Table 39-2 Contraindications to Lung Transplantation
Disease-Specific Indications
Disease-specific indications for lung transplantation are listed in Table 39-3.
Chronic Obstructive Pulmonary Disease
Chronic obstructive pulmonary disease (COPD) was the most common indication for lung transplantation prior to the institution of the LAS score, accounting for 35.4% of transplants from 1990 to 2004 and decreasing to 30.9% after 2005.15 Advances in medical therapy for patients with emphysema that can prolong survival include smoking cessation, long-acting bronchodilators, oxygen supplementation, optimization of nutrition, pulmonary rehabilitation, and vaccination for pneumonia and influenza. In addition, lung volume reduction surgery confers a survival benefit in patients with upper lobe–predominant disease, impaired exercise capacity, and the absence of high-risk factors including forced expiratory volume in 1 second (FEV1) less than 20% or carbon monoxide diffusing capacity (DLCO) less than 20%.26 Patients who deteriorate despite maximal therapy and who are not candidates for lung volume reduction should be referred for transplantation.
Factors associated with poor survival in patients with COPD include respiratory exacerbation with hypercapnia, hypoxia, pulmonary hypertension, increasing age, decreasing FEV1, decreasing DLCO, and increased BMI.27 A prospectively validated index based on body mass, airflow obstruction, degree of dyspnea, and exercise tolerance (BODE) has been proposed as a robust predictor of mortality in patients with COPD. A BODE index of greater than 7 on a scale from 0 to 10 was associated with a median survival of 3 years.28
Idiopathic Pulmonary Fibrosis
Usual interstitial pneumonia (UIP) is the pathologic correlate of idiopathic pulmonary fibrosis (IPF) and is the most common subtype of interstitial lung disease. While IPF accounted for 28% of single lung transplants and 14% of bilateral lung transplants from 1995 to 2007, pulmonary fibrosis has become the most common indication for lung transplantation after the initiation of the LAS with 32% of all lung transplants performed for IPF in 2006 and 36.5% in 2013.16,29
Table 39-3 Disease-specific Considerations for Lung Transplantation
Although the options for effective medical therapy for IPF are limited and patient mortality is high with a median survival of 3 to 4 years following diagnosis, patients experience a variable clinical course, with some patients progressing rapidly and others with a more gradual decline. Risk factors for poor survival include a pathologic diagnosis of UIP as opposed to other types of interstitial lung disease, severe fibrosis by high-resolution CT scan, the presence and severity of pulmonary hypertension, and acute pulmonary exacerbations.
Assessment of pulmonary function and exercise capacity can also be used to identify patients at higher risk for mortality. A baseline DLCO of less than 39% and a greater than 10% decrease in DLCO or forced vital capacity (FVC) over a 6- to 12-month period are associated with an increased risk of death.30,31 Oxygen desaturation and a shorter 6-minute walk distance independently predicted increased mortality in patients with pulmonary fibrosis.32
Lung transplant improves survival and quality of life in patients with end-stage interstitial lung disease with a median survival of 4.5 years which may be affected by the increased age at diagnosis.33 Prior to the LAS, IPF patients had the highest waitlist mortality rate34,35 and decreased survival in the early posttransplant period compared to other diagnoses.36 The development of pulmonary hypertension significantly increases the risk of complications and decreases survival.37 LAS scores have increased since 2005 for patients with IPF to 43.3 compared to 38.3 with no change in postoperative mortality while waiting times have decreased from 266 days to 78 days.29
Cystic Fibrosis/Bronchiectasis/Infection-Related End-Stage Lung Disease
Cystic fibrosis (CF) is the third most common indication for lung transplantation, accounting for 16.3% of lung transplants performed in the last 15 years with a median survival of 7.8 years, with most recipients undergoing bilateral transplantation due to the risk of bacterial seeding of the transplanted lung.16 Colonization of the airway with resistant organisms is common in these patients and is not an absolute contraindication to transplantation, although sepsis is an absolute contraindication. Single-institution studies have identified colonization with Burkholderia cepacia genomovar III (redesignated as Burkholderia cenocepacia) to be associated with a 30% to 40% increase in posttransplant mortality, although this finding has not been validated in a multi-institutional setting.
Among patients with pulmonary disease due to CF, the time course of respiratory deterioration is highly variable making the criteria for pulmonary transplantation in this population difficult to define by risk modeling. Deterioration of FEV1 to below 30% of predicted remains an indication for referral for evaluation, although not necessarily for immediate listing.38,39 Other factors to consider include increasing oxygen requirements, hypercapnia, and pulmonary hypertension.40
Pulmonary Artery Hypertension
Idiopathic pulmonary artery hypertension (PAH) has a median survival of less than 3 years if untreated, and the cause of death in most patients is progressive right-sided heart failure. Pulmonary hypertension was a more common indication for transplantation in the past, and after the initiation of the LAS, the percentage of transplant patients waitlisted has decreased from 14.7% in 2002 to 10.8% in 2007 and 6.4% in 2012.23 There has been some concern that the LAS may not adequately prioritize these patients. Increasing use of medical vasodilator therapy including inhaled iloprost and oral sildenafil, prostacyclin analogs, phosphodiesterase 5 inhibitors, endothelin receptor antagonists, and guanylate cyclase stimulators have improved survival in this population and may also be responsible for the decrease in transplants performed for PAH. Patients with a history of right heart failure or poor pretreatment functional status and those with either no response or functional deterioration despite vasodilator therapy should be referred for transplantation. Recent guidelines emphasize progressive deterioration of right heart function despite maximal medical therapy as an indication for referral.40
Cardiac index is an independent predictor for improvement in survival in patients with PAH.7 Patients with left ventricular heart failure requiring inotropic support may not be suitable candidates for pulmonary transplantation alone but instead should be considered for heart–lung transplantation. Patients with PAH made up 23% of heart-lung transplant recipients from 2000 to 2012.4
Lung Allocation and Transplant Benefit
Lung Allocation Score
The goal of the lung allocation system should be to maximize the net benefit for donated lungs. Before 1995, allocation was based solely on wait times, geography, and blood type. In 1995, an exemption was created for an additional 90 days for idiopathic pulmonary fibrosis due to increased mortality for these patients on the waitlist. However, this did not account for differences in mortality for other pulmonary diseases. The allocation system preferentially selected patients with a longer survival, and the waitlist times were greater than 2 years for the majority of listed patients. Due to the increasing number of waitlist deaths, the Final Rule was instituted in 1998 and mandated that medical necessity be considered in organ allocation.35,41
In compliance with the Final Rule, the LAS was developed and has been one of the most significant changes in the practice of lung transplantation over the past 10 years. The LAS was implemented in 2005 and takes into account the urgency (1-year risk of dying on the waitlist without a transplant) and utility (1-year survival posttransplant) giving a net transplant benefit. The normalized LAS ranges from 0 (lowest priority) to 100 (highest priority) for recipients older than 12. The waitlist mortality is given a greater weight in the LAS score. Variables included in the LAS score are listed in Table 39-4.
