Protein-Energy Malnutrition1
Manuel Ramirez-zea
Benjamin Caballero
1Abbreviations: ART, antiretroviral therapy; DALYs, disability-adjusted life years; HA, height for age; HIV, human immunodeficiency virus; IUGR, intrauterine growth restriction; MUAC, midupper arm circumference; NG, nasogastric; ORS, oral rehydration solution; OTP, outpatient therapeutic program; PEM, protein-energy malnutrition; PUFA, polyunsaturated fatty acid; ReSoMal, rehydration solution for malnutrition; RUSF, ready-to-use supplementary food; RUTF, ready-to-use therapeutic food; SC, stabilization center; SFP, supplementary feeding program; SPEM, severe protein-energy malnutrition; UNICEF, United Nations Children’s Fund; WA, weight for age; WH, weight for height; WHO, World Health Organization.
The term malnutrition technically includes both undernutrition and overnutrition (obesity), but it continues to be used by most organizations to define nutrient deficiencies or inadequate body weight for age or relative to height. This chapter uses the term protein-energy malnutrition (PEM) to describe the condition in which the most salient elements are a depletion of body energy stores and of tissue proteins, observed over a range of combinations and severity, and usually accompanied by micronutrient deficiencies. PEM can be the direct result of inadequate food intake (primary PEM), or caused by recurrent illnesses associated with gastrointestinal malabsorption, reduced appetite, and/or increased nutrient needs (secondary PEM). This chapter discusses primary PEM.
HISTORICAL BACKGROUND
Although undernutrition has existed since ancient times, the condition was not clinically described until the seventeenth century, when Soranio coined the term marasmus to describe emaciated children (1). In 1865, Hinojosa in Mexico described a syndrome associated with edema, skin and mucosal lesions, hair discoloration, and apathy (2). The syndrome was attributed to multiple vitamin deficiencies (3) until 1932, when Cicely Williams, working in West Africa, correctly linked it with deficient protein intake and named it kwashiorkor, or disease of the weaned child (4). Numerous studies described the same syndrome under a variety of names: Seller (1906) in Germany as “Mehinahrschaden,” Patron-Correa (1908) in Mexico as “culebrilla,” Marfan (1910) in France as “dystrophoie desfarineux,” Frontali (1927) in Italy as “distrofia de farine,” Lieurade (1932) in Cameroon as “les enfants rouges,” Williams (1932) in England as kwashiorkor, Oropeza y Castillo (1937) in Venezuela as “síndrome de carencia: avitaminosis,” Trowell (1937) in Uganda as “infantile pellagra,” and Scroggie (1941) in Chile as “síndrome pluricarencial de la infancia”; it has rightly been called the disease of the 100 names (5, 6).
Between 1949 and 1953, the Food and Agriculture Organization and the World Health Organization (WHO) commissioned several teams to study the disease in Africa (John Brock and Marcel Autret), Central America and Mexico (Moises Behar and Marcel Autret), and Brazil (John Waterlow and Arturo Vergara). This initiative was the beginning of intense research activity during the following 20 years that resulted in consistent definition of the syndrome and of treatment approaches (6). Key discoveries included the association of kwashiorkor with low concentration of serum proteins, with low protein quality, and with extensive and cyclic interactions between undernutrition and infection (7, 8).
In the last third of the twentieth century to the present time, severe cases of undernutrition were seen mostly in refugee and emergency camps. World attention switched to moderate forms of undernutrition (moderate acute
undernutrition, moderate or severe stunting) as well as to their long-term consequences (e.g., Barker hypothesis).
undernutrition, moderate or severe stunting) as well as to their long-term consequences (e.g., Barker hypothesis).
EPIDEMIOLOGY
The most vulnerable period during the life course for stunting and acute undernutrition is early childhood, as a result of the high nutritional requirements relative to body size. Frequent acute infections aggravate the problem by further increasing nutrient demands or gastrointestinal losses. The prevalence of severe wasting is usually highest in the first 2 years of life, and it declines thereafter. The prevalence of stunting has been shown to increase progressively until reaching a plateau around 24 months (Fig. 68.1) (9).
