, and the values of EC50 were (34.16) μg/ml and (65.48) μg/ml for the extracts obtained by the classical and ultrasound extractions, respectively (Stanisavljević et al. 2009).
At level of 10 μg/ml, the scavenging abilities of tested samples on DPPH radicals were in the descending order of ascorbic acid > BHA (butylated hydroxyanisole) > flower extract > α-tocopherol (Tsai et al. 2011). At level of 30 μg/ml, the flower extract and α-tocopherol showed 90.82 and 93.10 % scavenging abilities, respectively. The radical scavenging ability of E. purpurea flower extract could be attributable to the caffeic acid derivatives, especially cichoric acid with two adjacent hydroxyl groups of its phenolic rings showed the highest radical scavenging ability. The order of potency against DPPH radicals was the following: echinacoside > cichoric acid > chlorogenic acid > caffeic acid > caftaric acid. At the level of 100 μg/ml, the reducing power of samples was in the descending order of ascorbic acid > BHA > flower extract > α-tocopherol. The ascorbic acid, BHA, and flower extract attained the same maximum reducing power (2.3 AU) at 100, 200 and 400 μg/ml, respectively. The flower extracts exerted an 81.88 % ferrous ions chelating effect at 3 mg/ml concentration when compared with the EDTA (ethylenediaminetetraacetic acid) concentration of 10 μg/ml.
Immunomodulatory Activity
Studies found that the main immunostimulatory activity of Echinacea resided in the water-soluble materials rather than the lipoidal small molecules (Pillai et al. 2007). The use of flow cytometry demonstrated a link between the polysaccharides in Echinacea and the biologic immunostimulatory effect. E. purpurea, E. pallida and E. angustifolia leaves, stems, flowering tops and roots all produced substantial immunostimulatory activity. In-vitro and in-vivo studies indicated that the therapeutic effects of Echinacea were due to a stimulation of cellular immune response (Mahady et al. 2001).
In-Vitro Studies
Purified polysaccharides (EPS) prepared from Echinacea purpurea were shown to strongly activate macrophages that developed pronounced extracellular cytotoxicity against tumour targets (Stimpel et al. 1984). The splenic lymphocytes from mice treated with E. purpurea and Hypericum perforatum at the two dose levels used (30 and 100 mg/kg/day) were shown to be significantly more resistant to apoptosis than those from mice treated only with the vehicle (Di Carlo et al. 2003). Further, mice treated with these natural substances showed a decrease in Fas-Ag expression and an increase in Bcl-2 expression.
The 4-O-methyl-glucuronoarabinoxylan, isolated from E. purpurea showed immune-stimulating activity in several in-vitro immunological test systems (Wagner et al. 1984, 1985; Proksch and Wagner 1987). The fucogalactoxyloglucan of mean molecular weight 25,000 isolated from E. purpurea cell culture enhanced phagocytosis in vitro and in-vivo (Wagner et al. 1988). The arabinogalactan specifically stimulated macrophages to excrete the tumour necrosis factor (TNF). The arabinogalactan protein (AGP) from pressed juice of the aerial parts of Echinacea purpurea demonstrated binding to lymphocytes, monocytes and granulocytes of different donors (Thude et al. 2006). A high molecular weight arabinogalactan protein (AGP) from the pressed juice of Echinacea purpurea, known to exhibit immunomodulatory properties in vitro, was characterized (Volk et al. 2007). Normal human peripheral blood macrophages cultured in concentrations of Echinacea purpurea fresh pressed juice as low as 0.012 μg/ml produced significantly higher levels of IL-1, TNF-α, IL-6 and IL-10 than unstimulated cells (Burger et al. 1997). The high levels of IL-1, TNF-α and IL-10 induced by very low levels of echinacea were consistent with an immune-activated antiviral effect. Echinacea induced lower levels of IL-6 in comparison to the other cytokines measured. Echinacea herb and root powders were found to stimulate murine macrophage cytokine secretion as well as to significantly enhance the viability and/or proliferation of human peripheral blood mononuclear cells in vitro (Rininger et al. 2000). In contrast, echinacea extracts chemically standardized to phenolic acid or echinacoside content and fresh pressed juice preparations were found to be inactive as immunostimulatory agents but did display, to varying degrees, antiinflammatory and antioxidant properties.
Studies showed that E. purpurea root and leaf–stem extract exhibited opposite (enhancing vs. inhibitory) modulatory effects on the expression of the CD83 marker in human dendritic cells (DCs) (Wang et al. 2006) Downregulation of mRNA expression of specific chemokines (e.g., CCL3 and CCL8) and their receptors (e.g., CCR1 and CCR9) was observed in stem- and leaf-treated DCs. Other chemokines and regulatory molecules (e.g., CCL4 and CCL2) involved in the c-Jun pathway were found to be upregulated in root-treated DCs. In another study, following 48 hours exposure of dendritic cells from C57Bl/6 mice to E. purpurea root and leaf extracts, it was found that the polysaccharide-rich root extract increased the expression of MHC class II, CD86 and CD54 surface biomarkers whereas the alkylamide-rich leaf extract inhibited expression of these molecules (Benson et al. 2010). Production of IL-6 and TNF-α increased in a concentration-dependent manner with exposure to the root, but not leaf, extract. In contrast, the leaf but not root extract inhibited the enzymatic activity of cyclooxygenase-2. The leaf but not root extract inhibited the antigen-specific activation of naïve CD4+ T cells from OT II/Thy1.1 mice. These results suggested that E. purpurea could be immunostimulatory, immunosuppressive and/or antiinflammatory depending on the plant part and extraction method.