Lung allocation currently depends on the LAS, blood type, recipient and donor size, geographic location, and age, with donor lungs being offered first to local candidates who are ABO identical and then to patients that are ABO compatible. The donor lungs are then offered to zones A–E, increasingly further away from the donor center. Since the initiation of the LAS, PaCO2 values and bilirubin were added in 2008 while FVC was removed for all groups except group D. The bilirubin has a high impact on potential recipients with idiopathic pulmonary hypertension (group B).
Table 39-4 Selected Components of the Lung Allocation Score
Transplant Benefit
Egan et al.10 analyzed over 4,000 patients on the lung transplant waiting list in order to identify markers of waitlist mortality in the Scientific Registry of Transplant Recipients (SRTR).The listing diagnoses for these patients, including COPD, IPF, CF, and PAH, accounted for 80% of the transplants performed at the time. Transplant candidate demographics, hemodynamic parameters, measures of pulmonary function, and other clinical variables were used to create a regression model. Substantial differences in waitlist mortality were found, with patients listed for emphysematous diseases experiencing a 1-year waitlist mortality of less than 14% compared with 1-year waitlist mortality between 28% and 33% among patients listed with the other three primary diagnoses. These results were confirmed in the International Society of Heart and Lung Transplantation (ISHLT) Registry data from 2005 showing that patients with IPF, PAH, and sarcoidosis had an increased relative risk for 1-year waitlist mortality and a waitlist mortality of 9.7%, 13.1%, 17.8%, and 23.1% for group A, B, C, and D diagnostic groups from 2001 to 2002.15 Before the LAS, patients with COPD were more likely to undergo transplantation since they had a greater chance of surviving to transplant; however, after the initiation of the LAS, incorporating waitlist urgency into lung allocation, IPF has now become the most common indication for lung transplant.
2 The LAS has had a clear impact on waitlist outcomes in lung transplantation. The number of active waitlist patients declined by 54% since early listing no longer conferred an advantage. Waiting time to transplantation has decreased from 792 days in 2004 to 141 days in 2007. The median waitlist time in 2012 was 4 months with 65.3% of recipients transplanted within 1 year.23 Mortality rates on the waiting list have decreased since implementation of the LAS but have started to increase recently to 15.4 per 100 waitlist years from 2010 to 2012, possibly due to an overall increase in the acuity and age of patients on the waiting list.23 The median age of recipients has increased from 45 years in 1985 to 55 years after the institution of the LAS, with 27.2% of recipients older than 65 in 2012.4,22,23 According to a recent report, 20.7% of patients on the waiting list were older than 65 in 2012 compared with 2.5% in 2002 (Fig. 39-1).23
An analysis of all transplant patients with COPD, IPF, and CF from 1992 to 1994 demonstrated a survival benefit for patients with IPF and CF but not with COPD.42,43 A similarly powered analysis of the European transplant experience demonstrated a survival benefit for all lung transplant groups.44 Other studies have also called into question the transplant benefit for children with CF listed between 1992 and 2002.45 Overall early survival (30-day and 1-year) has slowly improved since 1995, which has continued through the implementation of the LAS in 2005 with improvements in operative and postoperative care. A predictive model for posttransplant survival was also developed.10 When these models were applied to evaluate survival in 2,484 patients with these four primary diagnoses, factors including increasing recipient age, intensive care unit (ICU) admission, and the need for mechanical ventilation were significant predictors of 1-year mortality.
Other risk factors for earlier posttransplant mortality included donor/recipient BMI, recipient creatinine, bilirubin, and PA systolic pressure. Malnutrition increases morbidity and mortality after lung transplant. An albumin less than 3 g/dL has been correlated with an increased mortality at 1 year46; 9% to 25% of lung transplant patients are malnourished. Allen et al.47 found decreased survival in patients who were either under- or overweight.