In 2005, about 36 million (6.5%) children less than 5 years old who were living in developing countries had moderate wasting, and another 19 million (3.5%) had severe wasting or severe protein-energy malnutrition (SPEM). Approximately 69% of severely wasted children lived in Asia, 29% in Africa, and 2% in Latin America. This is part of the reason that 99% of deaths in children younger than 5 years old occur in those continents (10). This prevalence varies substantially within countries and is highest for the poorest segments of the population.
In 2010, there were 171 million (26.7%) stunted children worldwide, of whom 97.5% lived in developing countries (11). Although this represented a relative decrease of 33% since 1990, when the percentage was 39.7%, stunting remains a public health problem in many developing countries. Approximately 90% of stunted children live in just 36 countries (21 in Africa, 13 in Asia, and 2 in Latin America) (10). Of all stunted children, 58% live in Asia (half in India), 35% in Africa, and 7% in Latin America. The relative decrease between 1990 and 2010 has been remarkable in Asia (43%, from 48.6% to 27.6%) and Latin America (43%, from 23.7% to 13.5%), but in Africa the decrease was only 5% (from 40.3% to 38.2%). If these trends continue as anticipated, there will be the same number of stunted children in Asia and Africa by 2020 (11).
Undernutrition often starts during pregnancy as a result of dietary deficiencies and concurrent increases in nutrition requirements of the pregnant woman. Low birth weight infants secondary to intrauterine growth restriction (IUGR; at term babies who weighed <2500 g) represent around 11% of all live births each year in developing countries—12.8 million in 2004 (10). The prevalence of acute undernutrition in older children (>5 years of age) is lower than in younger children, and the condition tends to be less severe. Stunting can be highly prevalent in children over 5 years of age, but because it is usually an irreversible condition related to undernutrition in the first two years of life.
Acute primary PEM in adolescents and adults is rare and is usually associated with a primary illness that compromises food intake or increases intestinal losses. Acute PEM may result from chronic deprivation caused by medical conditions or by protracted food scarcity.
In developed countries, primary undernutrition is an uncommon condition, seen mainly among young children of the lowest socioeconomic groups, elderly persons who live alone, and adults addicted to alcohol and drugs. Some cases are also associated with food faddism or extreme nutritional practices (12).
ETIOLOGY
The conceptual framework of malnutrition developed by the United Nations Children’s Fund (UNICEF) in 1990 is still valid (Fig. 68.2) (13). Inadequate dietary intake and repeated infectious diseases are immediate causes of undernutrition. Infections are a major factor in the etiology of undernutrition as a result of increased nutrient demands, greater nutrient losses, and disruption of metabolic equilibrium. Conversely, the potentiating effects of undernutrition on infections, particularly diarrhea and acute lower respiratory infections, explain most deaths of children between 6 to 59 months of age in developing countries (14, 15, 16). For example, each episode of diarrhea in the first 24 months has been shown to increase the adjusted odds of stunting by a factor of 1.05 (10). This leads to a vicious cycle, in which undernutrition is a health outcome as well as a risk factor for disease and exacerbation of undernutrition (17).
Immediate causes of undernutrition are associated with environmental, economic, and sociopolitical factors, considered underlying and basic causes (see Fig. 68.2). Underlying causes are those that take place at the household level and can be categorized into three main factors: food insecurity; defective maternal and child caring practices; and unsafe water, poor sanitation, and inadequate health services. The first factor leads to inadequate dietary intake, the last cluster to disease, whereas the intermediate factor may contribute to both immediate causes.
