Pharmacology of the Adrenal Cortex
The major physiological and pharmacological effects of adrenocorticotropic hormone (ACTH, corticotropin) result from its action to increase the circulating levels of adrenocortical steroids. Synthetic derivatives of ACTH are used principally in the diagnostic assessment of adrenocortical function. Because corticosteroids mimic the therapeutic effects of ACTH, synthetic steroids generally are used therapeutically instead of ACTH.
Corticosteroids and their biologically active synthetic derivatives differ in their metabolic (glucocorticoid) and electrolyte-regulating (mineralocorticoid) activities. These agents are employed at physiological doses for replacement therapy when endogenous production is impaired. Glucocorticoids potently suppress inflammation, and their use in inflammatory and autoimmune diseases makes them among the most frequently prescribed classes of drugs. Because glucocorticoids exert effects on almost every organ system, the clinical use of and withdrawal from corticosteroids are complicated by a number of serious side effects. Therefore, the decision to institute therapy with systemic corticosteroids always requires careful consideration of the relative risks and benefits in each patient.
ACTH
Human ACTH, a peptide of 39 amino acids, is synthesized as part of a larger precursor protein, proopiomelanocortin (POMC), and is liberated from the precursor through proteolytic cleavage at dibasic residues by the serine endoprotease, prohormone convertase 1 (also known as prohormone convertase 3) (Figure 42–1). Other biologically important peptides, including endorphins, lipotropins, and the melanocyte-stimulating hormones (MSHs), also are produced by proteolytic processing of the same POMC precursor (see Chapter 18).
Figure 42–1 Processing of proopiomelanocortin POMC to ACTH. Proopiomelanocortin (POMC) is converted to adrenocorticotropic hormone (ACTH) and other peptides in the anterior pituitary. The boxes within the ACTH structure indicate regions important for steroidogenic activity (residues 6-10) and binding to the ACTH receptor (15-18). α-Melanocyte-stimulating hormone also derives from the POMC precursor and contains the first 13 residues of ACTH. LPH, lipotropin; MSH, melanocyte-stimulating hormone.
The actions of ACTH and the other melanocortins liberated from POMC are mediated by their specific interactions with 5 melanocortin receptor (MCR) subtypes (MC1R-MC5R) comprising a subfamily of G protein-coupled receptors (GPCRs). The well-known effects of MSH on pigmentation result from interactions with the MC1R on melanocytes. ACTH, which is identical to α-MSH in its first 13 amino acids, exerts its effects on the adrenal cortex through the MC2R. The affinity of ACTH for the MC1R is much lower than for the MC2R; however, under pathological conditions in which ACTH levels are persistently elevated, such as primary adrenal insufficiency, ACTH also can signal through the MC1R and cause hyperpigmentation. β-MSH and possibly other melanocortins, acting via the MC4R and MC3R in the hypothalamus, play a role in regulating appetite and body weight. The role of MC5R is less well defined.
ACTIONS ON THE ADRENAL CORTEX. Acting via MC2R, ACTH stimulates the adrenal cortex to secrete glucocorticoids, mineralocorticoids, and the androgen precursor dehydroepiandrosterone (DHEA). The adrenal cortex histologically and functionally can be separated into 3 zones (Figure 42–2) that produce different steroid products under different regulatory influences:
Figure 42–2 The three anatomically and functionally distinct compartments of the adrenal cortex. The major functional compartments of the adrenal cortex are shown, along with the steroidogenic enzymes that determine the unique profiles of corticosteroid products. Also shown are the predominant physiological regulators of steroid production: angiotensin II (AngII) and K+ for the zona glomerulosa and ACTH for the zona fasciculata. The physiological regulator(s) of dehydroepiandrosterone (DHEA) production by the zona reticularis are not known, although ACTH acutely increases DHEA biosynthesis.
• The outer zona glomerulosa secretes the mineralocorticoid aldosterone.
• The middle zona fasciculata secretes the glucocorticoid cortisol.
• The inner zona reticularis secretes DHEA and its sulfated derivative DHEAS (plasma concentration 1000× that of DHEA). DHEA sulfatase converts DHEAS to DHEA in the periphery.
