Point-of-Care Testing
Advances in a range of analytical and fabrication technologies, such as (1) thin film sensors, (2) semiconductor engineering, (3) plastic molding, (4) microfluidics, (5) nanotechnology, and (6) consumer electronics, have made it possible to adapt most of the methods used in the laboratory to the point-of-care setting.78 This prompts the question as to whether there is the beginning of a general movement of testing away from the central laboratory to the point of care.112,113,120 Christensen and associates believe that point-of-care testing (POCT) is one of the key enablers required to deliver changes in healthcare provision through disruptive innovation, namely, radically changing the way that healthcare is delivered.23
Although early POCT technologies were very much associated with acute clinical needs (e.g., medical emergencies), it is more appropriate to define POCT as “when a test is performed at the time at which the test result enables a decision to be made and an action taken that leads to an improved health outcome.” Other terms used to describe POCT have included (1) “bed side,”97 (2) “near patient,”32 (3) “physician’s office,”87 (4) “extralaboratory,”108 (5) “decentralized,”6 (6) “off site,” (7) “satellite,” (8) “kiosk,” (9) “ancillary,” and (10) “alternative site” testing50; many of the latter descriptors identify a specific location where the test is intended to be performed, while others clearly describe the adjunctive nature of the testing site to the central laboratory. “Self-testing” should be added to these descriptions to reflect that there is an increasing repertoire of tests that are, performed by patients themselves.
In the hospital setting, POCT has been described in (1) coronary care units,31 (2) intensive care units,46 (3) surgical wards,36 (4) emergency rooms,41,81 and (5) pediatric units,42,119 and for monitoring patients with acute49,54 and chronic conditions.1 More testing is now being performed using POCT in primary care, both to help in rapid diagnosis and to determine the appropriateness of triage to secondary care, as well as to manage patients with chronic conditions. The “wish list” for the capability to perform POCT in primary care is extensive!92 However a systematic review conducted in 1997 provided little high quality evidence to support this utility.57
Improving clinical and process outcomes has the potential to reduce errors and wastage of resources while also reducing the cost of care and increasing societal gain. This argument can be applied simply to the testing process (Figure 20-1) or to the wider aspects of the whole patient journey. Thus, such a radical change in the provision of healthcare can be seen to have several potential advantages, while exposing stakeholders to different risks, and hence disadvantages (Box 20-1).
Figure 20-1 Schematic representation of the key steps in requesting, delivering, and using a diagnostic test result.
Thus an important feature of POCT is identification of healthcare needs and performance requirements associated with meeting those needs, which must be complemented by the quality management and reimbursement strategies required to ensure “fitness for purpose.”
The following sections of this chapter describes the technology available for POCT and the organizational factors that are important when POCT is implemented in a healthcare setting. The chapter concludes with a short discussion of the future of POCT.
Analytical and Technological Solutions
The commercial POCT market is reported to make up nearly 30% of the total in vitro diagnostics (IVD) market and is worth in excess of $13 billion (U.S.).68 Within this market are a wide variety of devices that are difficult to classify succinctly but that can be divided into smaller, single-use, hand-held devices and larger benchtop devices that may be single- or multiple-use items (Table 20-1). An additional group, is the wearable devices, an example of which was the GlucoWatch G2 Biographer.124
Although all manufacturers of diagnostic medical devices aim to make their devices simple to use and to reduce the risk of error, these goals assume a greater imperative for POCT devices because they are likely to be used by personnel who have not received laboratory training and who lack the skills required to detect when devices may not be working correctly and are giving incorrect results. Thus much of the ingenuity and engineering that have gone into producing the myriad of POCT devices that exist today have been directed toward achieving these goals. The novel mechanical design and engineering solutions are matched in importance by software that makes the device operate in a way that minimizes the risks of mechanical failure and operator error.
This section focuses on some of the key technologies that are used in hand-held and benchtop devices, including specific design features that are aimed at preventing misuse or errors, and refers to some commercial examples. Box 20-2 provides a summary of the ideal requirements for a POCT device against which new technologies and devices are assessed. Readers requiring additional information are referred to more comprehensive texts75,109 or to vendors of POCT devices.
