Skeletal Tissues
FUNCTIONS OF BONE
A good place to begin a study of the skeletal system is with the overall functions of its organs, the bones and ligaments. Ligaments are fibrous bands that help hold the various bones together into an organized skeleton. Bones are rigid, mineralized structures that help perform five major roles in the body. Each is important for maintaining overall stability of the body’s internal environment, or homeostasis.
3. Movement. Bones along with their joints constitute levers. Muscles are anchored firmly to bones. As muscles contract and shorten, they pull on bones, thereby producing movement at a joint. This process is discussed further in Chapter 10.
4. Mineral storage. Bones serve as the major reservoir for calcium, phosphorus, and certain other minerals. Homeostasis of the blood calcium concentration—essential for healthy survival—depends largely on changes in the rate of calcium movement between blood and bones. If, for example, the blood calcium concentration increases above normal, calcium moves more rapidly out of blood into bones and more slowly in the opposite direction. The result? Blood calcium concentration decreases—usually to its homeostatic level. Hormonal control of blood calcium is discussed further in Chapter 19.
5. Hematopoiesis. Hematopoiesis, or blood cell formation, is a vital process carried on by red bone marrow, or myeloid tissue. Myeloid tissue, in the adult, is located primarily in the ends, or epiphyses, of certain long bones, in the flat bones of the skull, in the pelvis, and in the sternum and ribs. The process of hematopoiesis is discussed further in Chapter 20.
TYPES OF BONES
Structurally, a simple way to categorize the 206 or more bones of the skeleton is by shape. Usually they are divided into five categories: long bones, short bones, flat bones, irregular bones, and sesamoid bones. Figure 8-2 shows an example of each type. The size, shape, and appearance of bones vary according to the role of each bone in the skeleton. Some bones must bear great weight; others serve a protective function or serve as delicate support structures, such as for the fingers and toes.
Bones differ in size and shape and also in the amount and proportion of the two different types of bone tissue that compose them. Compact bone is dense and “solid” in appearance. Cancellous bone, on the other hand, is characterized by open space with a network of thin, branched crossbeams. Recall from Chapter 6 that cancellous bone is also called spongy bone or trabecular bone. Both compact and cancellous bone types are discussed when the microscopic structure of bone is described later in the chapter.
All five shape categories of bone discussed below have varying amounts of cancellous and compact bone in their structure.
Long bones are easily identified by their roughly cylindrical shape that is longer than it is wide. They also have enlarged and often uniquely shaped ends that articulate with other bones. The femur of the thigh and humerus of the arm are examples. Other examples include the radius, ulna, tibia, fibula, metacarpal bones, metatarsal bones, and phalanges.
Short bones are often described as cube- or box-shaped structures that are about as broad as they are long. Examples include each of the wrist (carpal) and ankle (tarsal) bones.
Flat bones are generally broad and thin with a flattened and often curved surface. Certain bones of the skull, the shoulder blades (scapulae), ribs, and breastbone (sternum) are typical flat bones.
Irregular bones are often clustered in groups and come in various sizes and shapes. The vertebral bones that form the spine and the facial bones of the skull are good examples.
Sesamoid bones, which are sometimes grouped with the irregular bones, often appear singly rather than in groups. The name comes from “sesame seed” because these bones often resemble sesame seeds in size and shape. The number of sesamoid bones in the body can be many or only a few—the number and size vary from one person to the next. Sesamoid bones usually develop in the tendons close to the joints. The patella (kneecap) is the largest sesamoid bone—one of the few that consistently appear in the human skeleton.
Parts of a Long Bone
A long bone consists of the following structures visible to the naked eye: diaphysis, epiphyses, articular cartilage, periosteum, medullary (marrow) cavity, and endosteum. Identify each of these structures in the tibia shown in Figure 8-3, A. The tibia is the longer, stronger, and more medially located of the two leg bones.
2. Epiphyses (eh-PIF-i-seez)—the proximal and distal ends of a long bone. Epiphyses have a bulbous shape that provides generous space near joints for muscle attachments and also gives stability to joints. Look at Figure 8-3 to note the innumerable small spaces in the bone of the epiphysis. They make this kind of bone look a little like a sponge—hence its name spongy, or cancellous, bone. A soft connective tissue called red marrow fills the spaces within this spongy bone. Early in development, epiphyses are separated from the diaphysis by a layer of cartilage, the epiphyseal plate. The cartilage layer is eventually replaced by bone, forming an epiphyseal line. The region between the epiphyses and diaphysis (in a mature bone) or the epiphyseal plate region (in a growing bone) is called the metaphysis (meh-TAF-i-sis).