Results of studies evaluating posttransplant survival after initiation of the LAS are conflicting. Since the initiation of the LAS, sicker patients are being transplanted (Fig. 39-1), and an increasing LAS has been associated with a lower 1-year survival. Transplanted patients with an LAS score less than 35 had a 1-year survival of 85.7%, whereas those with an LAS score greater than 60 had a 1-year survival of 71.3%.48 The median LAS continues to increase to 40.8 in 2011 with 6.3% of patients with LAS scores greater than 50 compared with a median LAS of 36.6 in 2005 with less than 2% greater than 50.49 A study in 2008 showed an increased incidence of primary graft dysfunction and increased ICU stays after initiation of the LAS although the 1-year survival was similar.50 McCue et al.51 reported no difference in posttransplant morbidity with a small statistically significant improvement in 1-year survival. One-year survival improved from 70% in 1988 to 1994 to 87% from 2007 to 2012.22,23 Thabut et al.52 evaluated the survival benefit of transplant patients with CF after initiation of the LAS and found that lung transplant decreased the risk of death by 69%, and a higher LAS was associated with an increased survival benefit.
Reevaluation and revision of the criteria that determine the LAS remains one of the objectives of the Thoracic Organ Transplantation Committee of the OPTN. Data will continue to be analyzed through large studies including REVEAL (Registry to Evaluate Early and Long-term Pulmonary Arterial Hypertension Disease Management) and the Lung Retrospective Data Collection Projects, and the LAS will continue to evolve to maximize the net lung transplant benefit.53,54 The first comprehensive revision of the LAS was approved in November 2012 by the OPTN Board of Directors but has yet to be implemented. The revision includes changes to the variables included in the LAS such as cardiac index, CVP, and serum creatinine and the weight given to each variable in the LAS calculation. These changes will have the greatest effect on patients in diagnosis group B.53
Figure 39-1. Since the initiation of the Lung Allocation Score (LAS) in 2005, the percentage of waitlist patients older than 65 has markedly increased. Patients are also sicker with an increasing percentage of patients with an LAS score >50. Meanwhile, COPD (group A), with a lower waitlist mortality, has decreased as an indication for lung transplant while idiopathic pulmonary fibrosis (group D) has grown to become the most common indication. (Adapted from the 2012 OPTN/SRTR Annual Report.)
Critically Ill Patients
Before the institution of the LAS, critically ill patients were at a disadvantage because the primary criterion was time on the waiting list. Under the LAS, the risk of waitlist mortality carries a greater weight than posttransplant survival.10 A study by Russo et al.49 evaluated associations between the LAS and postoperative morbidity and mortality. They found that an LAS of greater than 75 was correlated with a decreased 1-year survival and increased perioperative complications. There was no difference in mortality at 1 and 3 years. With the LAS, there has been some concern that the increased impact of predicted waitlist mortality will result in allocation of donor lungs to patients with a lower posttransplant survival. Russo et al.49found that patients with an LAS score between 90 and 100 had a survival rate of 1.56 years while those between 80 and 90 LAS had a survival of 2.28 years. A higher LAS score was also associated with primary graft dysfunction (PGD) and decreased graft survival.22 The rate at which the LAS changed over a patient’s course affected survival with changes greater than five associated with a worse survival.55
Extracorporeal Membrane Oxygenation
The use of ECMO can be lifesaving in cases of severe PGD posttransplant. Some centers prefer venoarterial ECMO in order to reduce blood flow to the donor lung thus limiting the degree of pulmonary edema56 while others prefer venovenous ECMO due to the lower complication rate. ECMO is now available in more than 200 centers.57,58 Using ECMO as a bridge to lung transplant has been associated with poor outcomes in the past.59–61 However, outcomes have improved with developments in oxygenator technology using polymethylpentene membranes to reduce plasma leakage, dual lumen cannulas and smaller pumps and oxygenators allowing ambulatory ECMO, centrifugal pumps, and improvements in critical care.
In venovenous ECMO blood is drawn from the central venous system, passed through the pump and oxygenator, and returned to the right atrium. Newer dual lumen cannulas allow this to be performed through a single cannulation site, often using the jugular vein. Patients with high pulmonary arterial pressures and right heart failure may require conversion to venoarterial ECMO or an atrial septostomy. In venoarterial ECMO, blood is withdrawn from a central vein and returned to a peripheral or central artery providing hemodynamic and respiratory support. The configuration and cannulation depend on the patient’s needs and mobility. Venovenous ECMO is a good choice in patients with hypercapnic or hypoxemic respiratory failure without significant right heart dysfunction, such as most patients with CF. A single dual lumen cannula with insertion in the jugular vein allows patients to ambulate. Physical therapy should be consulted as soon as possible. Ambulating patients require close coordination with the ECMO specialist, respiratory therapist, nursing, and the critical care team. Some patients can be extubated while on ECMO, with the goal to keep patients comfortable so they are able to participate in physical therapy. It is also important to avoid blood transfusions when possible to avoid development of antibodies that can limit donor organ availability, only transfusing patients when the hemoglobin is <6 g/dL, if there is hemodynamic instability, or if patients are hypoxemic despite ECMO support. For CO2 clearance alone, a pumpless system driven by the patient’s own arterial pressure can be used with groin cannulation, decreasing ventilator-induced trauma.62,63
Venovenous ECMO may be insufficient for patients with significant right heart dysfunction and pulmonary hypertension. Venoarterial ECMO or pulmonary artery to left atrial cannulation may be more beneficial by unloading the right ventricle. Central cannulation is avoided when possible to prevent complicating the subsequent lung transplant. Femoral arterial cannulation makes ambulation more difficult and does not oxygenate the carotids and coronary arteries as well. Axillary arterial cannulation using a 6- to 8-mm vascular graft provides more effective upper body oxygenation.