Hand-Held, Single-Use Devices
No hard and fast definition of a hand-held device as compared with a benchtop instrument is available, but one typical definition used by Kricka and Thorpe is that hand-held devices are up to about the size of the electronic book readers that are now gaining popularity.79 Hand-held devices first appeared in the form of paper strips many centuries ago for the testing of urine. Test strips as we know them today were introduced in the 1940s and 1950s through companies such as Ames and Roche, while the first disposable immunochemical test could be deemed to be the relatively rapid (2 hours) pregnancy test presented by Wampole in 1970.
To provide some insight into the wealth of different designs that make up disposable hand-held devices, four categories of devices will be reviewed in some detail (1) urine or blood dipsticks; (2) glucose and coagulation strips together with accompanying meters; (3) lateral flow devices including immunosensors; and (4) cartridge or cassette devices. A key attribute of all these devices is that they are used only once and therefore are referred to as single-use devices. Test strips were originally designed to be read by the operator, and with some devices this is still the case, but many strips are now used in conjunction with meters or reading devices. Advances in meter design have been greatly influenced by advances in consumer electronics, and the ability of POCT devices to meet the goals mentioned previously is a consequence of both the design of the strip or analytical system and the meter with which the strip or device is used. Control and communication systems are a major component of all POCT devices other than qualitative or semiquantitative strip tests that do not require a reader or measurement device. In even the smallest device, a control subsystem coordinates all the other systems and ensures that all required processes for an analysis take place in the correct order. Operations that require control include (1) insertion or removal of the strip, cartridge, or cassette; (2) temperature control; (3) sample injection or aspiration; (4) sample detection; (5) mixing; timing of incubation and detection processes (such as absorbance measurement); and (6) waste removal. Fluid movement often is accomplished by mechanical means through pumps or centrifugation, and by fluidic properties, such as surface tension; the latter can be a critical element in the design of simple strip tests and in microfabricated systems.72
Urine and Blood Dipsticks
Dipsticks are relatively simple in construction and are composed of a pad of porous material, such as cellulose, that is impregnated with reagent and then dried.134 The reaction is indicated by a visible color reaction that is compared with a chart. More complex pads are composed of several layers, the uppermost of which is a semipermeable membrane that prevents red cells from entering the matrix. Despite their simplicity, critical operator factors can affect the result, and manufacturers have tried to overcome some of these problems through innovative designs. Thus not all strips now require that the whole pad be covered with the sample to obtain a reliable result. Although reactions in dipsticks do not always proceed to completion, the time between placement of the sample on the pad and comparison of the resulting color with a color chart is less critical for some strips than for others.
Further progress with these single-stick devices has included the use of multiple pads for measurement of (1) different concentrations of the same analyte, such as hemoglobin and glucose134; (2) both albumin and creatinine (semiquantitative) to obtain an albumin-to-creatinine ratio114; and (3) up to 10 different urine analytes; as well as the use of a reflectance meter to quantitate the color change.85 Table 20-2 lists some of the tests performed by single or multipad dipsticks and the chemistry used for analysis.
TABLE 20-2
Examples of Single or Multipad Stick Tests
Test | Sample | Chemistry |
Acetaminophen | Whole blood | Acyl dehydrogenase |
Alanine aminotransferase | Whole blood | Alanine/glutamate |
Albumin | Whole blood, urine | Dye binding |
Cholesterol | Whole blood | Cholesterol oxidase |
Creatinine | Whole blood, urine | Copper complexation |
Glucose | Whole blood | Glucose oxidase |
Lactate | Whole blood | Lactate dehydrogenase |
Uric acid | Whole blood | Uricase |
Alcohol | Urine | Alcohol dehydrogenase |
Bilirubin | Urine | 2,4-Dichloroaniline |
Hemoglobin | Urine | Peroxidase activity |
Leukocyte esterase | Urine | Pyrrole amino ester hydrolysis |
Ketones | Urine | Sodium nitroprusside reaction |
Nitrite | Urine | p-Arsanilic acid reaction |
pH | Urine | Double indicator principle |
Protein | Urine | Protein error of indicators |
Specific gravity | Urine | Polyacid pH change |
Urobilinogen | Urine | Ehrlich’s reaction |
Glucose Strips and Meters
Point-of-care glucose testing using disposable strips represents a market that was worth nearly $10 billion (U.S.) in 2008.68 The size of the market has driven considerable innovation in strip and meter design in an effort to produce devices produce results comparable with those obtained through laboratory methods. Significant improvement has been noted in attributes such as ease of use; however, limitations of this technology are particularly important when testing is conducted in hospitals.