Parts of Flat Bones and Other Bones
The structure of the flat bone is very similar to that of a long bone, but simpler. As you can see in Figure 8-4, a flat bone of the cranium has outer and inner walls made of compact bone. These hard walls are called the internal table and external table. Between is a region called the diploe, which is made up of cancellous bone. Other flat bones, such as the ribs and sternum, have a similar overall structure. Like long bones, flat bones are covered in a periosteum and the inner spaces are lined with endosteum.
Red marrow fills the spaces of the cancellous bone inside many flat bones. The sternum, which contains red marrow even in adulthood, is one example. To help in the diagnosis of leukemia and certain other diseases, a physician may decide to perform a needle puncture of one of these bones. In this type of diagnostic procedure, a needle is inserted through the skin and compact bone into the red marrow, and a small amount of the marrow is then aspirated and examined under the microscope for evidence of normal or abnormal blood cells. The procedure, called aspiration biopsy cytology (ABC), is discussed on p. 619.
Short bones, irregular bones, and sesamoid bones all have features similar to those of flat bones.
BONE TISSUE
Bone tissue, sometimes called osseous tissue, is perhaps the most distinctive form of connective tissue in the body. It is typical of other connective tissues in that it consists of cells, fibers, and extracellular material, or matrix. However, its extracellular components are hard and calcified. In bone the extracellular material, or matrix, predominates. It is much more abundant than the bone cells, and it contains many fibers of collagen (the body’s most abundant protein). The rigidity of bone enables it to serve supportive and protective functions.
As a tissue, bone is ideally suited to its functions. You can easily see the concept that structure and function are interrelated in bone tissue! It has a tensile strength nearly equal to that of cast iron but at less than one third the weight. Bone is organized so that its great strength and minimal weight result from the interrelationships of its structural components. The relationship of structure to function is seen all the way through the chemical, cellular, tissue, and organ levels of organization.
Composition of Bone Matrix
The extracellular matrix (ECM) of bone, or bone matrix, can be subdivided into two principal chemical components: inorganic salts and organic matrix. About two thirds of the matrix by dry weight analysis consists of inorganic salts and one third, organic material. You may find it helpful to review the section describing the unique characteristics of ECM in Chapter 6 on p. 134 and illustrated in Figure 6-1 on the same page.
INORGANIC SALTS
The calcified nature and thus the hardness of bone result from the deposition of rocklike crystals of calcium and phosphate. Chemists call them hydroxyapatite crystals. The process of forming these crystals within a softer tissue is called calcification. The tiny, needlelike hydroxyapatite crystals make up about 85% of the total inorganic matrix and are found oriented in the microscopic spaces between collagen fibers so that they can most effectively resist stress and mechanical deformation. In addition to hydroxyapatite and about 10% calcium carbonate, other mineral constituents such as magnesium, sodium, sulfate, and fluoride are also found in bone. Unfortunately, harmful elements can become incorporated into bone matrix as well and result in loss of normal function or active disease. For example, radioactive elements such as radium, strontium-90, uranium, or plutonium can become concentrated in bone and continue to emit radiation, which can lead to various types of cancer and other serious disease.
ORGANIC MATRIX
The organic matrix of bone and other connective tissues is a composite of collagenous fibers and a mixture of protein and polysaccharides called ground substance. Connective tissue cells secrete the gel-like ground substance. The ground substance of bone provides support and adhesion between cellular and fibrous elements and also serves an active role in many cellular metabolic functions necessary for growth, repair, and remodeling.
Chondroitin sulfate and glucosamine are the names of chemical substances that many people recognize as components of over-the-counter dietary supplements. Taken alone or together they are widely thought to facilitate healing and reduce the “wear and tear” pain of osteoarthritis. However, research has yet to fully support that claim. Chondroitin sulfate and glucosamine are found naturally in the body and are important constituents of the ground substance in both bone and cartilage. Chemically, chondroitin sulfate is a large protein molecule that helps cartilage remain compressible and elastic and may slow its destruction. Glucosamine is an amino sugar important in cartilage formation, maintenance, and repair. Go back to Figure 6-1 (p. 134) to see how such molecules are incorporated into the ECM of a tissue.
