(1)
Canberra, Aust Capital Terr, Australia
Scientific Name
Trifolium pratense L.
Synonyms
Trifolium borysthenicum Gruner, Trifolium bracteatum Schousb., Trifolium lenkoranicum (Grossh.) Roskov, Trifolium pratense var. lenkoranicum Grossh., Trifolium ukrainicum Opperman
Family
Fabaceae, also placed in Papilionaceae
Common/English Names
Beebread, Broad-Leaved Clover, Chilean Clover, Cow Clover, Cowgrass Clover, Early Blooming Clover, June Clover, King’s Crown, Mammoth Clover, Mammoth Red Clover, Meadow Clover, Meadow Honeysuckle, Meadow Trefoil, Medium Red Clover, Multi-Cut Clover, Peavine Clover, Purple Clover, Red Clover, Sweet Clover, Sweet Kitty Clover, Wild Clover
Vernacular Names
Arabic: Barsym ‘Āhamar
Brazil: Trevo Dos Prados
Chinese: Hong Che Zhou Cao, Hong San Ye Cao
Czech: Jetel Luční, Jetel Luční Červený
Danish: Rød-Kløver, Rødkløver
Dutch: Rode Klaver
Eastonian: Aasristik
Esperanto: Ruĝa Trifolio, Trifolio Ruĝa
Finnish: Nurmiapila, Puna-Apila
French: Trèfle Commun, Trèfle Des Prés, Trèfle Rouge, Trèfle Violet, Triolet
Gaelic: Seamair Dhearg
German: Feldknoppern, Fleischklee, Futterklee, Himmelsbrot Honigklee, Hummellust, Rot-Klee, Rotklee, Roter Wiesen-Klee, Steyrerklee, Sügerli, Wiesen-Klee, Weissklee, Zuckerblümli, Zuckerbrot
Greek: Trifilli To Limonio
Hebrew: Tiltan
Hungarian: Réti Here, Lóhere, Vörös Here
Icelandic: Rauðsmari
India: Laal Tipatiya Ghaas, Tripatra (Hindi)
Italian: Trifoglio Dei Prati, Trifoglio Pratense, Trifoglio Rosso, Trifoglio Violetta
Japanese: Aka Kurooba, Aka Tsume Kusa, Aka-Tsumekusa, Murasaki-Tsumekusa Murasaki Tsume Kusa, Reddo Kurooba
Korean: Bulgeuntokkipul
Mexico: Trébol De Los Prados
Norwegian: Raudkløver, Rødkløver Sukkertopp, Sukkertust, Søtekolle
Polish: Koniczyna Czerwona, Koniczyna Łąkowa
Portuguese: Pé-De-Lebre, Trevo-Comum, Trevo-Dos-Prados, Trébol Pratense, Trevo-Violeta
Russian: Klever Krasnyj, Klever Lugovoi
Slovašcina: Črna Detelja, Detelja Črna
Slovencina: Ďatelina Lúčna
Spanish: Trébol Común, Trébol Morado, Trébol Rojo, Trébol Violeta
Swedish: Amerikansk Rödklöver, Rödklöve
Taiwan: Hong Shu Cao
Turkish: Çayır Dutu, Çayır Üçgülü, Dirfil, Yonca
Vietnamese: Chẽ Ba Đồng Cỏ; Ba Chẽ Đỏ
Welsh: Meillion Coch
Origin/Distribution
Red clover is indigenous to Europe, western Asia and northwest Africa but has been introduced and naturalized in many other parts of the world.
Agroecology
Red clover favours cool weather conditions. It is well-adapted to a wide range of soil types and conditions. It tolerate wet conditions but thrives best in well-drained fertile soils. It is found in meadows, wet and dry grassland, woodland, forest margins, field borders, roadsides and paths and widely planted as pastures.
Edible Plant Parts and Uses
Leaves and young flowering heads are edible raw or cooked (Hedrick 1972; Tanaka 1976; Launert 1981; Facciola 1990). The young leaves are harvested before the plant comes into flower and are used in salads and soups or cooked like spinach (Launert 1981) The leaves can be dried, powdered and sprinkled on foods such as boiled rice (Facciola 1990). The dried leaves impart a vanilla flavour to cakes (Schofield 2003) Young flowering heads can be eaten raw in salads (Cribb and Cribb 1976; Schofield 2003). Some recipes with clover flowers are lentil and clover risotto and cauliflower and clover cheese (Roberts 2000). Fresh or dried flowers can be made into a delicate sweet herbal tea (Lust 1974; Facciola 1990). Flowers and seed pods can be dried, ground into a powder and used as a flour. The seeds can be spouted and used in salads (Facciola 1990). Roots can be eaten cooked (Kunkel 1984; Schofield 2003).
The high quercetin concentration and soyasaponin occurrence make the seeds of some Trifolium species (T. repens, T. pratense) a potential source of health beneficial phytochemicals for use in human nutrition (Sabudak and Guler 2009).
Botany
A herbaceous pubescent to glabrescent perennial, with erect to ascending stems, 20–80 cm high and with a fairly deep tap root. Leaves are alternate, trifoliate, basal and cauline, long petiolate in lower leaves, shortly petiolate in upper leaves with membranous, ovate-lanceolate stipules (Plates 1, 2, 3, and 4). Leaflets ovate-elliptic or obovate, 15–30 mm long by 8–15 mm broad, green with a characteristic pale inverted v-pattern in the outer half of the leaflet, base broadly cuneate, margins obscurely serrulate, apex obtuse, rarely retuse. Flowers 30–70, in dense globose or ovoid, terminal, sessile to shortly pedunculate heads, subtended by involucre of stipules (Plates 2, 3, and 4). Calyx with subulate teeth, pubescent or glabrous. Corolla purple or dark pink, standard spatulate, ovary elliptic. Legume ovoid with one yellowish-brown ovoid seed.