Glucose strips are biosensors in that they all use an enzyme as the recognition agent; glucose oxidase (GO), hexokinase (HK), or glucose dehydrogenase (GDH) with photometric (reflectance) or, more commonly, electrochemical detection may be used.98 Each strip comprises several layers, each having a specific function. When blood is added, both water and glucose must pass into the film or analytical layer; for photometric systems, erythrocytes must be excluded. These processes are achieved by what is called the separating layer that contains various components, including glass fiber fleeces, membranes, and special latex formulations. In photometric systems, a spreading layer is important for fast homogeneous distribution of the sample, whereas electrochemical strips use capillary fill systems. The support layer is usually a thin plastic material that in the case of reflectance-based strips may have reflective properties. Additional reflectance properties have been achieved through the inclusion of substances such as titanium oxide, barium sulfate, and zinc oxide.
A major advance in the development process was the use of ferrocene and its derivatives as immobilized mediators in the construction of electrochemical glucose strips.33 The strip is composed of an Ag-AgCl reference electrode and a carbon-based active electrode, both manufactured using screen printing technology with the ferrocene or its derivatives contained in the printing ink. The sample is placed in the sample observation window, and the hydrophilic layer serves to direct the sample over the reagent layer. Conversion of glucose is accompanied by the reduction of ferrocene and the release of electrons (Figure 20-2).33 Another significant advance has been the use of techniques such as capillary filling to ensure that the right amount of sample is in place.
In practice, glucose strips are produced in large batches; after extensive quality assurance procedures, each batch is given a code that is stored in a magnetic strip on the underside of each test strip. This code describes the performance of the batch, including the calibrating relationship between the photometric or electrochemical signal and the concentration of glucose. For many years, it was necessary to provide a means for recalibrating a meter (e.g., providing the algorithm on a bar coded strip that could be read by the meter) when a new batch of strips was employed, which led to errors when patients forgot to perform the recalibration.115 However, strips now can be manufactured with sufficient consistency such that recalibration between different lots is no longer required.8
Parallel with advances in strip technology has been the evolution of the consumer electronics industry, in particular the miniaturization of electronics.95 The latter, together with the use of amperometric methods, has enabled the production of smaller meters, nonwipe strips, sample volumes <1 µL, less need to clean instrument optics, and results obtained within 5 seconds. Some meters also include substantial information technology (IT) capability, including the ability to lock users out if they have not completed required procedures such as quality control (QC) and the capacity to store and download patient results. In conjunction with docking stations and data managers is the capability to institute the type of informatics procedures that are required of central laboratory testing, such as identification and possible lockout of operators, together with transmission of results to the laboratory information system (LIS), along with accompanying patient information.