3 Since the initiation of the LAS, patients on mechanical ventilation have higher scores and are more likely to undergo a transplant. These patients are also more likely to be deconditioned and have decreased posttransplant survival. In a study from 2010, patients undergoing transplant on mechanical ventilation had a significantly lower survival of 57% versus 70% at 1 year, although survival is similar after 6 months.64 Ambulatory ECMO can be used to help prevent deconditioning of these patients prior to transplant.
The decision-making process must include a multidisciplinary team including the ECMO and critical care intensivist, transplant surgeon, pulmonologist, and social work. The goals of care include establishing adequate CO2 removal, oxygenation, and circulatory support. Whether ECMO is being used as a bridge to recovery or to lung transplant should be established when possible before initiating ECMO. Factors to consider include the patient’s age, the underlying pulmonary disease, social support, other organ dysfunction, the presence of infection, and variables affecting the potential waiting times including the patient’s size and panel of reactive antibodies (PRA). Patients who have completed their transplant evaluation or are in the process of being evaluated, younger patients, and those who were listed prior to an acute exacerbation generally have better outcomes. Timing is important and the initiation of ECMO should not be delayed to the point where the patient is no longer a transplant candidate due to deconditioning. Their pulmonary disease should have progressed to the point where it is unlikely that they will improve, but patients must have sufficient functional reserve. Active bacteremia or highly resistant infections are contraindications. Patients with renal, hepatic, and cardiac failure are also not candidates for ECMO. It is important to carefully discuss the decision to proceed to ECMO with the patient and family, as well as considering the possibility of withdrawal from ECMO in the event that the patient is no longer a transplant candidate due to complications including sepsis, renal, liver, or left heart failure, stroke, or worsening functional status despite circulatory support. Daily clinical assessment is important to ensure that the patient continues to meet transplant criteria.
Due to the severity of pulmonary disease, patients with an LAS that is greater than 90 have a postoperative mortality that is higher than expected. In a study from 2005, ECMO was used as a bridge to transplant in 31 patients with 25 surviving to transplantation.65 The 2-year survival rate was 74% in both the unsupported and the bridge to transplant groups. The rate of PGD was higher in the ECMO group.65 In a study from 2013, 31 patients were successfully bridged to lung transplant, including 19 who were ambulatory, with an 80% 3-year survival.66 In another study, 30 of 36 patients on ECMO were bridged to lung transplant with a 2-year survival rate of 60.5%. Survival was greater among patients with CF at 71% compared to idiopathic pulmonary fibrosis at 27.3%.67 The duration of ECMO also affects morbidity and mortality. One study showed a 76% 1-year survival with patients on ECMO less than 14 days having a significantly improved survival.68 Fuehner et al. evaluated 26 patients bridged to lung transplant compared to 34 historical control patients on mechanical ventilation. Survival at 6 months was higher in the ECMO group at 80% versus 50%.69 While transplant outcomes for patients undergoing ECMO as a bridge to transplant have been acceptable, larger cohort studies are needed to optimize selection criteria for patients bridged to transplant.
DONOR CONSIDERATIONS
Donor Selection
While the number of lung transplants has steadily increased, with 1,830 transplants in 2011, and waitlist times and waitlist death rates have decreased, the total number of patients on the waitlist has continued to increase to 2,200 highlighting the shortage of acceptable donors. The reasons that fewer lungs are available compared to other organs are multiple. Death from head injury or intracranial bleeding may lead to neurogenic pulmonary edema, while chest trauma can result in contusions or pneumothorax. All brain-dead donors are intubated and at risk for aspiration and nosocomial pneumonia, especially if there is a prolonged interval between hospitalization and declaration of brain death. In addition, hemodynamic instability, whether from herniation or trauma, often results in significant volume resuscitation that can contribute to acute lung injury. Criteria for an ideal lung donor include age <55 years, less than 20 pack-year smoking history, < 48 hours on the ventilator, a PaO2:FiO2 ratio >300, and no evidence of edema or infection (Table 39-5).70,71
Bronchoscopy allows for direct examination of the bronchial tree and for microbiologic examination of the bronchoalveolar lavage (BAL), the results of which may influence later treatment of the recipient. Chest radiography and computed tomography are useful to evaluate for effusions, pulmonary infiltrates, consolidation, and contusions that could contribute to donor hypoxemia. Unlike other solid organ transplants, lungs are unique in carrying a relatively high risk of infection (i.e., pneumonia) following transplant. Because all brain-dead patients have endotracheal tubes and are on mechanical ventilation, there is a high likelihood that the airway is either colonized with bacteria or that there is ongoing pulmonary infection.
Not infrequently, the donor lungs may not be suitable because of infiltrates when all other organs are acceptable. Significant infiltrates typically preclude the use of donor lungs. However, a bilateral lung transplant may still be considered, in a recipient with a higher LAS, when there is a small infiltrate in one lung without evidence of purulent secretions and the contralateral lung appears normal. In addition, unilateral pulmonary infiltrates do not necessarily preclude use of the normal contralateral lung for a single lung transplant.72
Aspiration at the time of the initial insult resulting in brain death is a common cause of pulmonary infiltrates in potential donors. Signs of aspiration may not be evident on a chest radiograph for 24 to 48 hours, underscoring the importance of the bronchoscopic examination. Characteristic early bronchoscopic evidence of aspiration includes erythematous tracheobronchial mucosa, purulent secretions, and occasionally the presence of food particles.
Table 39-5 Criteria for Ideal Lung Donors
Major pulmonary contusion resulting from blunt chest trauma may also eliminate lungs from donor consideration, but minor to moderate unilateral contusions are often acceptable. Evaluating the full extent of the contusions at the time of donor procurement can be difficult because the interval from injury to determination of brain death and donation may be short. Although the detrimental effect on gas exchange caused by a pulmonary contusion is usually transient, further bleeding into the lung parenchyma could occur if cardiopulmonary bypass is required to perform the transplant. Pulmonary edema may occur as a result of massive head injury and may be exacerbated by donor management protocols directed at maintaining satisfactory perfusion and function of nonpulmonary organs.
