Physiology of the Muscular System
GENERAL FUNCTIONS
If you have any doubts about the importance of muscle function to normal life, think about what it would be like without it. It is hard to imagine what life would be like if this matchless power were lost. However, as cardinal as it is, movement is not the only contribution muscles make to healthy survival. They also perform two other essential functions: production of a large proportion of body heat and maintenance of posture.
2. Heat production. Muscle cells, like all cells, produce heat by the process known as catabolism (discussed in Chapters 4 and 30). The heat produced by just one cell is inconsequential, but because skeletal muscle cells are both highly active and numerous, together they produce a major share of total body heat. Skeletal muscle contractions therefore constitute one of the most important parts of the mechanism for maintaining homeostasis of temperature.
FUNCTION OF SKELETAL MUSCLE TISSUE
Skeletal muscle cells have several characteristics that permit them to function as they do. One such characteristic is the ability to be stimulated, often called excitability or irritability. Because skeletal muscle cells are excitable, they can respond to regulatory mechanisms such as nerve signals.
Contractility of muscle cells, the ability to contract or shorten, allows muscle tissue to pull on bones and thus produce body movement. Sometimes muscle fibers do work by steadily resisting a load without actually becoming shorter. In such a case, the muscle cell is still said to be contracting. The term contraction, when applied to muscles, is meant in a broad sense of pulling the ends together—regardless of whether the cell actually gets shorter.
Extensibility, the ability to extend or stretch, allows muscles to return to their resting length after having contracted. Muscles may also extend while still exerting force, as when lowering a heavy object in your hand.
All of these characteristics of muscle cells are related to the microscopic structure of skeletal muscle cells. In the following passages, we first discuss the basic structure of a muscle cell. We then explain how a muscle cell’s structural components allow it to perform its unique functions.
Overview of the Muscle Cell
Look at Figure 12-1. As you can see, a skeletal muscle is composed of bundles of skeletal muscle fibers that generally extend the entire length of the muscle. Muscle cells or myocytes are most frequently called “muscle fibers.” One reason they are often called fibers instead of cells is their long, thin, threadlike shape. They are 1 to 40 mm long but have a diameter of only 10 to 100 μm. The gastrocnemius muscle of the calf, for example, has approximately a million threadlike muscle fibers.
During muscle tissue development, individual precursor cells fuse together to form a new, combined structure with many nuclei—the mature muscle fiber. That is why muscle fibers don’t follow the general rule of one nucleus per cell: each fiber is made up of several cells that are combined into one. Some adult muscle fibers have one of these tiny precursor cells hugging their outer boundary. These satellite cells are stem cells that fuse with myocytes during strength training to make bigger muscle fibers. The satellite cells can also become active after a muscle injury to produce more muscle fibers. Scientists are now trying to understand this mechanism better in the hope of developing new therapies for replacing lost muscle tissues.
Skeletal muscle fibers have many of the same structural parts as other cells. Several of these parts, however, are referred to by different names in regard to muscle fibers. For example, sarcolemma is a name often used for the plasma membrane of a muscle fiber. Sarcoplasm is its cytoplasm.
Muscle fibers contain many more mitochondria than usually found in other types of cells (Figure 12-2), and as we have already learned, each fiber has several nuclei. Muscle cells contain networks of tubules and sacs known as the sarcoplasmic reticulum (SR)—the muscle fiber’s version of smooth endoplasmic reticulum. The function of the SR is to temporarily store calcium ions (Ca++). The membrane of the SR continually pumps Ca++ from the sarcoplasm and stores the ions within its sacs.
A structure unique to muscle cells is a system of transverse tubules, or T tubules. This name derives from the fact that these tubules extend transversely across the sarcoplasm, at a right angle to the long axis of the cell. As Figures 12-1, B, and 12-2 show, T tubules are formed by inward extensions of the sarcolemma. The chief function of T tubules is to allow electrical signals, or impulses, traveling along the sarcolemma to move deeper into the cell.
Notice in Figures 12-1, B, and 12-2 that a tubular sac of the SR butts up against each side of every T tubule in a muscle fiber. This triplet of tubules (a T tubule sandwiched between sacs of the SR) is called a triad. The triad is an important feature of the muscle cell because it allows an electrical impulse traveling along a T tubule to stimulate the membranes of adjacent sacs of the SR. Figure 12-3 shows how this works. At rest, calcium ion pumps in the SR membrane pump Ca++ into the terminal sacs of the SR. However, when an electrical impulse travels along the sarcolemma and down the T tubule, gated Ca++ channels in the SR open in response to the voltage fluctuation. This floods the sarcoplasm with calcium ions—an important step in initiating muscle contraction, as we shall see.