Thus the capability of glucose meters has advanced significantly over the years and is such that appropriately trained and motivated patients with diabetes can monitor their own glucose with a reasonable degree of assurance and tailor their insulin therapy accordingly. The use of glucose meters in sick or hospitalized patients has been more problematic. For example, a major problem with glucose oxidase methods was their susceptibility to the ambient tension of oxygen in blood, which may vary substantially in critically ill patients. This interference was essentially removed by using glucose dehydrogenase as the enzyme, but several reports and at least one death have been the result of maltose interference in glucose dehydrogenase strips that use the pyrroloquinolinequenone form of the enzyme (GDH-PQQ), the maltose being given as part of the patient’s therapy.43 Subsequently, improved strips such as the StatStrip by Nova BioMedical (Waltham, Mass) appear to overcome this problem.58
In addition, evaluation reports indicate that this newer design is not affected by hematocrit, unlike most other glucose strips. Hematocrit can vary substantially in young infants and in critically ill adults with the glucose concentration being inversely related to the hematocrit; those who look after the critically ill need to be aware of this phenomenon. Many interferent issues and the resulting performance gap between glucose meters and laboratory results have been brought into sharp focus with the adoption of procedures such as tight glycemic control (TGC) in intensive care unit (ICU) patients.132 Although meter performance has undoubtedly improved over the years, reports show that the analytical performance of most current meters is not good enough for them to be used in TGC protocols.14 An International Organization for Standardization (ISO) document reviews the performance goals required for meters in hospitals, in physician and practitioner offices, and for patients performing self-monitoring.62
Coagulation Strips and Meters
The clinical need to monitor anticoagulant therapy in both acute and chronic medical settings has been well defined, and the value of POCT devices has been demonstrated in several studies.36,53 The number of patients requiring long-term anticoagulant therapy is growing, and coagulation monitoring is one of the largest sectors of the POCT market after glucose monitoring and pregnancy testing. General features of a typical coagulation meter include a design that utilizes fingerstick samples in the case of home or clinic monitoring, with minimal demands on the user in terms of sample application. The reaction usually takes place in a cassette or test strip that is maintained at a temperature above 37 °C to maximize reaction kinetics and minimize changes in outside temperature. The latest generation of portable meters generally used in hospital locations is much easier to use, compared with earlier systems, and requires smaller specimen volumes.
Manufacturers have used a variety of systems to detect the clotting event in coagulation meters, but newer detection technologies that are classified broadly as electrochemical are used in HemoSense and Roche CoaguChek XS instruments. With the HemoSense InRatio2 coagulation meter (HemoSense Inc., Inverness Medical Innovations, Waltham, Mass) for measurement of prothrombin time (PT), clotting is detected by impedance rather than by current.64 Because this device is likely to be used by patients, it has various features designed to make QC automatic or to require minimal involvement of the patient. These include a test strip that has three channels—one for the patient test and two for different concentrations of internal QC reference materials; all three channels operate simultaneously when a patient sample is applied to the strip (Figure 20-3). Similar features of integrated on-board QC operate in other smaller meters or devices likely to be used by patients, such as the ITC ProTime Microcoagulation System (ITC, Edison, NJ). Several studies have shown that the ProTime device can be used satisfactorily by patients for self-testing purposes.99,100 Many meters have QC lockout features that prevent operation of the device when QC results are not within prescribed limits, as well as software to detect common errors such as expired reagents and incorrect specimen placement or volume. The Roche CoaguChek XS (Roche Diagnostics, Mannheim, Germany) uses a similar amperometric detection principle. Thrombin-activated cleavage of thromboplastin in the strip leads to generation of electrochemically active phenylenediamine, and when the current generated by this species reaches a certain value, the PT can be determined.105 Environmental changes in the strip that might occur as the result of incorrect handling are detected by an integrated QC system in the strip, which, under the Clinical Laboratory Improvement Amendments (CLIA) of the United States, means there is no need to run liquid quality controls on the strip.
One persistent problem that remains for point-of-care coagulation testing is the poor correlation between POCT devices and coagulation analyzers in the central laboratory. This is a particular problem for activated clotting time (ACT), for which a so-called gold standard for comparison does not exist.106 Reports in the literature indicate that despite improvements and progress with standardization, lack of agreement can still be a problem.69,125
Lateral Flow Strips
Many POC tests use lateral flow strips, usually for immunoassays; the original application that was filed as a patent in 1979 was called an immunochromatograph.48,111 These devices are biological sensors in which the recognition agent is usually an antibody that binds to the analyte. Detection of the binding event or signal transducer usually occurs via an optical mechanism—reflectance or fluorescence spectrophotometry—for quantification. A typical sandwich immunoassay format in a lateral flow is the Roche Troponin T test shown in Figure 20-4.3,30 Two antibodies are present in the strip: one labeled with gold particles, and the other conjugated to biotin. When whole blood is added to the test well, it flows through the fleece, separating the plasma, and the troponin binds to the two antibodies. The antibody-troponin complex flows along the cellulose nitrate strip and reaches the capture zone, which contains streptavidin. The latter binds to the biotin in the troponin complex, immobilizing it and appearing as a purple band due to the presence of gold particles on one of the antibodies. Unreacted gold-labeled antibody is able to move down the strip, where it is captured by a synthetic peptide that contains the epitope of human cardiac troponin T, which is visualized as a separate second band. This band indicates that the sample has flowed along the strip and the device has worked correctly, which represents a form of built-in control.
