CHAPTER 35 Potassium, Calcium, and Phosphate Homeostasis
K+ HOMEOSTASIS
Hypokalemia is one of the most common electrolyte disorders in clinical practice and can be observed in as many as 20% of hospitalized patients. The most frequent causes of hypokalemia include administration of diuretic drugs, surreptitious vomiting (e.g., bulimia), and severe diarrhea. Gitelman’s syndrome (a genetic defect in the Na+-Cl− symporter in the apical membrane of distal tubule cells) also causes hypokalemia (see Chapter 33, Table 33-3). Hyperkalemia is also a common electrolyte disorder and is seen in 1% to 10% of hospitalized patients. Hyperkalemia often occurs in patients with renal failure, in patients taking drugs, including angiotensin-converting enzyme (ACE) inhibitors and K+-sparing diuretics, in patients with hyperglycemia (i.e., high blood sugar), and in the elderly. Pseudohyperkalemia, a falsely high plasma [K+], is caused by traumatic lysis of red blood cells during blood drawing. Red blood cells, like all cells, contain K+, and lysis of red blood cells releases K+ into plasma, thereby artificially elevating plasma [K+].
The large concentration difference of K+ across cell membranes (approximately 146 mEq/L) is maintained by the operation of Na+,K+-ATPase. This [K+] gradient is important in maintaining the potential difference across cell membranes. Thus, K+ is critical for the excitability of nerve and muscle cells, as well as for the contractility of cardiac, skeletal, and smooth muscle cells (Fig. 35-1).
After a meal, the K+ absorbed by the gastrointestinal tract enters the ECF within minutes (see Fig. 35-3). If the K+ ingested during a normal meal (≈33 mEq) were to remain in the ECF compartment (14 L), plasma [K+] would increase by a potentially lethal 2.4 mEq/L (33 mEq added to 14 L of ECF):
Cardiac arrhythmias are produced by both hypokalemia and hyperkalemia. The electrocardiogram (ECG; see Fig. 35-2 and Chapter 16) monitors the electrical activity of the heart and is a fast and easy way to determine whether changes in plasma [K+] influence the heart and other excitable cells. In contrast, measurement of plasma [K+] by the clinical laboratory requires a blood sample, and values are often not immediately available. The first sign of hyperkalemia is the appearance of tall, thin T waves on the ECG. Further increases in plasma [K+] prolong the PR interval, depress the ST segment, and lengthen the QRS interval of the ECG. Finally, as plasma [K+] approaches 10 mEq/L, the P wave disappears, the QRS interval broadens, the ECG appears as a sine wave, and the ventricles fibrillate (i.e., manifest rapid, uncoordinated contractions of muscle fibers). Hypokalemia prolongs the QT interval, inverts the T wave, and lowers the ST segment of the ECG.
REGULATION OF PLASMA [K+]
As illustrated in Figure 35-3 and Table 35-1, several hormones, including epinephrine, insulin, and aldosterone, increase uptake of K+ into skeletal muscle, liver, bone, and red blood cells by stimulating Na+,K+-ATPase, the 1Na+-1K+-2Cl− symporter, and the Na+-Cl− symporter in these cells. Acute stimulation of K+ uptake (i.e., within minutes) is mediated by an increased turnover rate of existing Na+,K+-ATPase, 1Na+-1K+-2Cl−, and Na+-Cl− transporters, whereas a chronic increase in K+ uptake (i.e., within hours to days) is mediated by an increase in the quantity of Na+,K+-ATPase. The rise in plasma [K+] that follows K+ absorption by the gastrointestinal tract stimulates secretion of insulin from the pancreas, release of aldosterone from the adrenal cortex, and secretion of epinephrine from the adrenal medulla. In contrast, a decrease in plasma [K+] inhibits the release of these hormones. Whereas insulin and epinephrine act within a few minutes, aldosterone requires about an hour to stimulate uptake of K+ into cells.
Table 35-1 Major Factors, Hormones, and Drugs Influencing the Distribution of K+ between the Intracellular and Extracellular Fluid Compartments
ALTERATIONS IN PLASMA [K+]
Several factors can alter plasma [K+] (Table 35-1). These factors are not involved in the regulation of plasma [K+] but rather alter the movement of K+ between the intracellular fluid (ICF) and ECF and thus cause the development of hypokalemia or hyperkalemia.