Additional structures not found in other cells are present in skeletal muscle fibers. For instance, the cytoskeleton of the muscle fiber is quite unique. Myofibrils are bundles of very fine cytoskeletal filaments that extend lengthwise along skeletal muscle fiber and almost fill the sarcoplasm. A typical muscle fiber may have somewhere in the neighborhood of a thousand or more myofibrils tightly packed inside it.
Myofibrils, in turn, are made up of still finer fibers called myofilaments. Notice in Figure 12-1, D, that there are two types of myofilaments in the myofibril: thick filaments and thin filaments. You can see at a glance that some of the filaments are much thicker than others. Although they appear in contrasting colors in the diagram, they are actually colorless.
A sarcomere is a segment of the myofibril between two successive Z disks (Z lines) (Box 12-1). Find the label sarcomere in Figure 12-1, C. You will note that each myofibril consists of a lineup of many sarcomeres. Each sarcomere functions as a contractile unit. The A bands of sarcomeres appear as relatively wide, dark stripes (cross striae) under the microscope, and they alternate with narrower, lighter-colored stripes formed by the I bands (see the figure in Box 12-1). Because of its cross striations (or striae), skeletal muscle is sometimes called striated muscle. Electron microscopy of skeletal muscle (Figure 12-4) reveals details that help us understand concepts of its structure and function.
Myofilaments
Each muscle fiber contains a thousand or more parallel myofibrils that are only about 1 μm thick. Lying side by side in each myofibril are approximately 15,000 sarcomeres, each made up of hundreds of thick and thin filaments. The molecular structure of these myofilaments reveals the mechanism of how muscle fibers contract and do so powerfully. It is wise, therefore, to take a moment to first study the structure of myofilaments before discussing the detailed mechanism of muscle contraction.
First of all, four different kinds of protein molecules make up myofilaments: myosin, actin, tropomyosin, and troponin. The thin filaments are made of a combination of three proteins: actin, tropomyosin, and troponin. Figure 12-5, A, shows that globular actin molecules (g-actin) are strung together like beads to form two fibrous strands (f-actin) that twist around each other to form the bulk of each thin filament. Actin and myosin molecules have a chemical attraction for one another, but at rest, the active sites on the actin molecules are covered by long tropomyosin molecules. The tropomyosin molecules seem to be held in this blocking position by troponin molecules spaced at intervals along the thin filament (see Figure 12-5, A).
As Figure 12-5, B, shows, the thick filaments are made almost entirely of myosin molecules. Notice that the myosin molecules are shaped like two golf clubs twisted together. Their long shafts bundle together to form a thick filament and their doubled “heads” stick out from the bundle. The myosin heads are chemically attracted to the actin molecules of the nearby thin filaments, so they angle toward the thin filaments. When they bridge the gap between adjacent myofilaments, the myosin heads are usually called cross bridges. Recall from Figure 3-17 (p. 82) that myosin acts as a molecular motor. The myosin in thick filaments can actively pull on actin when adenosine triphosphate (ATP) is available.
Within a myofibril, the thick and thin filaments alternate, as shown in Figure 12-1, D. They are also very close to one another, as you can see in the cross section in Figure 12-5, C, and Figure 12-6. This arrangement is crucial for contraction. Another fact important for contraction is that the thin filaments attach to both Z disks (Z lines) of a sarcomere and that they extend in from the Z disks partway toward the center of the sarcomere. When the muscle fiber is relaxed, the thin filaments terminate at the outer edges of the H bands. In contrast, the thick myosin filaments do not attach directly to the Z disks, and they extend only to the length of the A bands of the sarcomeres.
Mechanism of Contraction
To accomplish the powerful shortening, or contraction, of a muscle fiber, several processes must be coordinated in a stepwise fashion. These steps are summarized in the following sections and in Box 12-2.
EXCITATION OF THE SARCOLEMMA
Under normal circumstances, a skeletal muscle fiber remains “at rest” until it is stimulated by a signal from a special type of nerve cell called a motor neuron. As Figure 12-7 shows, motor neurons connect to the sarcolemma of a muscle fiber at a folded motor endplate to form a junction called a neuromuscular junction.