The limitation in overall donor organ availability is further compounded by the relatively smaller number of usable donor lungs. The standard criteria for an acceptable donor lung are particularly stringent (Table 39-5).73 There are limited data available to validate these criteria, and multiple single-institution retrospective reports evaluating “extended criteria” donors have shown conflicting short- and long-term outcomes for almost every criterion. Clinically, these selection criteria provide an overall indication of the quality of the donor lungs. When multiple criteria are not met, the risks of transplanting such lungs likely outweigh the benefits. However, if one aspect of the donor lungs is marginal, the lungs may still be acceptable, and the risks must be weighed against recipient acuity and the likelihood of receiving another offer, taking the patient’s size and antigenic status into account.
There are several caveats that have come into “standard” practice. Although leukocytes or occasional bacteria on sputum Gram stain can be acceptable, the presence of gross pus or fungal elements confers a high risk of perioperative complications.74 In the annual report of lung transplant outcomes, increasing donor age modestly increased the risk of 5-year mortality.16 Analysis of a large cohort of more than 750 lung and heart–lung recipients demonstrated significantly worse long-term survival with ischemic times greater than 330 minutes. Hazard ratios for death were threefold higher in patients with ischemic times of 8 hours and nearly eightfold higher when ischemia reached 10 hours.75 The detrimental effects of older donor age and longer ischemic times appear to be additive.76
Donor Management
4 Despite the inability to identify “extended” donor criteria that reliably provide acceptable grafts, donor management optimization has allowed an increase in donor recovery and is likely responsible for the increase in the overall number of transplants performed over the last decade.The number of donor lungs recovered has increased from 0.25 lungs per donor in 2000 to 0.39 lungs per donor in 2012.23 Algorithm 39-1 outlines the donor management algorithm used at the University of Michigan. Key principles include the early use of steroids and thyroxine to prevent neurogenic pulmonary edema and to maintain cardiovascular stability, aggressive alveolar recruitment with positive end-expiratory pressure (PEEP) for patients with a partial pressure of arterial oxygen (PaO2) less than 350 mm Hg, optimization of intravascular volume, maneuvers to prevent aspiration, and lung-protective ventilation utilizing high frequency, low tidal volumes, and PEEP to prevent volutrauma and barotrauma to the lungs. Using these maneuvers has been shown to double the organ recovery rate without detrimental effects on 30-day or 1-year survival.77
Investigators at the Texas Organ Sharing Alliance identified 330 potential lung donors over a 4-year period preceding initiation of an active donor management protocol and 381 potential donors managed on the protocol in the subsequent 4 years.77 Overall, 1.7 organs per donor were procured in 19% (136/711) of potential donors. Prior to initiation of the management protocol, organ procurement occurred in only 12% (38/330), recovering 1.6 lungs per donor. In contrast, with lung-protective donor management, organ procurement occurred in 26% (98/381), recovering 1.7 organs per donor.
Algorithm 39-1. The donor management algorithm used at the University of Michigan in coordination with the organ procurement organization, Gift of Life. Optimal PEEP is determined by increasing PEEP 2 cm of H2O every 3 to 5 minutes until compliance decreases. Recruitment maneuvers include CPAP at 30 cm of H2O for 30 seconds every 20 minutes × 3. P/F, PaO2/FiO2; IBW, ideal body weight; TV, tidal volume; APRV, airway pressure release ventilation; CPAP, continuous positive airway pressure.
Following initial organ acceptance, the lungs might be declined if the procurement team identifies significant purulent secretions during bronchoscopy, if arterial blood gases deteriorate significantly to a P/F ratio <300 or if the donor lungs are found to have poor compliance. On-site measurement of selective pulmonary vein blood gases at the time of donor procurement can aid the procuring team in determining whether donor hypoxemia is due to unilateral or bilateral lung compromise.78 Size of the donor lungs is less important when the recipient suffers from emphysema, in which each hemithorax is large, compared to pulmonary fibrosis, in which the hemithorax can be significantly contracted. The most important size consideration is a reasonable match between donor and recipient height.
Lung Preservation
Unlike the kidney, liver, or pancreas, immediate acceptable function of the transplanted lung is vital for survival of the recipient. PGD occurs in 10% to 25% of cases and is the most common cause of 30-day mortality after lung transplantation. PGD is associated with higher rates of chronic rejection (bronchiolitis obliterans syndrome [BOS]) and correlates with increased 1- and 5-year mortality.79
5 Ischemia-reperfusion injury refers to the cellular and architectural changes that occur after cross-clamp of the donor aorta to release of the recipient pulmonary arterial clamp (ischemic phase) through the immediate restoration of systemic gas exchange (reperfusion phase). Minimizing ischemia-reperfusion injury is crucial to ensuring good early graft function. Advances in donor lung preservation techniques have led to more consistent quality of the donor allograft and lower rates of PGD.