Figure 20-4 Schematic diagram of a lateral flow immunoassay strip for troponin T. Rights were not granted to include this figure in electronic media. Please refer to the printed book. (Courtesy Roche Diagnostics.) Roche Diagnostics
A growing application of lateral flow strip immunoassay is for infectious disease testing and the rapid detection of various infectious antigens and antibodies, including (1) Chlamydia trachomatis,65 (2) group A streptococci,21 (3) Helicobacter pylori,51 (4) infectious mononucleosis,40 and (5) human immunodeficiency virus.67
Several immunosensor-based devices have been developed that are capable of measuring a panel of analytes, such as cardiac markers,4 drugs of abuse tests,17 and fertility tests.12 With these devices, a mixture of antibodies is placed at the origin, and complementary antibodies for various analytes are located at varying positions along the porous strip. In the case of drugs of abuse, devices are designed such that positive responses are obtained only if the concentration is above a precalibrated cutoff value.17
Although lateral flow devices are the most common form of POCT immunosensor, other formats include a flow-through design.130 Alternative detection mechanisms to reflectance or absorbance are also being investigated; these include (1) surface plasmon resonance, (2) evanescent wave, (3) fixed-polarized ellipsometry, and (4) diffraction.
Cartridge or Cassette Devices
Another major category of single-use devices consists of integrated cartridges or cassettes that operate in conjunction with a hand-held meter. The most common example is the i-Stat Analyzer (Abbott Laboratories, Chicago, Ill), which when introduced some 2 decades ago, measured only blood gases, electrolytes, and glucose but now has a menu that includes creatinine, certain coagulation parameters, and troponin.11,39 To achieve the full menu requires different combinations of cartridges. Each of these contains sensors or electrodes as wafer structures constructed with thin metal oxide films using microfabrication techniques directly comparable with those used in the computer industry.35 Another important component of the cartridge is the microfluidic network, which directs and controls the movement of calibration liquids and blood sample to each of the sensors.72 This device was one of the first to introduce the concept of electronic QC, a procedure that checks the status of the cartridge prior to analysis of a patient sample. Although this is a valuable procedure, it is agreed by most laboratory professionals that it cannot substitute for conventional QC procedures.135 The size of the device allows it to be used directly at the bedside and via the use of a docking station or cradle connected to the LIS or hospital information system (HIS); patient results can be captured and retained in the patient record. Some hospitals use these systems for all critical care testing; others retain them as a backup or use them in locations where testing is done relatively infrequently.
The need for clean air fabrication means that the cost of I-stat–type devices is relatively high, which has driven research into alternative and less costly ways to manufacture such complex cartridges. One example is the Epoc system (Epocal Inc., Ottawa, Ontario, Canada), which uses technology from the smart-card industry by manufacturing blood gas, electrolyte, and immunoassay devices as credit card–sized items on 35-mm tape-on-reel format.80 Similar to the I-Stat system, the cards are used in conjunction with a hand-held meter, but this type of technology has significant advantages in terms of manufacturing cost.