Acid-Base Balance
Metabolic acidosis increases the plasma [K+], whereas metabolic alkalosis and respiratory alkalosis decreases it. In contrast, respiratory acidosis has little or no effect on the plasma [K+]. Metabolic acidosis produced by the addition of inorganic acids (e.g., HCl, H2SO4) increases plasma [K+] much more than an equivalent acidosis produced by the accumulation of organic acids (e.g., lactic acid, acetic acid, keto acids). The reduced pH (i.e., increased [H+]) promotes the movement of H+ into cells and the reciprocal movement of K+ out of cells to maintain electroneutrality. This effect of acidosis occurs in part because acidosis inhibits the transporters that accumulate K+ inside cells, including Na+,K+-ATPase and the 1Na+-1K+-2Cl− symporter. In addition, movement of H+ into cells occurs as the cells buffer changes in [H+] of the ECF (see Chapter 36). As H+ moves across the cell membranes, K+ moves in the opposite direction, and thus cations are neither gained nor lost across cell membranes. Metabolic alkalosis has the opposite effect; plasma [K+] decreases as K+ moves into cells and H+ exits.
Plasma Osmolality
The osmolality of plasma also influences the distribution of K+ across cell membranes. An increase in the osmolality of ECF enhances the release of K+ by cells and thus increases extracellular [K+]. Plasma [K+] may increase by 0.4 to 0.8 mEq/L with a 10 mOsm/kg H2O elevation in plasma osmolality. In patients with diabetes mellitus who do not take insulin, plasma [K+] is often elevated, in part because of the lack of insulin and in part because of the increase in plasma [glucose] (i.e., from a normal value of ≈100 mg/dL to as high as ≈1200 mg/dL), which increases plasma osmolality. Hypoosmolality has the opposite action. The alterations in plasma [K+] associated with changes in osmolality are related to changes in cell volume. For example, as plasma osmolality increases, water leaves cells because of the osmotic gradient across the plasma membrane (see Chapter 1). Water leaves cells until the intracellular osmolality equals that of the ECF. This loss of water shrinks cells and causes [K+] in cells to rise. The rise in intracellular [K+] provides a driving force for the exit of K+ from cells. This sequence increases plasma [K+]. A fall in plasma osmolality has the opposite effect.
K+ EXCRETION BY THE KIDNEYS
The kidneys play a major role in maintaining K+ balance. As illustrated in Figure 35-3, the kidneys excrete 90% to 95% of the K+ ingested in the diet. Excretion equals intake even when intake increases by as much as 10-fold. This balance in urinary excretion and dietary intake underscores the importance of the kidneys in maintaining K+ homeostasis. Although small amounts of K+ are lost each day in feces and sweat (approximately 5% to 10% of the K+ ingested in the diet), this amount is essentially constant, is not regulated, and therefore is relatively less important than the K+ excreted by the kidneys. K+ secretion from blood into tubular fluid by cells of the distal tubule and collecting duct system is the key factor in determining urinary K+ excretion (Fig. 35-4).
Because acid-base balance, plasma osmolality, cell lysis, and exercise do not maintain plasma [K+] at a normal value, they do not contribute to K+ homeostasis (Table 35-1). The extent to which these pathophysiological states alter plasma [K+] depends on the integrity of the homeostatic mechanisms that regulate plasma [K+] (e.g., secretion of epinephrine, insulin, and aldosterone).
Because K+ is not bound to plasma proteins, it is freely filtered by the glomerulus. When individuals ingest 100 mEq of K+ per day, urinary K+ excretion is about 15% of the amount filtered. Accordingly, K+ must be reabsorbed along the nephron. When dietary K+ intake increases, however, K+ excretion can exceed the amount filtered. Thus, K+ can also be secreted.
The proximal tubule reabsorbs about 67% of the filtered K+ under most conditions. Approximately 20% of the filtered K+ is reabsorbed by the loop of Henle, and as with the proximal tubule, the amount reabsorbed is a constant fraction of the amount filtered. In contrast to these segments, which can only reabsorb K+, the distal tubule and collecting duct are able to reabsorb or secrete K+. The rate of K+ reabsorption or secretion by the distal tubule and collecting duct depends on a variety of hormones and factors. When 100 mEq/day of K+ is ingested, it is secreted by these nephron segments. A rise in dietary K+ intake increases K+ secretion. K+ secretion can increase the amount of K+ that appears in urine so that it approaches 80% of the amount filtered (Fig. 35-4). In contrast, a low-K+ diet activates K+ reabsorption along the distal tubule and collecting duct so that urinary excretion falls to about 1% of the K+ filtered by the glomerulus (Fig. 35-4). The kidneys cannot reduce K+ excretion to the same low levels as they can for Na+ (i.e., 0.2%). Therefore, hypokalemia can develop in individuals placed on a K+-deficient diet. Because the magnitude and direction of K+ transport by the distal tubule and collecting duct are variable, the overall rate of urinary K+ excretion is determined by these tubular segments.