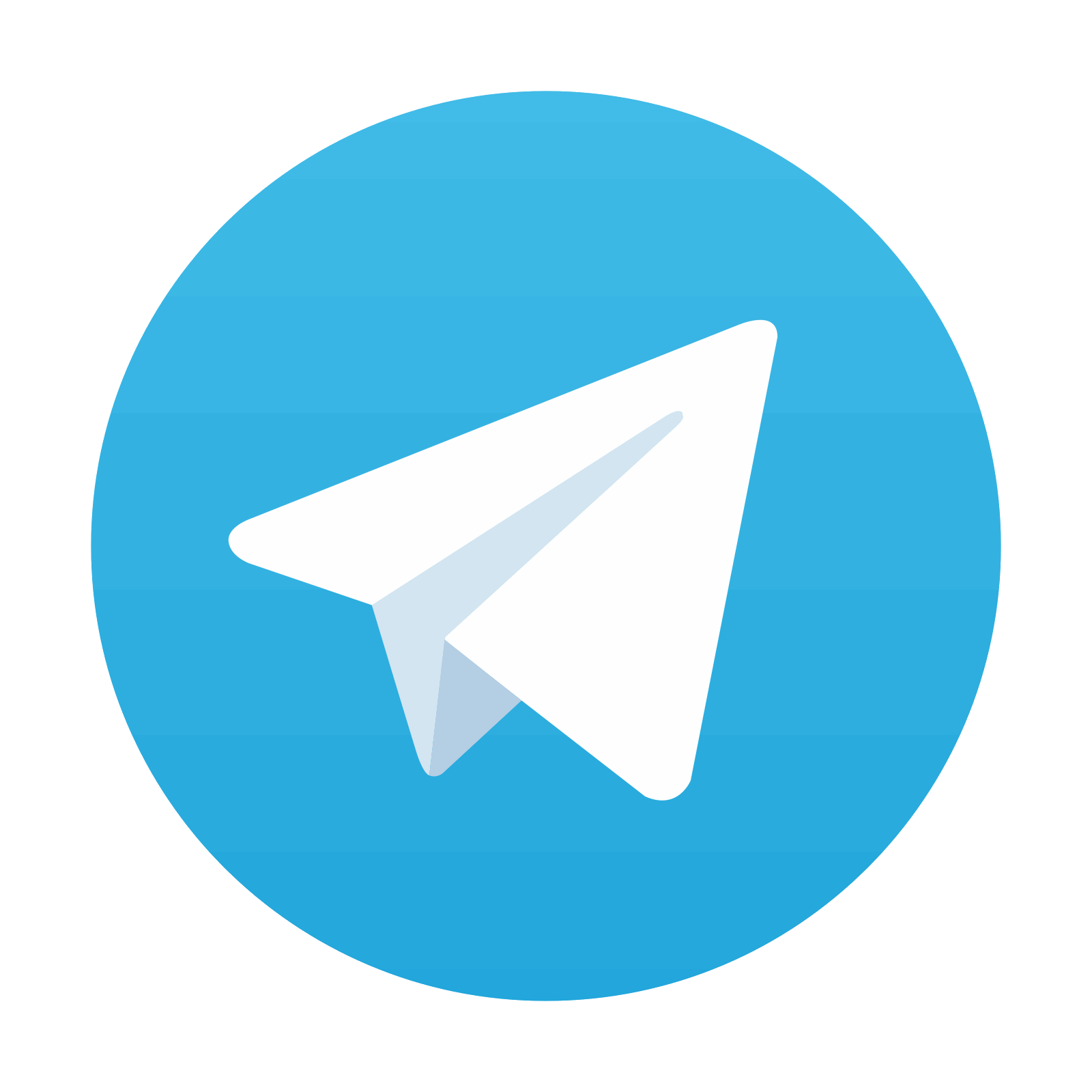
Stay updated, free articles. Join our Telegram channel

Full access? Get Clinical Tree
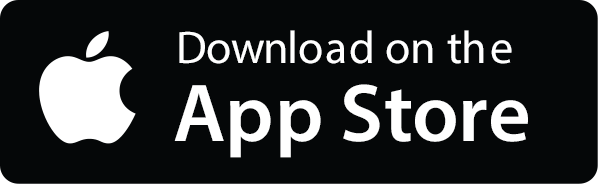
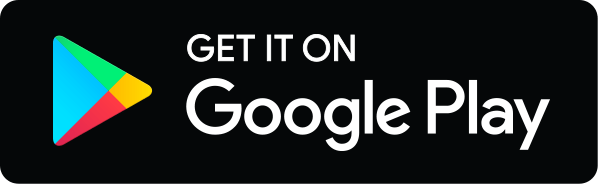