Underlying causes are directly influenced by basic causes such as limited education, poverty, and marginalization. The status of women in particular (education, income) tends to influence infant and child feeding. Food security is related to a complex interaction of factors that include agricultural and food production policies, regulation of food marketing and advertisement, and food subsidies. Additionally, sociocultural elements such as religious beliefs and traditions can affect food preferences and net energy intake. A key biologic factor for childhood PEM is maternal malnutrition, resulting in IUGR and low birth weight (18).
In each particular context, the dynamic interrelation among basic and underlying causes can vary. For example, in an environment where enough energy is supplied and sanitation is improved, wasting may be reduced, but stunting could still be constrained by other limiting factors (e.g., micronutrient deficiencies) (19).
PATHOPHYSIOLOGY AND ADAPTIVE RESPONSES
Stunting and acute undernutrition develop gradually over weeks or months, with a series of metabolic and behavioral adjustments that result in decreased nutrient demands and a nutritional equilibrium compatible with a lower level of cellular nutrient availability. If the supply of nutrients is persistently low, the individual can no longer adapt and may die. When undernutrition develops slowly, as is usually the case in stunting, moderate wasting, and
marasmus, individuals are better adapted to their current nutritional status and have a less fragile metabolic equilibrium than do those with more acute undernutrition, as in kwashiorkor of rapid onset.
marasmus, individuals are better adapted to their current nutritional status and have a less fragile metabolic equilibrium than do those with more acute undernutrition, as in kwashiorkor of rapid onset.
Observational and experimental evidence increasingly supports an association between undernutrition during fetal and early postnatal life and increased susceptibility to chronic diseases later in life. The mechanisms underlying this relationship are related to epigenetics, which refers to the ways in which the developmental environment can influence the mature phenotype (20). Epigenetic processes, such as DNA methylation and histone modification, are induced by cues from the developmental environment, thus modulating gene expression (developmental plasticity). Maternal and early postnatal undernutrition can induce a series of thrifty phenotypes as a defensive response of the developing fetus or infant against an immediate challenge. For example, maternal undernutrition reduces the number of nephrons in the child, and this may be related to low mRNA expression resulting from a mutation of the paired box gene 2 (PAX2) during kidney development (21). Fewer nephrons have been related to hypertension later in life (22). Proteinrestricted diets have been associated with reduced promoter methylation and increased expression in the liver of the transcription factor peroxisome proliferator-activated receptor-α (PPAR-α), which causes an increase in circulating concentrations of the ketone β-hydroxybutyrate and glucose (23, 24). Even mild undernutrition can cause phenotypic modifications that affect physiology to aspects of the predicted adult environment (e.g., sparse environment) more precisely (25). If the adaptive change is not appropriate for the subsequent environment (e.g., energyrich environment), the risk of disease increases.
Mild and Moderate Protein-Energy Malnutrition
In the first stages of PEM, a decrease in energy intake is followed by an adaptive reduction in energy expenditure. This includes a decrease in playtime and physical activity in children, which may subsequently develop into overt apathy and unresponsiveness (26, 27, 28, 29). In adults, the need for longer periods of rest is increased, and the capacity for prolonged physical work is reduced (30, 31). When the decrease in energy expenditure cannot compensate for the insufficient intake, energy is mobilized from fat depots, thus leading to weight loss (31). Mobilization of energy from lean body mass also occurs as skeletal muscle protein catabolism contributes energy via conversion of glucogenic amino acids, such as alanine. In children, an additional critical adaptive response is reduction in or cessation of longitudinal growth, which results in chronic undernutrition (stunting). These changes are usually associated with multiple micronutrient deficiencies of variable severity.
As protein and energy deficits progress, the initial adaptation evolves into accommodation, a term coined by Waterlow to describe a response in which normal functions are present but operating at a reduced level (adaptation); survival is achieved at the cost of suppressing or severely reducing certain key physiologic functions (accommodation). For example, protein catabolism is an adaptive mechanism to provide glucose during periods of fasting, such as nighttime sleep. Similarly, prolongation of the half-life of plasma albumin is an adaptive mechanism to reduce protein synthesis. However, if protein synthesis is further curtailed, plasma albumin concentration will fall to abnormal concentrations, thus resulting in clinical edema (32, 33). Similar transitions from adaptation to accommodation can be described for blood pressure, skin characteristics, glomerular filtration rate, and others.