A neuromuscular junction (NMJ) is a type of connection called a synapse and is characterized by a narrow gap, or synaptic cleft, across which neurotransmitter molecules transmit signals. When nerve impulses reach the end of a motor neuron fiber, small vesicles release a neurotransmitter, acetylcholine (ACh), into the synaptic cleft. Diffusing swiftly across this microscopic gap, acetylcholine molecules contact the sarcolemma of the adjacent muscle fiber. There they stimulate acetylcholine receptors and thereby initiate an electrical impulse in the sarcolemma. The process of synaptic transmission and induction of an impulse—a process often called excitation—is discussed in detail in Chapter 13.
CONTRACTION
The impulse, a temporary electrical voltage imbalance, is conducted over the muscle fiber’s sarcolemma and inward along the T tubules (Figure 12-8). The impulse in the T tubules triggers the release of a flood of calcium ions from the adjacent sacs of the SR (see Figures 12-3 and 12-8).
In the sarcoplasm, the calcium ions combine with troponin molecules in the thin filaments of the myofibrils (Figure 12-9). Recall that troponin normally holds tropomyosin strands in a position that blocks the chemically active sites of actin. When calcium binds to troponin, however, the tropomyosin shifts to expose active binding sites on the actin molecules (Figure 12-10). Once the active sites are exposed, energized myosin heads of the thick filaments bind to actin molecules in the nearby thin filaments. The myosin head temporarily forms a cross bridge between the thick and thin filaments (Figure 12-11). After forming cross bridges, the myosin heads bend with great force, literally pulling the thin filaments past them. Each head then releases itself, binds to the next active site, and pulls again. Figure 12-12 shows how sliding of the thin filaments toward the center of each sarcomere quickly shortens the entire myofibril—and thus the entire muscle fiber.
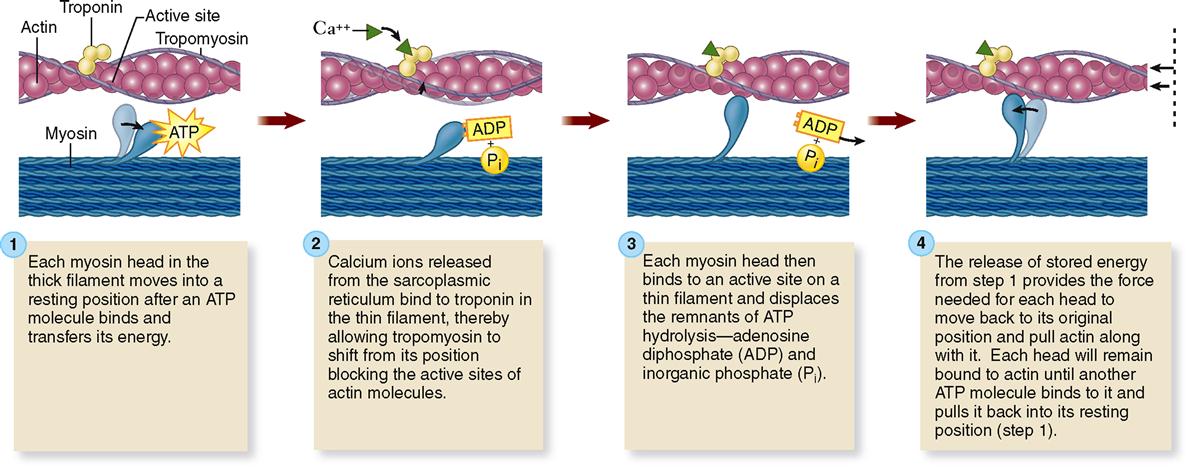
This model of muscle contraction has been called the sliding-filament model. Perhaps a better name might be ratcheting-filament model because the myosin heads actively ratchet the thin filaments toward the center of the sarcomere with great force.
Muscle fibers usually contract to about 80% of their starting length—rarely to 60% or 70%. Some muscle fibers contract hardly at all because they are pulling on an unmoving load. Even so, such muscle fibers are still said to be “contracting” in a broad sense. Their sarcomeres are still working hard to pull the ends toward one another, as though they are at a steady “draw” in a game of tug-of-war (Figure 12-13). Because the actin-myosin bond is not permanent, the sarcomere cannot “lock up” once it has shortened to hold its position passively. Thus it takes energy to actively maintain a shortened position—by continually repeating the actin-myosin reaction as long as necessary.