Current preservation techniques depend on cold static preservation to decrease metabolic activity using topical cooling and cold pulmonary perfusion. A major development in lung preservation has been the widespread clinical use of a preservation solution specifically designed for lung procurement. Traditional preservation solutions such as Euro-Collins solution were designed to maintain intracellular ion balance and cell wall integrity. Low-potassium dextran (Perfadex, Vitrolife, Goteborg, Sweden) has an extracellular fluid ion balance and has been shown to have beneficial effects on endothelial cell function and pulmonary microcirculation. Five single-institution reports have demonstrated significantly better initial lung function with low-potassium dextran, while one study showed no difference.80
The donor allograft is cooled to minimize cellular metabolism while maintaining vital cellular function. Although small animal studies have demonstrated better postischemic function at preservation temperatures between 15°C and 23°C, practical limits have led to the common practice of cooling to 4°C to 8°C (the temperature of ice water). Other techniques that have traditionally been employed to ensure rapid homogeneous cooling of the lung include topical cooling, antegrade flush through the pulmonary artery, and techniques to maintain patency of the microcirculation including the administration of prostaglandins prior to cross-clamp and the maintenance of ventilation after cross-clamp. Retrograde flush through the pulmonary veins purges the pulmonary and bronchial circulations, has a more homogeneous distribution, and can evacuate pulmonary arterial clots or fat emboli. Clinically, retrograde perfusion in combination with the antegrade flush has been shown to improve immediate posttransplant oxygenation.81
Optimal storage techniques have also evolved. After flushing of the pulmonary circulation, the lungs are recruited to expand atelectatic areas, and the trachea is stapled and divided prior to separation from the donor and storage for transport. Methods of lung recruitment have been studied in animal models and have shown that ventilation with less than 50% oxygen and maintenance of airway pressure between 15 and 20 cm H2O have beneficial effects on capillary leak, lipid peroxidation, and barotrauma.82–84
Lung injury likely results not only from the ischemic insult but also from reperfusion of the ischemic lung. Several experimental models of acute lung injury implicate oxygen free radicals as a factor in reperfusion injury. A significant early increase in lung permeability is seen after an ischemic period followed by reperfusion, which improves within several hours. After single lung transplantation, changes in the contralateral, nonischemic lung are presumably the result of substances released during reperfusion of the ischemic lung. Animal models have demonstrated that gradual pressure-controlled reintroduction of blood flow and a protective ventilation strategy of high frequency and low tidal volume reduce lung injury.85,86
Expanding the Donor Pool
Ex Vivo Lung Perfusion
Currently only 20% of brain death donor lungs are used for transplantation due to damage from trauma or complications related to brain death and critical care including barotrauma and edema.87,88 The number of patients on the waiting list exceeds the number of donor lungs available. With current cold static preservation techniques, it is difficult to assess lung function after organ procurement. Normothermic ex vivo lung perfusion (EVLP) (Fig. 39-2) allows for objective evaluation of marginal lungs in an optimized environment leading to improved utilization of available donor lungs.
Early attempts at EVLP failed due to the development of pulmonary edema.89 Steen et al.90 initially evaluated EVLP as a means to evaluate donor lungs from uncontrolled donation after cardiac death (DCD) circumstances. They developed an optimized colloid lung perfusate solution that keeps fluid within the intravascular space and also provides nutrition during lung perfusion (Steen Solution, XVIVO, Sweden).91 The perfusion period was initially short, at around 60 minutes, and the solution was mixed with red blood cells. The Toronto group first described stable long-term perfusion for 12 hours using an acellular Steen solution, protective perfusion with only 40% of the cardiac output, and physiologic left atrial positive pressure.92
Lung injury is reflected in the development of pulmonary edema during EVLP. Parameters used to evaluate the donor lungs include the perfusate pO2, airway pressure, lung compliance, and pulmonary vascular resistance. While PaO2 is one of the most important in vivo donor parameters, pO2 is less useful ex vivo. Dynamic compliance is the most sensitive parameter for the development of pulmonary edema ex vivo.93 Parameters are monitored over a 3- to 4-hour period, and it is important to take the trends into account in addition to the absolute values. A delta pO2 (pulmonary vein pO2 – pulmonary artery pO2) of greater than 300 mm Hg on 100% is used as criteria for lung acceptance for transplantation. Chest x-rays, bronchoscopies, and deflation tests are also performed.
Figure 39-2. The donor lungs have been placed on ex vivo lung perfusion with pulmonary arterial and left atrial cannulation and the change in pO2, compliance, and pulmonary vascular resistance will be evaluated to determine if the lungs are acceptable for transplantation.
Several prospective trials have recently been completed or are underway including the NOVEL trial (United States; XVIVO), the HELP trial (Toronto; XVIVO), the INSPIRE and EXPAND trials (Europe and the United States; Transmedics), the Perfusix trial (United States; Perfusix), the Vienna trial (Vienna; XVIVO), and the DEVELOP trial (United Kingdom; Vivoline). Different technologies and techniques are used in the various trials. While the NOVEL, HELP, DEVELOP, EXPAND, and Perfusix trials are evaluating extended criteria lungs, the INSPIRE and Vienna trials are evaluating standard criteria lungs. In the HELP trial, 80% of the lungs from brain dead and DCD donors that were not initially acceptable for transplantation were transplanted after EVLP with outcomes equivalent to standard controls.94,95 The incidence of PGD grade 3, airway complications, and the need for ECMO has been <5%.