Plate 1
Trifoliate leaves of red clover

Plate 2
Close view of flower head and trifoliate leaf (J. Holopainen)

Plate 3
Flower head, hairy leaves and peduncle (T. James)

Plate 4
Top view of flower head (David Llyod)
Nutritive/Medicinal Properties
Plant Phytochemicals
From the plant, glucose, galactose, arabinose, xylose and mannose and amino acids phenylalanine, leucine, isoleucine, methionine, aspartic acid, proline, alanine and histidine were found (Kazakov and Samokish 1980). Phytin and a mixture of Ca and Mg salts of inositol phosphate were also isolated. The amount of ash in the herbage of clover was 8.4 % and the amount insoluble in HC1 was 1.5 %. The amounts of macroelements (mg − %) determined were K+ 1620, Na+ 310, Ca2+ 1240 and Mg2+ 1090. The oil yield for T. pratense was small 0.018 % (weight/fresh weight basis) (Tava et al. 2009). Several classes of compounds were found in the oil, including alcohols, aldehydes, ketones, terpenes, esters, hydrocarbons, phenolics and acids.
A total of eleven flavonoids were identified by liquid chromatography–electrospray mass spectrometry: genistin, isoquercitrin, ononin, daidzein, sissotrin, formononetin, biochanin A, pratensein, pectolinarigenin, pseudobaptigenin and hyperoside (He et al. 1996). The following isoflavones were isolated from red clover: prunetin, irilone, biochanin A, formononetin, pratensein, genistein, calycosin and daidzein (Huang and Tu 2004).
Isoflavone compounds identified in the methanol/water (7:3 v/v) extract of red clover included daidzein, genistin, daidzein, glycitein, orobol, calycosin, genistein, pratensein, pseudobaptigenin, formononetin, texasin, irilone, prunetin, biochanin A, trifoside (prunetin-4′-O-β-d-glucoside), daidzein-7-O-β-d-glucoside-6″-O-malonate, daidzein-7-O-β-d-glucoside-6″-O-acetate, glycetin-7-O-β-d-glucoside, genistein-7-O-β-d-glucoside-6″-O-malonate, calycosin-7-O-β-d-glucoside, calycosin-7-O-β-d-glucoside-6″-O-malonate, prunetin-4′-O-β-d-glucoside-6″-O-malonate, prunetin-4′-O-β-d-glucoside-6″-O-acetate, pratensein-7-O-β-d-glucoside, pratensein-7-O-β-d-glucoside-6″-O-malonate, pseudobaptigenin-7-O-β-d-glucoside, pseudobaptigenin-7-O-β-d-glucoside-6″-O-malonate, pseudobaptigenin7-O-β-d-glucoside-6″-O-acetate, orobol-7-O-β-d-glucoside-6″-O-malonate, 3-methylorobol, 3-methylorobol-7-O-β-d-glucoside, 3-methyloronol-7-O-β-d-glucoside-6″-O-malonate, irilone-4′-O-β-d-glucoside, irilone-4′-O-β-d-glucoside-6″-O-malonate, irilone-4′-O-β-d-glucoside-6″-O-acetate, irilin B-5,7,2′-trihydroxy-6-methoxyisoflavon-7-O-β-d-glucoside, irilin B-5,7,2′-trihydroxy-6-methoxyisoflavon-7-O-β-d-glucoside-6″-O-malonate, sissotrin (biochanin A-7-O-β-d-glucoside), rormosin-7-O-β-d-glucoside, afrormosin-7-O-β-d-glucoside-6″-O-malonate, formononetin-7-O-β-d-glucoside-6″-acetate, formononetin-7-O-β-d-glucoside-6″-O-malonate, ononin (formononetin-7-O-β-d-glucoside), isomer (formononetin-7-O-β-d-glucoside-6″-O-malonate), isomer (formononetin-7-O-β-d-glucoside-6″-O-malonate), isomer (formononetin-7-O-β-d-glucoside-6″-O-acetate), texasin-7-O-β-d-glucoside-6″-O-malonate, biochanin A-7-O-β-d-glucoside-6″-O-malonate, and biochanin A-7-O-β-d-glucoside 6″-O-acetate (Klejdus et al. 2001).
Thirty-one isoflavones were found in red clover leaves and stem: daidzein-G (daidzein), glycitein-G (glycitin), genistein-G (genistin), daidzein-G–M, calycosin-G–M, pratensein-G–M, pseudobaptigenin-G, formononetin-G (ononin), genistein-G–M, pratensein-G–M, daidzein, glycitein, irilone-G, calycosin, pseudobaptigenin-G–M, formononetin-G–M, formononetin-G, biochanin A–G, prunetin-G, genistein, irilone-G–M, irilone-G–M, irilone-G–M, biochanin A–G–M, prunetin-G–M, pseudobaptigenin, biochanin A–G–M, formononetin, irilone, prunetin and biochanin A, where G represents glucosyl or galactosyl moiety, and M, malonyl (in general, glucosyl group, occasionally galactosyl group, was substituted on 7/4′ position of aglycone and malonyl group was linked to 6″ position of sugar moiety) (Wu et al. 2003). Twenty-five isoflavones were detected in the roots except for daidzein, daidzein-G-M, biochanin A-G-M, prunetin-G, prunetin and biochanin A. Twenty-six were found in the flowers except for daidzein, glycitin, genistin, daidzein-G-M and prunetin.