Components of the organic matrix help cartilage maintain a smooth surface and springy consistency. They add to overall strength and also give bone some degree of plasticlike resilience so that applied stress—within reasonable limits—does not result in frequent crush or fracture injuries.
MICROSCOPIC STRUCTURE OF BONE
The basic structural components and cell types of bone were described briefly in Chapter 6. In the paragraphs that follow, additional information about bone structure and cell types will serve as a basis for learning the functional characteristics of this important tissue. Understanding how a bone forms and grows, how it repairs itself after injury, and how it interacts with other tissues and organs in maintaining various important homeostatic mechanisms is based on a knowledge of its basic structure—a structure as unique as its chemical composition.
Compact Bone
Compact bone constitutes about 80% of the total bone mass in the adult human body. It contains many cylinder-shaped structural units called osteons (AHS-tee-onz), or Haversian systems. Note in Figure 8-5 that each osteon surrounds a central canal that runs lengthwise through the bone. Living bone cells in these units are literally cemented together to constitute the structural framework of compact bone. The unique structure of the osteon permits delivery of nutrients and removal of waste products from metabolically active, but imprisoned, bone cells.
Several simple structures make up each osteon: lamellae, lacunae, canaliculi, and a central canal. As you read the following descriptions, identify each structure in Figure 8-5.
Parallel central canals are connected to each other by transverse canals (Volkmann canals). These communicating canals contain nerves and vessels that carry blood and lymph from the exterior surface of the bone to the osteons.
Cancellous Bone
Cancellous, or spongy, bone constitutes about 20% of the total bone mass and differs in microscopic structure from compact bone. As you recall, the structural unit of compact bone is the highly organized osteon. There are no osteons in cancellous bone. Instead, it consists of crisscrossing bony branches called trabeculae. Bone cells are found within the trabeculae. Nutrients are delivered to the cells and waste products are removed by diffusion through tiny canaliculi that extend to the surface of the very thin bony branches. Cancellous bone is often called trabecular bone.
Note that the cancellous bone shown in Figure 8-6 lies between two layers of compact bone, much like the filling in a sandwich. Recall that the middle layer of spongy bone is called the diploe. This layered organization is typical of flat bones such as those found in the skull. Cancellous bone is also found inside short and irregular bones, as well as inside the epi-physes (see Figure 8-3) and lining the medullary cavities of long bones (see Figure 8-5, A).
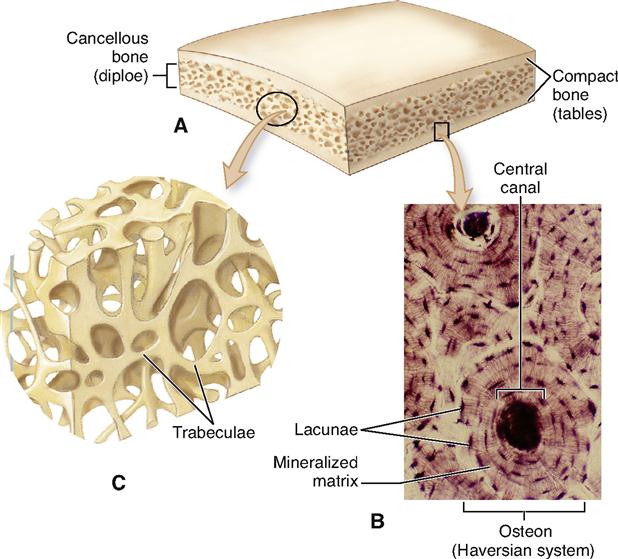
The placement of trabeculae in spongy bone is not as random and unorganized as it might first appear. They are fractal in nature, meaning that they appear random, but there is really an underlying, complex organization to them. The bony branches are actually arranged along lines of stress, and their size and orientation will therefore differ between individual bones according to the nature and magnitude of the applied load (Figure 8-7). This feature greatly enhances a bone’s strength and is yet another example of the relationship between structure and function.