EVLP also provides the potential for rehabilitating injured lungs. Specific therapies could be used depending on the type of lung injury. Terbutaline increases clearance of alveolar fluid during EVLP96 while applying surfactant to porcine lungs injured by aspiration improved graft function.97 Treatment of donor lungs with high-dose antibiotics could also be useful with the large number of lungs rejected for pneumonia.98,99 For donors with acute pulmonary emboli, tissue plasminogen activator has been used to lyse emboli with good outcomes after transplant.100 Gene therapy with IL-10 is also being studied using adenoviral vectors in rejected donor lungs and has shown improved function.101 In addition, the application of stem cells has been reported to restore endothelial barrier permeability after lung injury.102
Donation after Cardiac Death
DCD may be valuable in increasing the donor pool with only 20% of donor lungs acceptable for transplant. The lung is unique compared to other organs since it does not depend solely on perfusion for oxygen, and cell viability can be maintained by mechanical ventilation after cardiac arrest. Cadaveric lungs have been shown experimentally to remain viable for 1 hour to 90 minutes after arrest with function declining after 2 hours although this does not account for the agonal phase, which can vary in duration and the degree of hypoxia and hypotension.103–105 On the other hand, DCD donor lungs may actually have better function, since they are not exposed to the catecholamine surge and neurogenic pulmonary edema associated with brain death and may be less sensitive to ischemia-reperfusion injury.106 The Toronto group reported that lungs from brain-dead donors had higher levels of proinflammatory cytokines than DCD lungs.107
Currently only 2% of DCD lungs are utilized in the United States.108 However 13.3% of lung transplants in the United Kingdom and 22.5% in Canada are from DCD donors.109 DCD donors are classified using the Maastricht classification into four groups: I, dead on arrival; II, failed resuscitation; III, awaiting cardiac arrest; IV, cardiac arrest in a brain-dead donor.110 Controlled DCD (category III and IV) is the approach of choice and includes withdrawal of life support in the ICU or operating room so the organs can be allocated in advance with the ability to evaluate graft function and discuss donation with the family. DCD donors generally have an irreversible cerebral injury, high spinal cord injury, or end-stage muscular disorder and are expected to die within 1 hour of the withdrawal of support. Predictive models have been developed to predict the potential for expiration based on the need for pressors, cardiopulmonary status, age, BMI, Glasgow Coma Scale, peak and inspiratory pressures, and the PaO2/FiO2 ratio.111–113
Death of the donor, with irreversible cessation of respiration and circulation, must be declared before organ retrieval can begin, and the retrieval process must not alter or hasten death. One of the main differences from brain death donation is the addition of a warm ischemia period. The length of the agonal phase, from the withdrawal of care until the declaration of death which includes a 2- to 5-minute no touch period after circulatory arrest, varies among donors and is associated with impaired graft function and longer hospital stays.114,115 Most DCD protocols limit the agonal phase to 60 minutes for lung donation. Heparin is generally given before cardiac arrest although some donor hospitals restrict its use since heparin can hasten death in donors with intracranial hemorrhage. Heparin given postarrest can prevent pulmonary thromboemboli, and retrograde perfusion is helpful in clearing any emboli116,117 with no difference in thrombus formation.118–121 After arrest, the donor lungs can be rapidly cooled topically, through chest tubes or after exposure of the lungs, followed by antegrade perfusion with cold Perfadex and retrograde perfusion through the pulmonary veins.
EVLP may increase the use of DCD lungs by allowing evaluation after cardiac death in optimized conditions, which is especially useful in DCD cases since in vivo evaluation is more limited and the warm ischemia time is variable. There is also the potential to improve lung function. The Toronto group transplanted lungs using 20 donor lungs placed on EVLP, including 9 DCD lungs, with no significant difference in 30-day mortality, ICU or hospital length of stay. Although the use of EVLP remains controversial since DCD lung transplants can also be performed with good outcomes without EVLP.108,109
The first successful lung transplant from a DCD donor was reported in 1995.122 Early and midterm outcomes after transplantation of lungs from controlled DCD donors are comparable to standard lung transplantation.123–125 Although the incidence of PGD grade 3 was not significantly different, in some series there was a trend toward increased early graft dysfunction with improvement within 72 hours and more frequent ECMO usage.125,126 The incidence of BOS is similar but was more frequent in the brain death group at 1 year in a series from the Netherlands.127 In a series of uncontrolled DCDs from Madrid, the incidence of BOS at 5 years was 45%, which was significantly higher, although outcomes were improved after using EVLP to evaluate donor lung function.128
TRANSPLANTATION OPERATION
Single Versus Bilateral Lung Transplant
Over the past decade the ratio of bilateral lung transplants has continued to increase from 49.9% of all lung transplants in 2002 to 67.3% in 2012.23 Bilateral lung transplant is required for septic lung diseases, including CF, due to the risk of contaminating the transplanted lung. Whether to perform a single or bilateral lung transplant for other diseases remains controversial, and short- and long-term outcomes as well as benefits to society should be considered.129,130 While double lung transplants may provide greater benefits to the recipient, single lung transplants may maximize the benefit to society by allowing two patients to be transplanted.
The decision to proceed with single or bilateral lung transplantation depends on several factors including the primary diagnosis, the presence of pulmonary hypertension, recipient age, and donor lung availability. Patients with chronic infection, such as those with CF or immunoglobulin deficiency disorders, require bilateral transplant. Single lung transplantation can be considered for patients with restrictive physiology (pulmonary fibrosis), particularly if there is no evidence of secondary pulmonary hypertension. The decision on which side to transplant is based on both donor lung availability and the recipient’s quantitative ventilation perfusion scan.