Different red clover cultivars showed significantly different concentrations of individual and total isoflavones (Tsao et al. 2001). The leaf contained the highest overall concentration, followed by the stem, petiole and flower. Biochanin A and formononetin were the predominant isoflavones in all cultivars and all parts, along with eight other minor aglycones, daidzein, genistein, glycitein, irilone, orobol, pratensein, pseudobaptigenin and prunetin, and four minor malonyl glycosides, genistein-7-glucoside-6″-malonate, orobol-7-glucoside-6″-malonate, formononetin-7-glucoside-6″-malonate and biochanin A-7-glucoside-6″-malonate. The isoflavone compositions and concentrations were also found to be different between red clover parts harvested at the early bud stage and the late flowering stage. Clover tissues were found to be rich in the isoflavonoids formononetin and biochanin A, particularly in plants left to wilt for 24 hours (Flythe and Kagan 2010).
The isoflavonoids prunetin, genistein, prunetin-4′-O-β-d-glucopyranoside, prunetin-4′-O-α-d-glucopyranoside, genistein-7-O-β-d-galactopyranoside and (+)-pinitol (1R,2S,3s,4S,5S,6r)-6-methoxycyclohexane-1,2,3,4,5-pentaol were isolated from the aerial parts (Drenin et al. 2011). Concentrations of isoflavones (μg/g) in red clover leaves were daidzein 42 μg, daidzein 0 μg, genistin 560 μg, genistein 0 μg, formononetin-7-O-β-d-glucoside-6″-O-malonate (FGM) 4900 μg, ononin 970 μg, formononetin 600 μg, biochanin A-7-O-β-d-glucoside-6″-O-malonate (BGM) 3300 μg, sissotrin 540 μg and biochanin A 330 μg (de Rijke et al. 2001). The two main isoflavones in red clover were FGM and BGM. Both were subject to decomposition according to formononetin-7-O-β-d-glucoside-6″-O-malonate (FGM) → ononin (FG) → formononetin (F) and biochanin A-7-O-β-d-glucoside-6″-O-malonate (BGM) → sissotrin (BG) → biochanin A (B). The main isoflavones in leaf extracts of T. pratense were biochanin A and formononetin, their 7-O-glucosides, and two glucoside malonate isomers of each of them (de Rijke et al. 2004). The two formononetin glucoside malonate isomers were identified as 7-O-β-d-glucoside 6″-O-malonate and 7-O-β-d-glucoside 4″-O-malonate. The biochanin A glucoside malonate isomers had different structures. The main and later eluting isomer was biochanin A 7-O-β-d-glucoside 6″-O-malonate, and the minor and earlier eluting isomer is 5-hydroxy-7-methoxyisoflavone 4′-O-β-d-glucoside 4″-O-malonate. The most prominent isoflavones found in red clover were formononetin and biochanin A and their corresponding glucosides and malonyl glucoside esters (Swinny and Ryan 2005). They found that postharvest freeze-drying inhibited the conversion of the glycosides to the aglycones, while vacuum drying allowed for maximum conversion of the glycosides to their corresponding aglycones. Air drying produced a low level of the aglycones formononetin and biochanin A, and oven drying promoted decarboxylation of the malonyl glucosides to the acetyl glucosides. Exposure to enhanced UV-B radiation resulted in an increase in total formononetin and biochanin A isoflavone levels, indicating that harvest during a period of high ambient UV-B radiation may be appropriate for maximum yield. The levels of caffeic acid and flavonols also increased by about 40 and 250 %, respectively, on exposure to enhanced UV-B radiation.
The emission of 24 compounds comprising monoterpenes, homoterpenes, esters, hydrocarbons and aldehydes from red clover plant was quantified in the laboratory, of which eight showed increased emission rates after herbivory by Spodoptera littoralis caterpillars (Kigathi et al. 2009). The most abundant compounds included the monoterpene (E)-β-ocimene (30–50 % of the total), the isomeric monoterpene (Z)-β-ocimene, the monoterpene linalool, the sesquiterpene (E)-β-caryophyllene, the sesquiterpene-derived homoterpene 4,8-dimethyl-1,3,7-nonatriene (DMNT), (E,E)-α-farnesene, 1-octen-3-ol, and methyl salicylate. Other compounds included α-pinene, sabinene, β-pinene, limonene, 1,8-cienole, indole, (Z)-jasmone, (E)-β-farnesene, germacrene D, (Z)-3-hexenyl acetate, benzyl alcohol, dodecane, undecane, octylaldehyde, nonanal and decanal.
Volatile components of red clover hay and silage comprised alkanes, alkenes, aldehydes, alcohols, ketones, esters, acids, terpenes, unidentified compounds and others (Figueiredo et al. 2007). In hay, reductions of the percentages of alcohols, such as 3-methylbutanol and 1-hexanol, of aldehydes and of low boiling point ketones were observed A sesquiterpene (β-farnesene; ca. 10 %) and a phytol degradation product (6,10,14-trimethyl-2-pentadecanone; ca. 12 %) were the most abundant compounds detected in hay. In silage, as a result of the fermentation of fresh red clover, esters (ca. 46 %) were a more representative class of compounds. Silage esters included methyl esters, ethyl esters, acetates, non-linear esters, phenylethyl esters and others.