Cells of the outer zone have receptors for angiotensin II (AngII) and express aldosterone synthase (CYP11B2), an enzyme that catalyzes the terminal reactions in mineralocorticoid biosynthesis. Although ACTH acutely stimulates mineralocorticoid production by the zona glomerulosa, this zone is regulated predominantly by AngII and extracellular K+ (see Chapter 25) and does not undergo atrophy in the absence of ongoing stimulation by the pituitary gland. With persistently elevated ACTH, mineralocorticoid levels initially increase and then return to normal (a phenomenon termed ACTH escape). Cells of the zona fasciculata have fewer receptors for AngII and express steroid 17α-hydroxylase (CYP17) and 11β-hydroxylase (CYP11B1) enzymes that catalyze the production of glucocorticoids. In the zona reticularis, CYP17 carries out an additional C17-20 lyase reaction that converts C21 corticosteroids to C19 androgen precursors.
In the absence of the anterior pituitary and ACTH stimulation, the inner zones of the cortex atrophy, and the production of glucocorticoids and adrenal androgens is markedly impaired. Persistently elevated levels of ACTH, due either to repeated administration of large doses of ACTH or to excessive endogenous production, induce hypertrophy and hyperplasia of the inner zones of the adrenal cortex, with overproduction of cortisol and adrenal androgens. Adrenal hyperplasia is most marked in congenital disorders of steroidogenesis, in which ACTH levels are continuously elevated as a secondary response to impaired cortisol biosynthesis.
MECHANISM OF ACTION. ACTH stimulates the synthesis and release of adrenocortical hormones by increasing de novo biosynthesis. ACTH, binding to MC2R, activates the Gs-adenylyl cyclase-cyclic AMP-PKA pathway. Cyclic AMP is the second messenger for most effects of ACTH on steroidogenesis. Temporally, the response of adrenocortical cells to ACTH has 2 phases. The acute phase, which occurs within seconds to minutes, largely reflects increased supply of cholesterol substrate to the steroidogenic enzymes. The chronic phase, which occurs over hours to days, results largely from increased transcription of the steroidogenic enzymes. Pathways of adrenal steroid biosynthesis and the structures of the major steroid intermediates and products of the human adrenal cortex are shown in Figure 42–3. The rate-limiting step in steroid hormone production is the conversion of cholesterol to pregnenolone, a reaction catalyzed by CYP11A1, the cholesterol side-chain cleavage enzyme. Most of the enzymes required for steroid hormone biosynthesis, including CYP11A1, are members of the cytochrome P450 superfamily (see Chapter 6).
Figure 42–3 Pathways of corticosteroid biosynthesis. The steroidogenic pathways used in the biosynthesis of the corticosteroids are shown, along with the structures of the intermediates and products. The pathways unique to the zona glomerulosa are shown in the orange box; those that occur in the inner zona fasciculata and zona reticularis are shown in the gray box. The zona reticularis does not express 3β-HSD and thus preferentially synthesizes DHEA; see Figure 42–2. CYP11A1, cholesterol side-chain cleavage enzyme; 3β-HSD, 3β-hydroxysteroid dehydrogenase; CYP17, steroid 17α-hydroxylase; CYP21, steroid 21-hydroxylase; CYP11B2, aldosterone synthase; CYP11B1, steroid 11β-hydroxylase.
REGULATION OF ACTH SECRETION
HYPOTHALAMIC-PITUITARY-ADRENAL AXIS. The rate of glucocorticoid secretion is determined by fluctuations in the release of ACTH by the pituitary corticotropes. These corticotropes are regulated by corticotropin-releasing hormone (CRH) and arginine vasopressin (AVP), peptide hormones released by specialized neurons of the endocrine hypothalamus. This hypothalamic-pituitary-adrenal (HPA) axis forms an integrated system that maintains appropriate levels of glucocorticoids (Figure 42–4). The 3 characteristic modes of regulation of the HPA axis are diurnal rhythm in basal steroidogenesis, negative feedback regulation by adrenal corticosteroids, and marked increases in steroidogenesis in response to stress.
Figure 42–4 The hypothalamic-pituitary-adrenal (HPA) axis and the immune inflammatory network. Also shown are inputs from higher neuronal centers that regulate CRH secretion. + indicates a positive regulator, – indicates a negative regulator, + and – indicates a mixed effect, as for NE (norepinephrine). In addition, arginine vasopressin stimulates release of ACTH from corticotropes.