Severe Protein-Energy Malnutrition
Immunity defense responses are diminished in children with SPEM because many immune proteins (e.g., immunoglobulins, complement components, acute phase proteins) are reduced or depleted. Similarly, lymph nodes, adenoids, and the thymus may be reduced in size (34, 35). Phagocytosis, chemotaxis, and intracellular functions are also impaired. As a consequence, the usual clinical signs of infection (inflammation, fever) may not be present in the child with SPEM suffering an acute infectious episode. Instead, signs of the failure of homeostasis, such as hypoglycemia or hypothermia, may appear.
Reduction in hemoglobin concentration and red cell mass almost always accompanies PEM, as a result of bone marrow suppression and reduced oxygen needs, the latter related to depleted skeletal muscle mass (36). These adaptive responses are reversed when and if nutritional rehabilitation is successful. Administration of hematinics to a SPEM patient will not induce a hematopoietic response until dietary treatment produces an increase in lean body mass. Giving iron early in treatment can increase free iron, with promotion of free radicals and their damaging effects, and can also make some infections worse.
Total body potassium decreases in SPEM because of the reduction in muscle proteins and increased urinary and fecal losses. At least one third of the cell’s energy expenditure results from the sodium/potassium-adenosine triphosphatase (Na+/K+-ATPase) pump. In patients with SPEM, this pump slows down because of the diminished energy substrates (ATP). This leads to