In patients with end-stage obstructive lung disease, specifically emphysema, there was concern initially that single lung transplantation would be associated with altered physiology due to hyperventilation of the overly compliant native lung and that mediastinal shift would result in significant ventilation and perfusion mismatch and compromised function of the transplanted lung. It has been demonstrated that single lung transplantation is acceptable in patients with emphysema,131 especially for older patients, with significant improvements in mean 6-minute walk results (Fig. 39-3). However, bilateral lung transplant recipients appear to have greater improvement in FEV1 at 3-month (Fig. 39-4) and 1-year follow-up.132
A single lung transplant is simpler technically and avoids a sternotomy. While some studies have found improved early 30-day and 3-month survival with single lung transplant,133,134 others have found no difference in survival135,136 or other postoperative outcomes including mechanical ventilation and ICU stay.137,138 Bilateral lung transplantation, when indicated, has been simplified by the development and refinement of the sequential rather than the en bloc double lung technique, which required a tracheal anastomosis with cardiopulmonary bypass and resulted in significant perioperative morbidity and mortality.139 Bilateral sequential lung transplant can be approached either by median sternotomy or bilateral thoracotomies using cardiopulmonary bypass when necessary depending on the degree of pulmonary hypertension and impairment in gas exchange. A bilateral thoracosternotomy (“clamshell”) incision permits easier exposure of the hilum especially on the left but tends to be more painful.
Figure 39-3. Mean 6-minute walk results for patients undergoing single lung transplantation for emphysema. There is significant improvement at 6 weeks with continued improvement after 12 weeks.
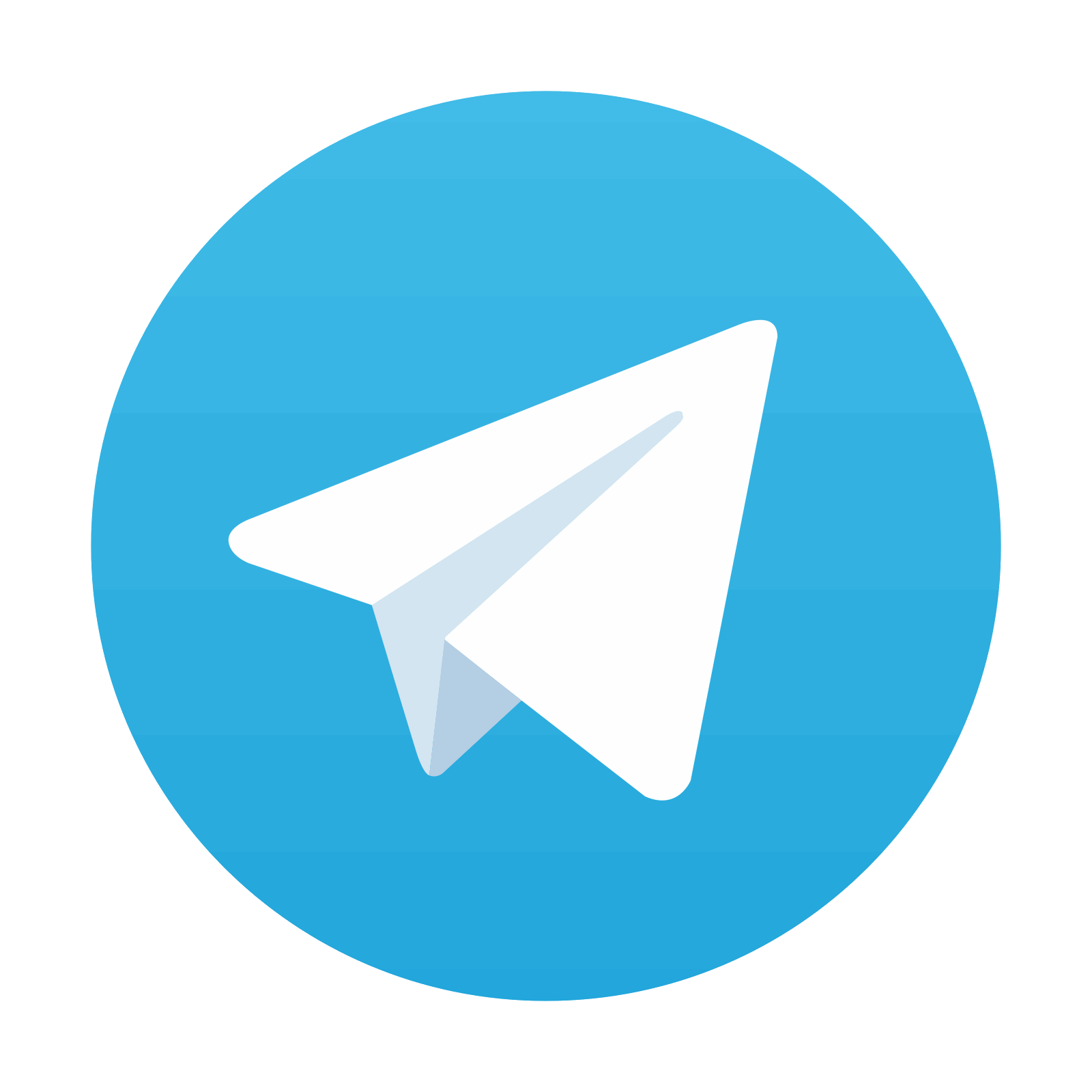
Stay updated, free articles. Join our Telegram channel

Full access? Get Clinical Tree
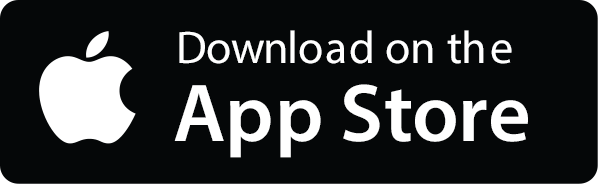
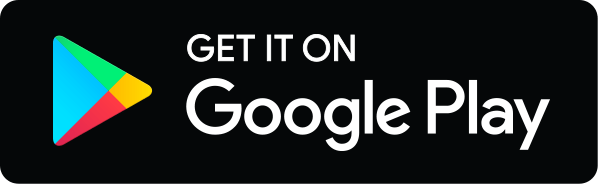