A synthetic pyridine derivate, 2-(2-fluoro-6-nitrobenzylsulfanyl) pyridine-4-carbothioamide, was found to be a good elicitor of isoflavonoid production in red clover suspension culture (Kašparová et al. 2012). After 48-hours application of 1 μmol/l concentration, maximum contents of genistin (11.60 mg/g DW), daidzein (8.31 mg/g DW) and genistein (1.50 mg/g DW) were recorded, and the production of these isoflavonoids was significantly increased, when compared with the control, by 152, 151 and 400 %. The tested substance showed to be an effective elicitor of phenylpropane metabolism.
Leaf Phytochemicals
The leaves were found to contain 81 % water, 4 % protein, 0.7 % fat, 2.6 % fibre and 2 % ash (Duke and Ayensu 1985). Based on over 500 analyses, Miller (1958) reported that green red clover forage had the following nutrient composition: 12.4–34.87 % protein (av. 18.2), 3.2–5.9 % fat (av. 4.0 %), 12.7–30.8 % crude fibre (av. 24.2), 7.0–13.6 % ash (av. 8.8), and 37.1–49.7 % N-free extract (av. 44.8 %), 0.58–3.21 % Ca (av. 1.76), 0.24–0.53 % P (av. 0.29), 1.49–2.94 % K (av. 2.10 %), 0.36–0.57 % Mg (av. 0.45), 0.016–0.032 % Fe (av. 0.03), 7.3–10.3 ppm Cu (av. 8.8 ppm), and 121–464 ppm Mn (av. 159 ppm). The leaf-protein concentrate (59 % protein) comprised 6.4 % arginine, 2.5 % histidine, 5.4 % threonine, 1.7 % tryptophan, 9.5 % leucine, 5.3 % isoleucine, 1.7 % methionine, 6.87. lysine, 6.1 % phenylalanine and 6.8 %. valine.
Major volatile components identified in red clover leaves were (Z)-3-hexenyl acetate, (Z)-3-hexenol and (E)-β-ocimene and (Z)-β-ocimene (Buttery et al. 1984). In red clover leaves, a total of 31 different phenolics were identified: cis–trans-phaselic acid, cis-clovamide, daidzein-G, daidzein-G-M, calycosin-G, calycosin-G-M, genistein, genistein-G, genistein-G-M, pratensein, irilone, irilin B, methylorobol, methylorobol-G, methylorobol-G-M, formononetin, formononetin-G, formononetin-G-M, afrormosin-G-M, maackiain, maackiain-G-M, biochanin A, biochanin A-G-M, prunetin, texasin, texasin-G-M, quercetin-glucoside, quercetin-galactoside, quercetin-G-M, kaempferol-G and pseudobaptigenin-G-M (Saviranta et al. 2010a). The most abundant isoflavones and flavonoids were biochanin A glycoside malonate (G-M), formononetin-G-M and quercetin-G-M. Elevated ozone (mean 32.4 ppb) increased the total phenolic content of leaves and also had minor effects on the concentrations of individual compounds. Elevated ozone increased the net photosynthesis rate of red clover leaves before visible injuries by 21–23 %.
The acetone-soluble lipids of red clover leaves largely comprised galactosyl-1-glycerol (I) and l linoleate (Weenink 1961). The fatty acids of the galactolipids contained (mole%) linolenic, 95.8 %; linoleic, 1.9 %; and palmitic, 2.3 %. Oleic, stearic and palmitoleic acids were not detected. Triglycerides were not found in the acetone-soluble lipid fraction. The hexane-soluble fraction contained galactose 4.2 %, glycerol 2.4 %, nonsaponifiable matter 43.3 % and fatty acids 26.0 %, the remainder being acidic, nonfatty material. Free sterols (0.8 %), sterol esters (0.57 %), triglycerides (1.5 %), diglycerides (0.6 %) and normal hydrocarbons (0.38 %) were isolated from the acetone-soluble lipids of red clover leaves (Weenink 1962). Free and combined sterol contained β-sitosterol, and the hydrocarbons consisted of homologous odd- and even-numbered series C15 to C25 with C25 predominating. The diglycerides contained over 50 % of palmitic acid, whereas the sterol esters and triglycerides contained linoleic and linolenic acids as the main components. The composition of the acetone-insoluble fraction (molar proportions) of red clover leaves comprised phosphatidycholine 37 %, phosphatidylglycerol 23 %, phosphatidylethanolamine 15 %, phosphatidylinositol 2 %, uncharacterized acidic compounds 13 % and unknown compounds 10 % (Weenink 1964). An unusual C14 unsaturated fatty acid was found in the phosphatidylglycerol fraction. Trans-Δ3-hexadecenoic acid (16:1t) was found to occur exclusively in the phosphatidylglycerol fraction of red clover leaves (Weenink and Shorland 1964). The fatty acid composition (mol %) of phosphatidylglycerol in red clover leaves comprised 16:0 (20 %), 16:1t (40 %), 18:0 (1 %), 18:1 (7 %), 18:2 (13 %) and 18:3 (25 %) (Murata et al. 1982). In response to waterlogging, the concentrations of biochanin A and biochanin A-7-O-glucoside malonate, biochanin A-7-O-glucoside and genistein-7-O-glucoside in the leaves increased two- to threefold after a lag period of 3 weeks because of disturbed root nodulation (De Rijke et al. 2005). The other isoflavones detected—formononetin, formononetin-7-O-glucoside malonate and formononetin-7-O-glucoside—did not show any significant changes related to waterlogging. After restoring normal soil water conditions, the concentrations of biochanin A and its glucoside and glucoside malonate rapidly returned to the initial values, whereas the concentration of genistein-7-O -glucoside remained high.