potassium loss and increased intracellular sodium (37). Water accompanies the sodium influx, and intracellular overhydration may occur. These alterations in cell electrolytes and energy sources may explain, at least in part, the increased fatigability and reduced strength of skeletal muscle, which can even affect respiratory muscles.
Cardiac output, heart rate, and blood pressure decrease; and central circulation takes precedence over peripheral circulation (38, 39). Cardiovascular reflexes are altered, leading to postural hypotension and diminished venous return. These circulatory changes also impair heat generation and loss. Peripheral circulatory failure comparable
to hypovolemic shock may occur. The reduced kidney filtration capacity may result in volume overload and heart failure under relatively moderate water loads.
to hypovolemic shock may occur. The reduced kidney filtration capacity may result in volume overload and heart failure under relatively moderate water loads.
TABLE 68.1 CLASSIFICATION OF SEVERITY OF CURRENT (WASTING) AND PAST OR CHRONIC (STUNTING) PROTEIN-ENERGY MALNUTRITION | |||||||||||||||||||||||||||||||||||||||||||||||||||||||
---|---|---|---|---|---|---|---|---|---|---|---|---|---|---|---|---|---|---|---|---|---|---|---|---|---|---|---|---|---|---|---|---|---|---|---|---|---|---|---|---|---|---|---|---|---|---|---|---|---|---|---|---|---|---|---|
|
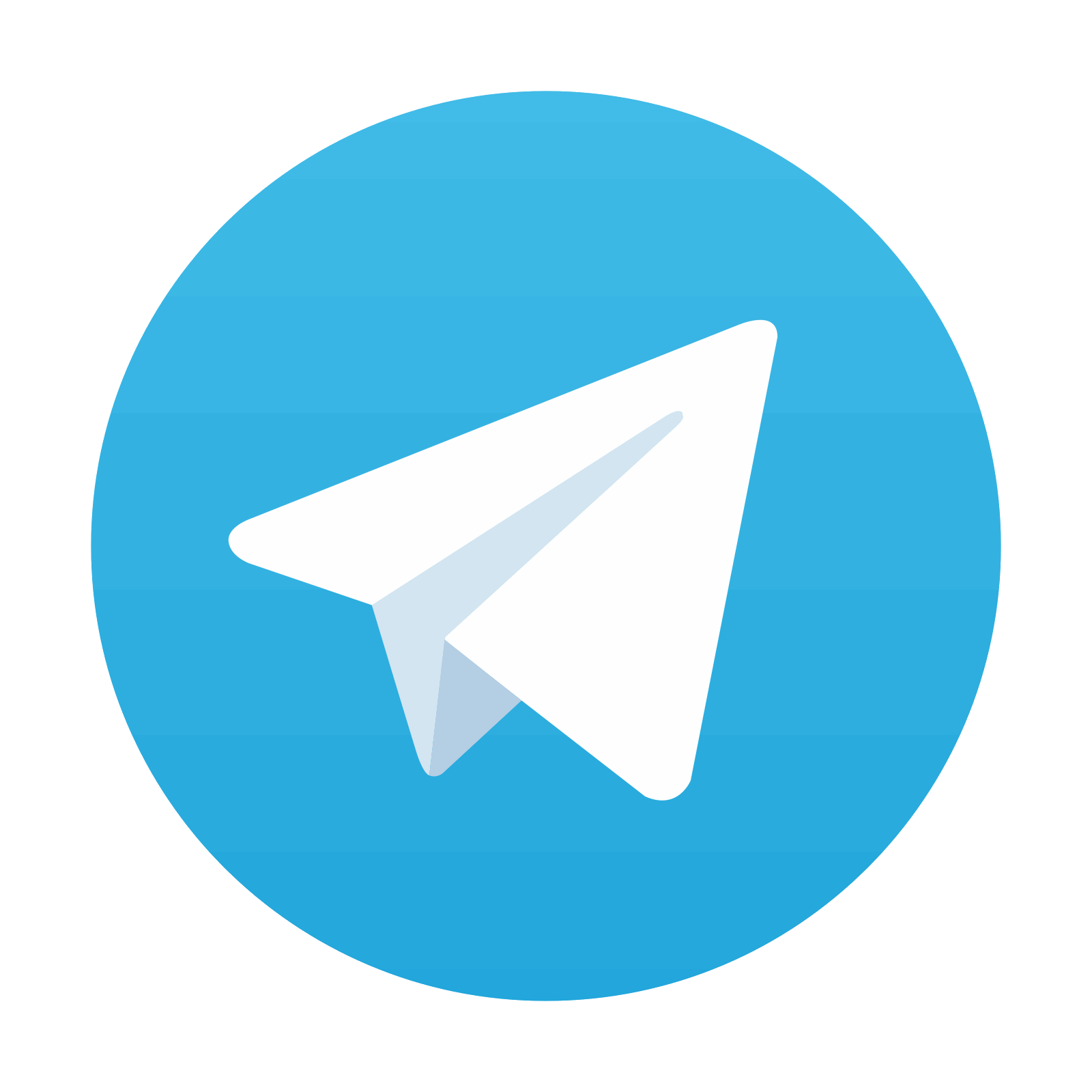
Stay updated, free articles. Join our Telegram channel

Full access? Get Clinical Tree
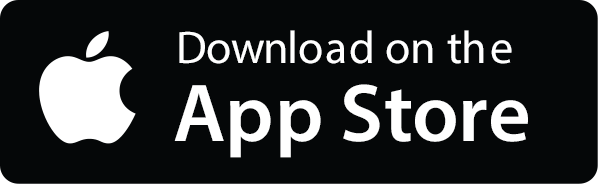
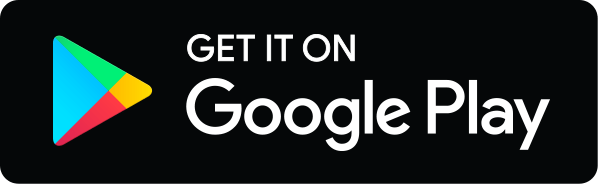