The diurnal rhythm is entrained by higher neuronal centers in response to sleep-wake cycles, such that levels of ACTH peak in the early morning hours, causing the circulating glucocorticoid levels to peak at ~8 A.M. Negative feedback regulation occurs at multiple levels of the HPA axis and is the major mechanism that maintains circulating glucocorticoid levels in the appropriate range. Stress can override the normal negative feedback control mechanisms, leading to marked increases in plasma concentrations of glucocorticoids.
ARGININE VASOPRESSIN. AVP also acts as a secretagogue for corticotropes, significantly potentiating the effects of CRH. AVP is produced in the paraventricular nucleus and secreted into the pituitary plexus from the median eminence. AVP binds to V1b receptor and activates the Gq-PLC-IP3-Ca2+ pathway to enhance the release of ACTH. In contrast to CRH, AVP does not increase ACTH synthesis.
NEGATIVE FEEDBACK OF GLUCOCORTICOIDS. Glucocorticoids inhibit ACTH secretion via direct and indirect actions on CRH neurons to decrease CRH mRNA levels and CRH release and via direct effects on corticotropes. The indirect inhibitory effects on CRH neurons appear to be mediated by specific corticosteroid receptors in the hippocampus. At lower cortisol levels, the mineralocorticoid receptor (MR), which has a higher affinity for glucocorticoids than classical glucocorticoid receptors (GRs), is the major receptor species occupied. As glucocorticoid concentrations rise and saturate the MR, the GR becomes increasingly occupied. Both the MR and GR apparently control the basal activity of the HPA axis, whereas feedback inhibition by glucocorticoids predominantly involves the GR. In the pituitary, glucocorticoids act through the GR to inhibit the release of ACTH from corticotropes and the expression of POMC. These effects are both rapid (occurring within seconds to minutes) and delayed (requiring hours and involving changes in gene transcription mediated through the GR).
THE STRESS RESPONSE. Stress overcomes negative feedback regulation of the HPA axis, leading to a marked rise in corticosteroid production. Examples of stress signals include injury, hemorrhage, severe infection, major surgery, hypoglycemia, cold, pain, and fear. Although the precise mechanisms that underlie this stress response and the essential actions played by corticosteroids are not fully defined, increased corticosteroid secretion is vital to maintain homeostasis in these stressful settings. As discussed later, complex interactions between the HPA axis and the immune system may be a fundamental physiological component of this stress response.
ASSAYS FOR ACTH. Immunochemiluminescent assays that use 2 separate antibodies directed at distinct epitopes on the ACTH molecule now are widely available. These assays increase the ability to differentiate patients with primary hypoadrenalism due to intrinsic adrenal disease, who have high ACTH levels due to the loss of normal glucocorticoid feedback inhibition, from those with secondary forms of hypoadrenalism, due to low ACTH levels resulting from hypothalamic or pituitary disorders. The immunochemiluminescent ACTH assays also are useful in differentiating between ACTH-dependent and ACTH-independent forms of hypercorticism: High ACTH levels are seen when the hypercorticism results from pituitary adenomas (e.g., Cushing disease) or nonpituitary tumors that secrete ACTH (e.g., the syndrome of ectopic ACTH), whereas low ACTH levels are seen in patients with excessive glucocorticoid production due to primary adrenal disorders. One problem with the immunoassays for ACTH is that their specificity for intact ACTH can lead to falsely low values in patients with ectopic ACTH secretion; these tumors can secrete aberrantly processed forms of ACTH that have biological activity but do not react in the antibody assays.
THERAPEUTIC USES AND DIAGNOSTIC APPLICATIONS OF ACTH. ACTH has limited utility therapeutically. All proven therapeutic effects of ACTH can be achieved with appropriate doses of corticosteroids with a lower risk of side effects. Moreover, therapy with ACTH is less predictable and less convenient than therapy with corticosteroids. ACTH stimulates mineralocorticoid and adrenal androgen secretion and may therefore cause acute retention of salt and water, as well as virilization. Cosyntropin (CORTROSYN, SYNACTHEN), a synthetic peptide that corresponds to residues 1-24 of human ACTH, is used testing the integrity of the HPA axis. At the considerably supraphysiological dose of 250 μg, cosyntropin maximally stimulates adrenocortical steroidogenesis. An increase in the circulating cortisol to a level greater than 18-20 μg/dL indicates a normal response.