Red clover leaves were found to accumulate several μmol of phaselic acid [2-O-caffeoyl-l-malate] per gram fresh weight (Sullivan and Zarnowski 2010). Their study found that phaselic acid was likely formed by transfer of a caffeoyl moiety to malic acid, although the existence of a second C3′H capable of hydroxylating p-coumaroyl-malate could not be definitively excluded. They identified coumarate 3′-hydroxylase (CYP98A44), a cytochrome P450 which was capable of hydroxylating p-coumaroyl-shikimate but not p-coumaroyl-malate in the biosynthesis of phaselic acid. The gene for cytochrome P450 C3′H (CYP98A44) was cloned from red clover.
Root Phytochemicals
Formononetin and the new isoflavonoid glycosides formononetin-7-O-β–d-galactopyranoside and inermin-3-O-β–d-galactopyranoside were isolated from Trifolium pratense roots (Drenin et al. 2008). The following volatile compounds were identified from red clover roots: butyl acetate, E-2-hexenal, α-pinene, benzaldehyde, 6-methyl-5-hepten-2-one, limonene, acetophenone, methyl benzoate, nonanal, octanoic acid and decanal (Tapia et al. 2007). The results showed that the clover root borer (Hylastinus obscures) was attracted to root volatiles of 1.5-year-old extracts but not to those from 2.5-year-old extracts. A 10 μg dose of methyl benzoate and E-2-hexenal attracted the insect, whereas the same dose of limonene repelled the red clover borer. The following volatiles and free fatty acids were detected in the dichloromethane extract of 9-month-old red clover roots: 1-nonene, 3-octanol, 2-pentyl furan, benzyl alcohol, limonene, maltol, benzoic acid, o-acetyl-p-cresol, eugenol, caryophyllene oxide, pentadecanal, lauric acid, palmitic acid, oleic acid and stearic acid (Manosalva et al. 2011). The four long-chain free fatty acids lauric, palmitic, oleic and stearic acids were found to be main components in the extract. Further studies on the response of clover root borer to volatiles emitted from red clover roots afforded the identification of ethanol, E-2-hexenal, hexanal, 3-octanone, limonene and α-pinene (Palma et al. 2012). For females, ethanol and E-2-hexenal were attractive at one or more of the tested doses, while hexanal, 3-octanone, R-limonene and S-limonene were repellent at one or more of the tested doses. In a much earlier study, of 15 root compounds, 10 were attractive to the borer clover root borer (Hylastinus obscures) (Kamm and Buttery 1984). None of the attractive compounds were competitive with crude extracts of diseased roots when tested individually. Certain combinations of ethyl laurate, ethyl benzoate, methyl salicylate, eugenol, hexadecanal and chavicol were competitive with crude extracts of diseased root in laboratory tests. Adult borers were not attracted to individual compounds or mixtures in preliminary field tests.
A total of 28 phenolic compounds were tentatively identified in red clover roots (Saviranta et al. 2010b). The most abundant phenolics in pot-grown roots were formononetin glycoside malonate (G-M) (1.51–4.26 mg/g), formononetin (2.21–3.57 mg/g) and biochanin A (1.73–2.17 mg/g), whereas field-grown roots were rich in formononetin-G-M (3.90–4.27 mg/g), maackiain-G-M (2.35–3.02 mg/g) and pseudobaptigenin-G-M (1.80–2.58 mg/g). Concentrations were affected by the growth stage and ozone exposure slightly affected the total phenolic content in roots and also had minor effects on individual compounds. The isoflavonoids formononetin, formononetin-7-O-β-d-galactopyranoside and inermin-3-O-β-d-galactopyranoside were isolated from the roots (Drenin et al. 2011).
Flower/Seed Phytochemicals
T. pratense seeds were found to contain quercetin, soyasaponin I, 22-O-glucoside and 22-O-diglucoside of soyasaponin I and astragaloside VIII (Oleszek and Stochmal 2002).
More than 50 compounds were identified in the essential oil samples of red and white Austrian clover flowers (Buchbauer et al. 1996). The main constituents (concentration >2 %) were maltol (8.2 %), linalool (4.2 %), 1-phenylethyl alcohol (3.2 %), phenol (2.9 %), phenylethyl acetate (2.7 %), acetophenone (2.4 %), and (Z)-3-hexenyl acetate (2.2 %) for red clover flowers and maltol (5.3 %), linalool (3.8 %), phenol (3.6 %), phenylethyl acetate (3.3 %) and 2-phenylethyl alcohol (2.8 %) for white clover flowers. Major volatile components identified in red clover flowers were acetophenone, methyl cinnamate and 1-phenylethanol (Buttery et al. 1984). Major volatile components identified in red clover seed pods were (E)-β-ocimene and (Z)-β-ocimene, longifolene and an unidentified sesquiterpene hydrocarbon (Buttery et al. 1984).