In a similar vein, microfluidic cards are now being developed as an alternative to more conventional cassettes for a number of applications, including polymerase chain reaction (PCR)-based tests for infectious agents. In one approach under development by Atlas Genetics (Bristol, United Kingdom; www.atlasgenetics.com/accessed April 14, 2011), the disposable card (Figure 20-5) has many functions, which use a common architecture to ensure a simple, low-cost device that combines sample preparation, PCR amplification, and electrochemical detection. The lower molding contains fluidic channels through which reagents are moved using a series of bellows and valves created by the flexible rubber layer. This enables an instrument to remain isolated from the fluids in the card by simply applying pressure to the card to run the assay. The foil on the bottom of the card has excellent barrier properties for long-term storage of wet reagents, while providing a low-resistance path for rapid thermocycling.55
Figure 20-5 Schematic diagram of a microfluidic card employing a molecular test for Chlamydia trachomatis. Rights were not granted to include this figure in electronic media. Please refer to the printed book. (Courtesy Atlas Genetics, Bristol, United Kingdom.) Atlas Genetics, Bristol, UK
Multiple-Use Cartridges and Benchtop Systems
A significant proportion of multiple-use systems are used for critical care testing in locations such as the ICU, the surgical suite, and the emergency room. The technology for measuring blood gases and associated parameters has existed for many years, but the menus of so-called blood gas instruments have recently been extended to include biosensors for parameters such as glucose, lactate, and urea. Although they use similar technology to that described previously, they differ in that the sensors are designed to be reusable.34 To improve convenience for non–laboratory trained users such as nurses and doctors, the sensors have been incorporated with reagents and calibrators into a single cartridge or pack that is placed in the body of a small to medium-sized, portable critical care analyzer (Figure 20-6). Each pack will measure a certain number of samples during a particular time period, after which replacement is a relatively simple procedure. This type of technology is available as the (1) Instrumentation Laboratory GEM Premier 3000 and 4000 (Instrumentation Laboratory GEM, Bedford, Mass),9,10 (2) Radiometer ABL800 (Radiometer ApS, Copenhagen, Denmark),84 and (3) Siemens Healthcare Diagnostics Rapidpoint 400 (Deerfield, Ill).86
Figure 20-6 The GEM Premier 3000 critical care analyzer. (Courtesy Instrumentation Laboratory, Lexington, Mass.)
Other key developments for critical care devices include liquid calibration systems that use a combination of aqueous base solutions and conductance measurements to calibrate the pH and PCO2 electrodes, with oxygen being calibrated with an oxygen-free solution and room air.90 This dispenses with the need for gas bottles. Another important innovation is the integration of automated QC packages into these analyzers, which ensure that QC samples are analyzed at regular intervals. These comprise packs or bottles of QC material that are contained within the instrument and are sampled at predetermined intervals with onboard software interpreting the results and generating alerts, if necessary. Such devices can even be remotely monitored and programmed to respond to problems with instruments located long distances from the central laboratory.56
Critical care POCT instruments are also available for measuring various hemoglobin species and performing CO-oximetry determinations. The latter relies upon multiwavelength spectrophotometry where light absorption by hemolyzed blood is measured at up to 60 or more wavelengths to determine the concentration of the five hemoglobin species.16 Manufacturers have extended the capability of multiwavelength spectrophotometry to measure bilirubin directly in whole blood.13,117
The menu of critical care tests is also being extended to parameters that can be measured only by immunoassay. Two particularly important parameters required for acute care are troponin and beta–chorionic gonadotropin (CG). As has been described, both can be measured using hand-held devices, usually using lateral flow or similar technology, but the precision and accuracy of such techniques are occasionally not fit for the purpose for which they are intended. Thus manufacturers have devised larger instruments for immunoassay measurement that are capable of comparable analytical quality to laboratory instruments, but they use whole blood, thus facilitating their use close to patient care. The Radiometer AQT90 Flex uses an all-in-one dry-reagent concept and the well-established technique of time-resolved fluorescence used in laboratory-based instruments. Whole blood or plasma samples are used, and the instrument includes an automated sample aspiration module, thus minimizing the time that operators have to interact with the instrument and reducing the risk of preanalytical error (Figure 20-7). The sample is added automatically to the cup containing assay-specific reagents, and in the case of troponin, the assay is a one-step immunofluorometric assay using two biotinylated antibodies for capture and one europium-labeled antibody for detection. Whole blood results are corrected for hematocrit using conductance measurement and are available in less than 20 minutes.52
Figure 20-7 Touchscreen of the Radiometer AQT Flex analyzer. (Courtesy Radiometer, Copenhagen, Denmark.)