CRH Stimulation Test. Ovine CRH (corticorelin [ACTHREL]) and human CRH are available for diagnostic testing of the HPA axis, with the former used in the U.S. and the latter preferred in Europe. In patients with documented ACTH-dependent hypercorticism, CRH testing may help differentiate between a pituitary source (i.e., Cushing disease) and an ectopic source of ACTH.
ABSORPTION AND FATE TOXICITY. ACTH is readily absorbed from parenteral sites. The hormone rapidly disappears from the circulation after intravenous administration; in humans, the t1/2 in plasma is ~15 min, primarily due to rapid enzymatic hydrolysis. Aside from rare hypersensitivity reactions, the toxicity of ACTH is primarily attributable to the increased secretion of corticosteroids. Cosyntropin generally is less antigenic than native ACTH.
ADRENOCORTICAL STEROIDS
The adrenal cortex synthesizes 2 classes of steroids: the corticosteroids (glucocorticoids and mineralocorticoids; see Figure 42–3), which have 21 carbon atoms, and the androgens, which have 19 carbons (see Figures 41–1 and 41–3). The actions of corticosteroids historically were described as glucocorticoid (reflecting their carbohydrate metabolism–regulating activity) and mineralocorticoid (reflecting their electrolyte balance–regulating activity). In humans, cortisol (hydrocortisone) is the main glucocorticoid and aldosterone is the main mineralocorticoid (Table 42–1).
Table 42–1
Normal Daily Production Rates and Circulating Levels of the Predominant Corticosteroids
Although the adrenal cortex is an important source of androgen precursors in women, patients with adrenal insufficiency can be restored to normal life expectancy by replacement therapy with glucocorticoids and mineralocorticoids. Adrenal androgens are not essential for survival. The levels of DHEA and DHEA-S peak in the third decade of life and decline progressively thereafter. Moreover, patients with a number of chronic diseases have very low DHEA levels, leading some to propose that DHEA treatment might at least partly alleviate the loss of libido, the decline in cognitive function, the decreased sense of well-being, and other adverse physiological consequences of aging. However, studies on the benefits of addition of DHEA to the standard replacement regimen in women with adrenal insufficiency have been inconclusive.
PHYSIOLOGICAL FUNCTIONS AND PHARMACOLOGICAL EFFECTS
PHYSIOLOGICAL ACTIONS. Corticosteroids have numerous effects, which include alterations in carbohydrate, protein, and lipid metabolism; maintenance of fluid and electrolyte balance; and preservation of normal function of the cardiovascular system, the immune system, the kidney, skeletal muscle, the endocrine system, and the nervous system. In addition, corticosteroids endow the organism with the capacity to resist stressful and noxious stimuli and environmental changes. In the absence of the adrenal cortex, survival is made possible only by maintaining an optimal environment, including adequate and regular feeding, ingestion of relatively large amounts of NaCl, and maintenance of an appropriate environmental temperature; stresses such as infection, trauma, and extremes in temperature in this setting can be life threatening.
The actions of corticosteroids are related to those of other hormones. For example, in the absence of lipolytic hormones, cortisol has virtually no effect on the rate of lipolysis by adipocytes. Conversely, in the absence of glucocorticoids, EPI and NE have only minor effects on lipolysis. Administration of a small dose of glucocorticoid, however, markedly potentiates the lipolytic action of these catecholamines. Those effects of corticosteroids that involve concerted actions with other hormonal regulators are termed permissive and most likely reflect steroid-induced changes in protein synthesis that, in turn, modify tissue responsiveness to other hormones.
Corticosteroids are termed mineralocorticoids and glucocorticoids, according to their relative potencies in Na+ retention, effects on carbohydrate metabolism (i.e., hepatic deposition of glycogen and gluconeogenesis), and anti-inflammatory effects. In general, potencies of steroids as judged by their ability to sustain life in adrenalectomized animals closely parallel those determined for Na+ retention, whereas potencies based on effects on glucose metabolism closely parallel those for anti-inflammatory effects. The effects on Na+ retention and the carbohydrate/anti-inflammatory actions are not closely related and reflect selective actions at distinct receptors. As noted below (see structure-activity relationships and Table 42–3), some steroid derivatives provide relative selectivity for effects on Na+ retention or anti-inflammatory effects.