Twenty flavonoid glycoside malonates were detected in red clover flower extract (Lin et al. 2000). Eight were identified as genistin 6″-O-malonate (39), formononetin 7-O-β-d-glucoside 6″-O-malonate (40), biochanin A 7-O-β-d-glucoside 6″-O-malonate (41), trifoside 6″-O-malonate (42), irilone 4′-O-β-d-glucoside 6″-O-malonate (43), pratensein 7-O-β-d-glucoside 6″-O-malonate (44), isoquercitrin 6″-O-malonate (45), and 3-methylquercetin 7-O-β-d-glucoside 6″-O-malonate (46). About 15 other flavonoids and clovamides were present in this extract. Floral procyanidins of red clover comprised a range of oligomeric procyanidin ions (DP of 2–11) (Sivakumaran et al. 2004). The thiolysis reaction products indicated a mean degree of polymerization (mDP) of 9.3 with epicatechin (81 %) as the abundant flavan-3-ol extension unit and the terminating units dominated by catechin (95 %).
Antioxidant Activity
All T. pratense leaf extracts especially water and ethyl acetate extracts showed good antioxidant activity when evaluated using DPPH, hydroxyl, superoxide anion and nitric oxide radical scavenging and lipid peroxidation assays (Kaurinovic et al. 2012). In the DPPH assay, the IC50 (50 % of reduction) values in μg/ml were as follows: 17.47 μg for H2O (water), 17.81 μg for EtOAc (ethyl acetate), 20.36 μg for Et2O (diethyl ether), 29.47 μg for n-BuOH (n-butanol), and 34.19 μg for CHCl3(chloroform) extract compared to synthetic antioxidants tert-butylated hydroxytoluene (BHT) and butylated hydroxyanisole (BHA)BHT (14.31 μg) and BHA (11.08 μg). In scavenging of superoxide and NO radicals, H2O extract demonstrated highest activity but was lower than that of BHT and BHA. The lowest antioxidant activity was expressed by the CHCl3 (IC50 = 92.37 μg/ml for superoxide and IC50 = 58.46 μg/ml for NO radical) extract. Good antioxidant scavenging of OH radical was shown by the H2O (IC50 = 18.44 μg/ml) and EtOAc (IC50 = 19.79 μg/ml) extracts, which showed higher ability than BHT and BHA (IC50 = 22.17 μg/ml). The worst effect on the neutralization of all the radicals studied in-vitro was shown by the n-BuOH extract. In lipid peroxidation suppressing activity, H2O extract exhibited the highest activity followed by EtOAc extract. In in-vivo experiments in the liver homogenate and blood of mice after their treatment with extracts of T. pratense leaves, or in combination with CCl4, all the extracts decreased the GSH (reduced glutathione) and GSHPx (glutathione peroxidase) content compared with control. Treatment with the EtOAc extract yielded an increase in GSHR (glutathione reductase) activity, whereas the other four extracts caused a statistically significant decrease of this enzyme, which was in agreement with the action of this enzyme on GSH. Only H2O extract produced a statistically significant increase in Px (peroxidase) activity. The LPx (lipid peroxidation activity) was lowered in the liver homogenate of animals treated with all extracts. The CAT (catalase) increased in the treatments with EtOAc, n-BuOH and H2O extracts, but the other extracts caused no essential changes of CAT with respect to control. A statistically significant decrease of XOD (xanthine oxidase) activity was observed in the experimental animals treated with last three extracts (EtOAc, n-BuOH and especially H2O). The amount of total phenolics in T. pratense leaf extracts ranged from 0.16 mg GAE/g d.e. (CHCl3 extract) to 0.43 mg GAE/g d.e. (EtOAc extract) (Kaurinovic et al. 2012). A significant amount of these compounds were observed in the H2O extract (0.34 mg GAE/g d.e.). Comparatively lower amount of total flavonoids was determined in the n-BuOH extracts, while the smallest quantity of was found in the Et2O and CHCl3 extracts. Quercetin glycosides and flavonoids (kaempferol-3-O-glucoside, quercetin-3-O– glucoside, luteolin-7-O– glucoside and apigenin-7-O-glucoside) were detected in EtOAc extract, while the presence of phenolic acids (such as caffeic acid) and flavonoids (luteolin, apigenin, naringenin and kaempferol) was confirmed in the H2O extract. Additionally, two phytoestrogens (daidzein and genistein) were detected in the H2O extract. The results suggested that the water and ethyl acetate leaf extracts afforded the best in-vitro and in-vivo antioxidant activity in protecting against carbon tetrachloride toxicity.
Anticancer Activity
Slater et al. (2002) found that in mice fed a diet supplemented with red clover isoflavones, the prostatic epithelium displayed a significant increase in the production of oestrogen receptor beta and the adhesion protein E-cadherin but a decrease in transforming growth factor beta 1. These proteins were oestrogenically induced markers of proliferation, maintenance of histological architecture, preservation of cell phenotype and reduction of the potential for neoplastic and metastatic transformation. Their study suggested that red clover isoflavones presented a nontoxic dietary treatment for prostatic hyperplasia and a reduction in the potential for neoplastic transformation. Jarred et al. (2003) found that red clover-derived dietary isoflavones (RC) suppressed prostate growth in-vivo in the aromatase knockout (ArKO) mouse exhibiting lifelong elevation of androgens leading to prostate enlargement. Following 28 days of RC diet, ArKO ventral prostate (VP), anterior prostate, and seminal vesicle weights were reduced to wild-type mouse weights, although testis and body weights remained unaltered. Stereological analysis of VPs revealed a reduction in all components of the tissue, particularly the lumen. The RC diet reduced ArKO serum testosterone and dihydrotestosterone to WT levels. In comparison to castration and oestrogen administration, the dietary isoflavones were shown to be antiandrogenic rather than weakly oestrogenic. Engelhardt and Riedl (2008) found that 1-year treatment of 20 men (mean age 65 years) with isoflavone extract from red clover elicited a significant reduction of 33 % in prostate-specific antigen (PSA) level and a slight decrease in prostate volume. Sexual hormone levels (total testosterone, oestrogen, luteotropic hormone, follicle-stimulating hormone and dehydroepiandrosterone sulphate) did not changed throughout the study. However, a significant increase in all three liver transaminases after 3 months was recorded. The International Prostate Symptom Score showed a mean value of 7.9 at baseline and 6.68 after 12 months. Sexual function was not influenced by the treatment. Daily oral administration of 60 mg of an isoflavone extract was well tolerated and caused no side effects. In in-vitro studies, red clover isoflavones at 25.0 μg/ml significantly inhibited the proliferation by 18.86 % and promoted the apoptosis of human benign prostatic hyperplasia (BPH) stromal cells by 18.54 % compared to negative and blank control (Chen et al. 2010).