In individuals with advanced renal disease, the kidneys are unable to eliminate K+ from the body. Therefore, plasma [K+] rises. The resulting hyperkalemia reduces the resting membrane potential (i.e., the voltage becomes less negative), and this reduced potential decreases the excitability of neurons, cardiac cells, and muscle cells by inactivating fast Na+ channels, which are critical for the depolarization phase of the action potential (Fig. 35-1). Severe, rapid increases in plasma [K+] can lead to cardiac arrest and death. In contrast, in patients taking diuretic drugs for hypertension, urinary K+ excretion often exceeds dietary K+ intake. Accordingly, K+ balance is negative, and hypokalemia develops. This decline in extracellular [K+] hyperpolarizes the resting cell membrane (i.e., the voltage becomes more negative) and reduces the excitability of neurons, cardiac cells, and muscle cells. Severe hypokalemia can lead to paralysis, cardiac arrhythmias, and death. Hypokalemia can also impair the ability of the kidneys to concentrate the urine and can stimulate the renal production of NH4+, which affects acid-base balance (see Chapter 36). Therefore, maintenance of high intracellular [K+], low extracellular [K+], and a high [K+] gradient across cell membranes is essential for a number of cellular functions.
CELLULAR MECHANISM OF K+ SECRETION BY PRINCIPAL CELLS IN THE DISTAL TUBULE AND COLLECTING DUCT
Figure 35-5 illustrates the cellular mechanisms of K+ secretion by principal cells in the distal tubule and collecting duct. Secretion from blood into the tubule lumen is a two-step process: (1) uptake of K+ from blood across the basolateral membrane by Na+,K+-ATPase and (2) diffusion of K+ from the cell into tubular fluid via K+ channels. Na+,K+-ATPase creates a high intracellular [K+] that provides the chemical driving force for exit of K+ across the apical membrane through K+ channels. Although K+ channels are also present in the basolateral membrane, K+ preferentially leaves the cell across the apical membrane and enters the tubular fluid. K+ transport follows this route for two reasons. First, the electrochemical gradient of K+ across the apical membrane favors its downhill movement into tubular fluid. Second, the permeability of the apical membrane to K+ is greater than that of the basolateral membrane. Therefore K+ preferentially diffuses across the apical membrane into tubular fluid. The three major factors that control the rate of K+ secretion by the distal tubule and the collecting duct are
Every change in K+ secretion results from an alteration in one or more of these factors.
Intercalated cells reabsorb K+ via an H+,K+-ATPase transport mechanism located in the apical membrane (see Chapter 36). This transporter mediates uptake of K+ in exchange for H+. The pathway for exit of K+ from intercalated cells into blood is unknown. Reabsorption of K+ is activated by a low K+-diet.
REGULATION OF K+ SECRETION BY THE DISTAL TUBULE AND COLLECTING DUCT
Regulation of K+ excretion is achieved mainly by alterations in K+ secretion by principal cells of the distal tubule and collecting duct. Plasma [K+] and aldosterone are the major physiological regulators of K+ secretion. Antidiuretic hormone (ADH) also stimulates K+ secretion; however, it is less important than plasma [K+] and aldosterone. Other factors, including the flow rate of tubular fluid and acid-base balance, influence secretion of K+ by the distal tubule and collecting duct. However, they are not homeostatic mechanisms because they disturb K+ balance (Table 35-2).
Table 35-2 Major Factors and Hormones Influencing K+ Excretion
Plasma [K+]
Plasma [K+] is an important determinant of K+ secretion by the distal tubule and collecting duct. Hyperkalemia (e.g., resulting from a high-K+ diet or from rhabdomyolysis) stimulates secretion of K+ within minutes. Several mechanisms are involved. First, hyperkalemia stimulates Na+,K+-ATPase and thereby increases K+ uptake across the basolateral membrane. This uptake raises intracellular [K+] and increases the electrochemical driving force for exit of K+ across the apical membrane. Second, hyperkalemia also increases the permeability of the apical membrane to K+. Third, hyperkalemia stimulates secretion of aldosterone by the adrenal cortex, which as discussed later, acts synergistically with plasma [K+] to stimulate secretion of K+. Fourth, hyperkalemia also increases the flow rate of tubular fluid, which as discussed later, stimulates secretion of K+ by the distal tubule and collecting duct.
Chronic hypokalemia (plasma [K+] <3.5 mEq/L) occurs most often in patients who receive diuretics for hypertension. Hypokalemia also occurs in patients who vomit, undergo nasogastric suction, have diarrhea, abuse laxatives, or have hyperaldosteronism. Hypokalemia occurs because excretion of K+ by the kidneys exceeds the dietary intake of K+. Vomiting, nasogastric suction, diuretics, and diarrhea can all decrease ECF volume, which in turn stimulates secretion of aldosterone (see Chapter 34). Because aldosterone stimulates excretion of K+ by the kidneys, its action contributes to the development of hypokalemia.