GENERAL MECHANISMS FOR CORTICOSTEROID EFFECTS. Corticosteroids bind to specific receptor proteins in target tissues to regulate the expression of corticosteroid-responsive genes, thereby changing the levels and array of proteins synthesized by the various target tissues (Figure 38–5). Most effects of corticosteroids are not immediate but become apparent after several hours; clinically, one generally sees a delay before beneficial effects of corticosteroid therapy become manifest. Although corticosteroids predominantly act by increasing gene transcription, there are examples in which glucocorticoids decrease gene transcription. In addition, corticosteroids may exert some of their immediate effects by nongenomic mechanisms.
Glucocorticoid Receptors (GRs). The receptors for corticosteroids are members of the nuclear receptor family of transcription factors. The GR resides predominantly in the cytoplasm in an inactive form complexed with other proteins. Steroid binding results in receptor activation and translocation to the nucleus (see Figure 38–5). Several GR isoforms result from alternative RNA splicing. Of these, GRα is the prototypical glucocorticoid-responsive isoform. A second major GR isoform, GRβ, is a truncated dominant negative variant that lacks 35 amino acids at the C-terminus and is unable to bind glucocorticoids or activate gene expression. Polymorphisms have been identified in the human GR that are associated with differences in GR function and have been linked to glucocorticoid insensitivity.
Regulation of Gene Expression by Glucocorticoids. After ligand binding, the GR dissociates from its associated proteins and translocates to the nucleus. There, it interacts with specific DNA sequences called glucocorticoid responsive elements (GREs), which provide specificity to the induction of gene transcription by glucocorticoids. Genes can be activated or inhibited by GR-GRE interactions. The mechanisms by which GR activates transcription are complex and not completely understood, but they involve the interaction of the GR with transcriptional coactivators and with proteins that make up the basal transcription apparatus. In a case of transcriptional inhibition by GR, GR inhibits transcription of POMC by a direct interaction with a GRE in the POMC promoter, thereby contributing to the negative feedback regulation of the HPA axis. Other genes negatively regulated by glucocorticoids include genes for cyclooxygenase-2 (COX-2), inducible NO synthase (NOS2), and inflammatory cytokines. Some inhibitory effects of glucocorticoids such as downregulation of expression of genes encoding a number of cytokines, collagenase, and stromelysin, have been linked to protein–protein interactions between the GR and other transcription factors (e.g., NF-κB and AP-1) rather than to negative effects of the GR at specific GREs. Such protein–protein interactions and their consequent negative effects on gene expression appear to contribute significantly to the anti-inflammatory and immunosuppressive effects of the glucocorticoids.
Regulation of Gene Expression by Mineralocorticoids. Like the GR, the mineralocorticoid receptor (MR) also is a ligand-activated transcription factor and binds to a very similar hormone-responsive element. The MR also associates with HSP90 and activates the transcription of discrete sets of genes within target tissues. The selective actions of GR and MR result from differences in their ability to inhibit AP-1–mediated gene activation and differential interactions with other transcription factors. In addition, the MR has restricted expression: It is expressed in epithelial tissues involved in electrolyte transport (i.e., the kidney, colon, salivary glands, and sweat glands) and in nonepithelial tissues (e.g., hippocampus, heart, vasculature and adipose tissue).