Studies by Ye et al. (2012) suggested that higher concentrations of formononetin inhibited the proliferation of prostate cancer cells (LNCaP and PC-3), while the most striking effect was observed in LNCaP cells. They further found that formononetin inactivated extracellular signal-regulated kinase1/kinase2 (ERK1/ERK2) mitogen-activated protein kinase (MAPK) signalling pathway in a dose-dependent manner, which resulted in increased the expression levels of BCL2-associated X (Bax) mRNA and protein, and induced apoptosis in LNCaP cells.
A series of cases in which Trifolium pratense was used in breast disease with hyperoestrogenic symptoms was reported by Parvu (2004). Several patients with cystic mastosis and breast cancer appeared to do well. In a 3-year randomized, double-blind, placebo-controlled pilot trial of healthy women aged 35–70 years with a family history of breast cancer, treatment with red clover isoflavones did not adversely affect breast density, skeletal strength or cardiovascular status (Powles et al. 2008). In postmenopausal women, endometrial status was not adversely affected. The adverse event profile was similar between red clover isoflavones, and placebo and endocrine status did not differ. Treatment with red clover isoflavones was found to be safe and well tolerated in healthy women. The principal isoflavones genistein and daidzein contained in red clover extracts acted as weak oestrogenic compounds when administered alone in ER positive breast cancer cells (Mannella et al. 2012). However, when provided in association with physiological amounts of estradiol, red clover extract acted as oestrogen antagonist on remodelling of actin cytoskeleton. The results indicated that isoflavones contained in red clover acted as natural selective oestrogen receptor modulators in the presence of physiological amounts of oestrogens.
The 95 % ethyl alcohol extract of one of red clover significantly inhibited the metabolism of [3H]benzo(a)pyrene [B(a)P] and decreased the level of binding of B(a)P to DNA by 30–40 % (Cassady et al. 1988). Its active isoflavone component, biochanin A, also decreased the metabolism of B(a)P by 54 % in comparison to control cultures and decreased B(a)P-DNA binding by 37–50 % at a dose of 25 μg/ml indicating its potential as a chemopreventive agent. A separate study showed that biochanin A was able to decrease dimethylbenz[a]anthracene (DMBA)-induced DNA damage in MCF-7 cells by curbing cytochrome P450 (CYP) 1 enzymes through the interference of XRE-dependent transactivation (Chan et al. 2003). Enzyme kinetic studies also indicated that biochanin A inhibited both CYP1A1 and CYP1B1 enzymes. The study illustrated that the red clover isoflavone could protect against polycylic aromatic hydrocarbon-induced DNA damage.
Red clover isoflavones, namely, daidzein, genistein, formononetin and biochanin, were found to inhibit COX enzyme activity in both the murine macrophage cell line RAW 264.7 and human monocytes (Lam et al. 2004). Within the range of 1–40 μM in RAW 264.7 cells and 10–100 μM in human monocytes, isoflavones were able to reduce significantly the synthesis of prostaglandin E2 and/or thromboxane B2 indicating COX inhibition. They concluded that it was possible that the lower rates of some cancers in populations with a high intake of dietary isoflavones could be linked to their inhibition of COX activity. Another study found that biochanin A and/or formononetin, predominant isoflavones from red clover, may exert anticarcinogenic effects directly by acting as competitive substrates for human cytochrome CYP1B1 or indirectly through their metabolites daidzein and genistein, which inhibited CYP1B1(Roberts et al. 2004). Three of the biochanin A metabolites (5,7,3′-trihydroxy-4′-methoxyisoflavone, 5,7,8-trihydroxy-4′-methoxyisoflavone and 5,6,7-trihydroxy-4′-methoxyisoflavone) were characterized. Daidzein (Ki = 3.7 μM) exhibited competitive inhibition of CYP1B1 7-ethoxyresorufin O-deethylase activity, and genistein (Ki = 1.9 μM) exhibited mixed inhibition. In-vitro studies in MCF-7 breast carcinoma cells showed that biochanin A, the red clover isoflavone, may act as a antagonist/agonist to bind on the aryl hydrocarbon receptor that mediates the carcinogen activation pathway (Han et al. 2006). Treating the cells with biochanin A alone caused the accumulation of CYP1A1 mRNA and an increase in CYP1A1-specific 7-ethoxyresorufin O-deethylase (EROD) activity in a dose-dependent manner. A concomitant treatment with 7,12-dimethylbenz[a]anthracene (DMBA) and biochanin A markedly reduced the DMBA-inducible EROD activity and CYP1A1 mRNA level. Biochanin A competitively inhibited the metabolic activation of DMBA, as measured by the formation of the DMBA-DNA adducts. In MCF-7 breast cancer cells stably transfected with CYP19, biochanin A inhibited aromatase activity and hampered cell growth attributing to the enzyme activity (Wang et al. 2008). Additionally, biochanin A significantly reduced CYP19 mRNA abundance in the oestrogen receptor-negative breast cancer cells SK-BR-3. Luciferase reporter gene assays also revealed that biochanin A could repress the transcriptional control dictated by the promoter regulation. The present study illustrated that biochanin A inhibited CYP19 and aromatase activities and gene expression.