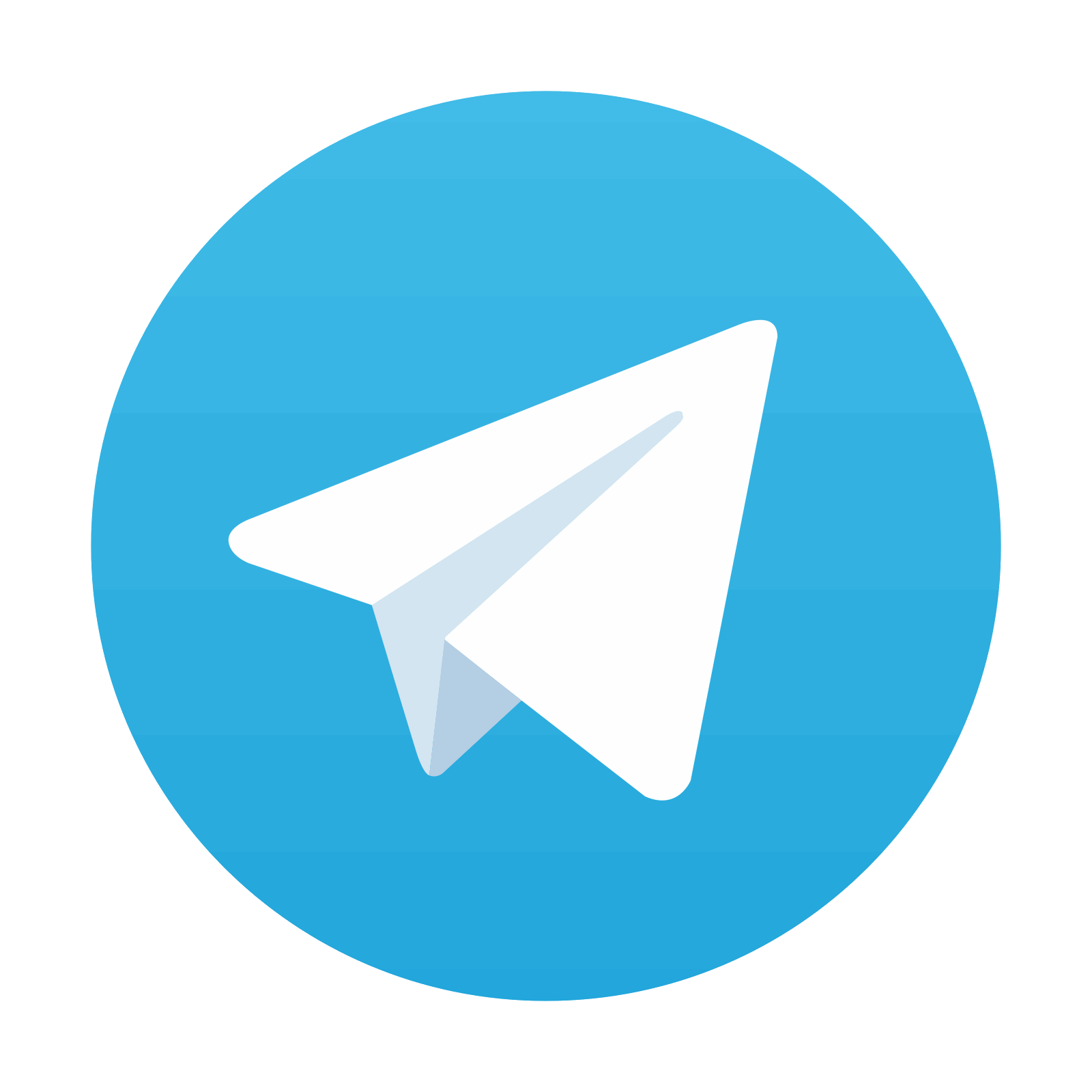
Stay updated, free articles. Join our Telegram channel

Full access? Get Clinical Tree
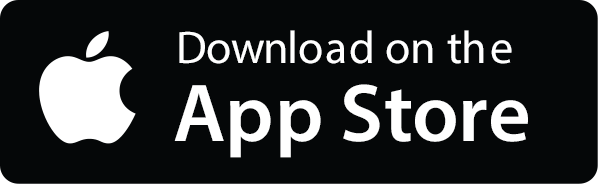
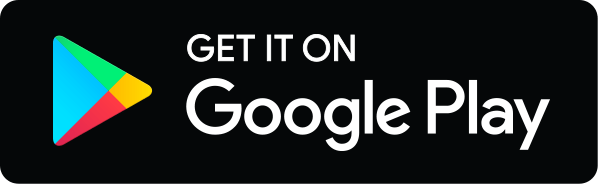