Aldosterone exerts its effects on Na+ and K+ homeostasis primarily via its actions on the principal cells of the distal renal tubules and collecting ducts, whereas the effects on H+
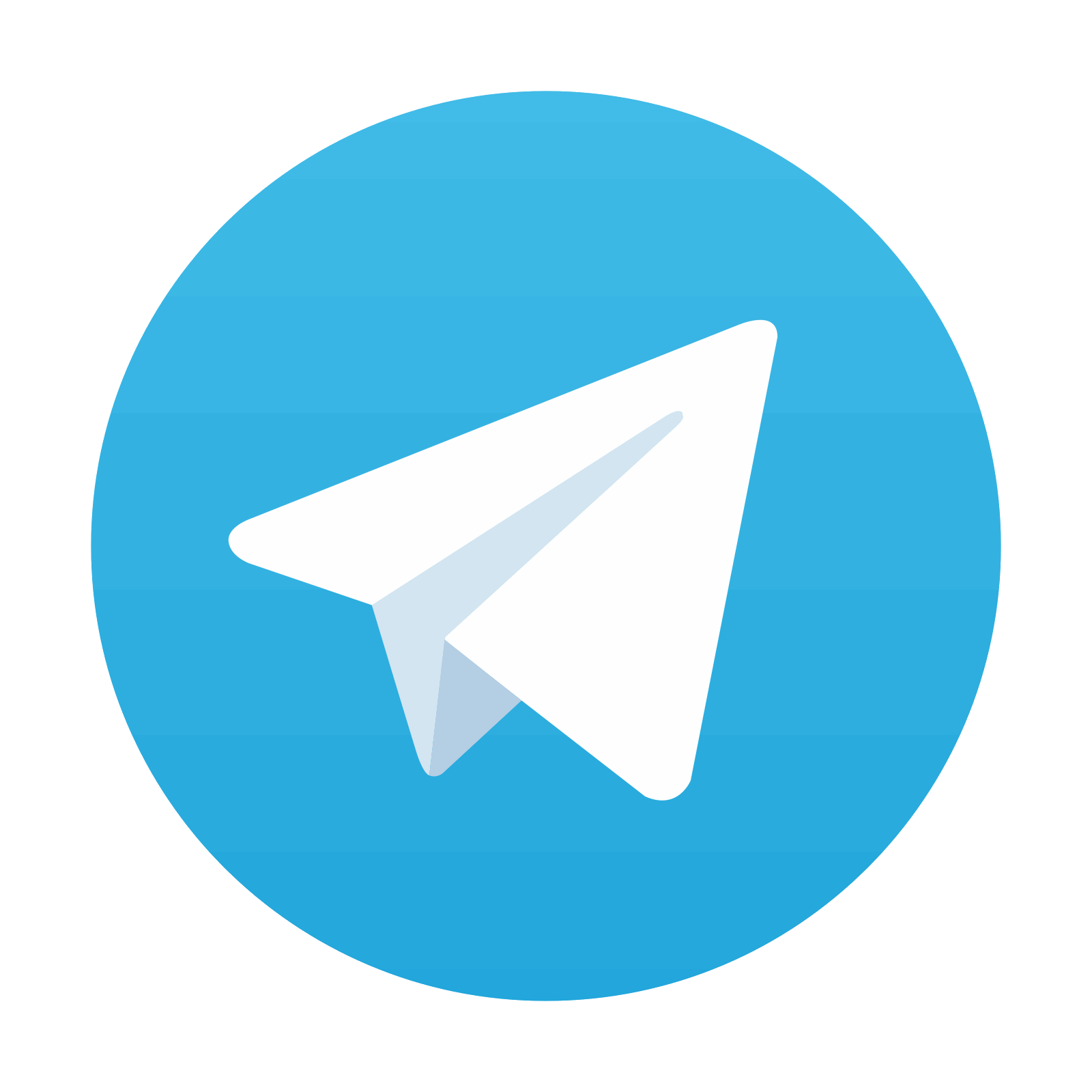
Stay updated, free articles. Join our Telegram channel

Full access? Get Clinical Tree
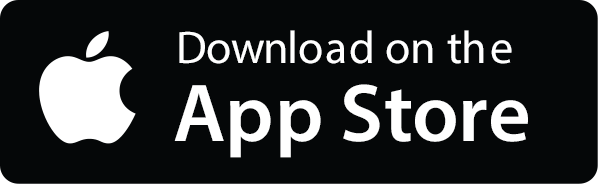
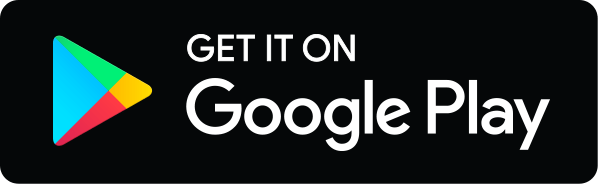