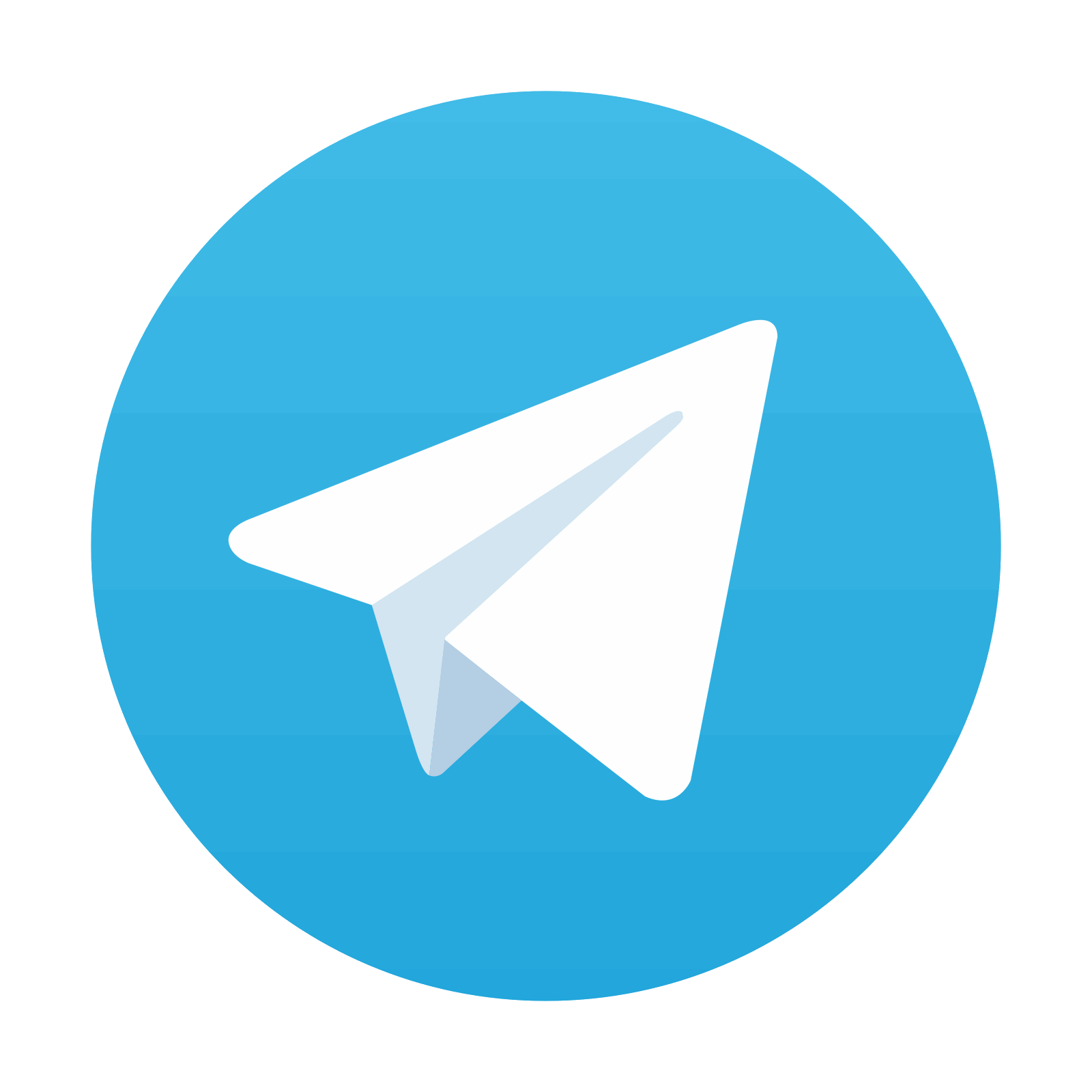
Stay updated, free articles. Join our Telegram channel

Full access? Get Clinical Tree
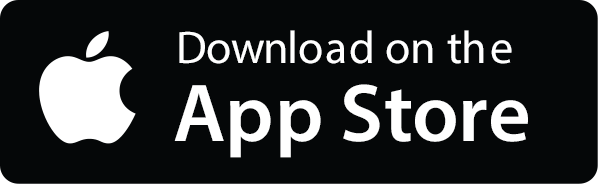
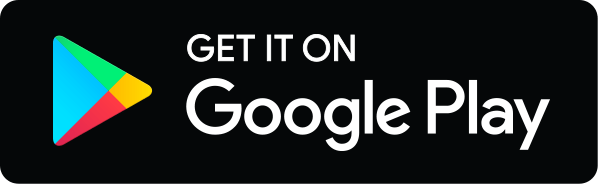