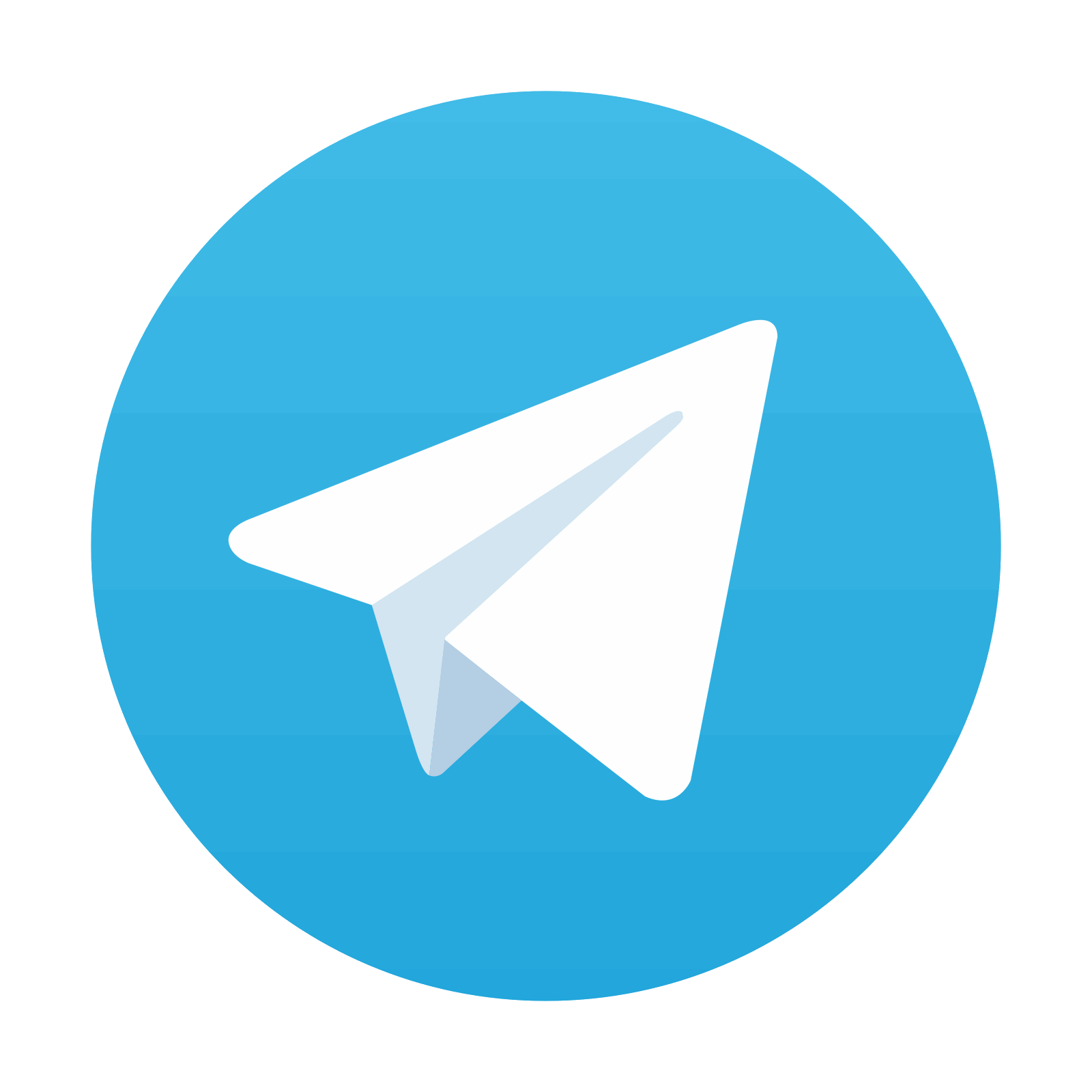
Stay updated, free articles. Join our Telegram channel

Full access? Get Clinical Tree
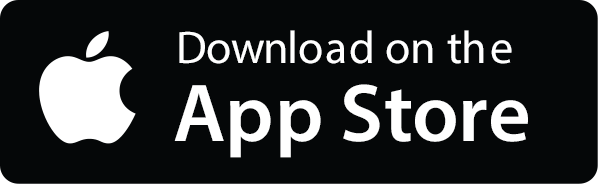
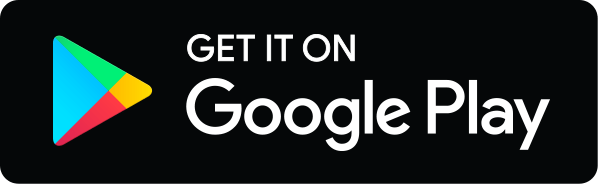