Chapter 8
Pharmacology of nitric oxide
8.1 Physiological significance of nitric oxide (NO)
- Powerful vasodilator—NO-releasing drugs are used in the treatment of cardiovascular disease
- Inflammatory mediator—inhibition of NO synthesis of interest as a therapeutic strategy in infection and chronic inflammation
- Neurotransmitter—signaling in the CNS and the autonomic nervous system
Notes: Nitric oxide differs in many ways from other signaling molecules, and because of its unusual nature was discovered relatively late. Its first physiological function to be discovered was the relaxation of blood vessels. While this function remains the focus of its application in pharmacotherapy, a range of other effects with at least potential applications in pharmacology were characterized subsequently.
The three listed physiological functions correspond to three different isoforms of nitric oxide synthase, the enzyme that produces NO (see slide 8.3).
8.2 Identification of “endothelium-derived relaxing factor” as nitric oxide
Vasorelaxation mediated by nitric oxide is controlled by the autonomic nervous system. The physiological experiments that uncovered the role of the endothelium, and subsequently of NO, in vasorelaxation are simple yet ingenious. In order to appreciate them, we need a little more anatomy.
8.2.1 Cholinergic and adrenergic nerve endings in a blood vessel wall
Notes: We had seen earlier that both the sympathetic and the parasympathetic nervous system innervate blood vessel walls and regulate their contraction and wall tension (slide 6.13.1). The adrenergic nerve endings of the sympathetic nerve fibers are found in the muscular layer of the vessel walls. In contrast, cholinergic nerve endings are found both in the muscular and the endothelial layers.
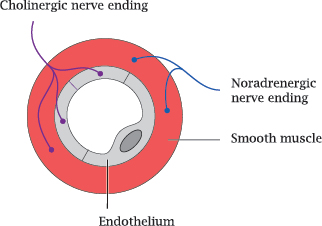
The wall tension of the blood vessel is sustained by the muscular layer, not the endothelium; therefore, the cholinergic innervation of the endothelium is surprising. As it turns out, however, it is needed for the vasorelaxation and vasodilation induced by parasympathetic nerve cells.
8.2.2 The endothelium is required for vascular relaxation in response to acetylcholine
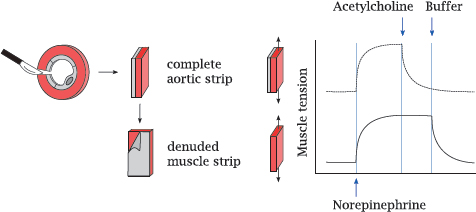
Notes: This slide illustrates the experiment that led to the discovery of nitric oxidemediated vasorelaxation.
Contraction and relaxation of the vascular smooth muscle cells in response to norepinephrine and acetylcholine were studied with aortic strips. In this technique, slices are cut from an animal’s aorta and then opened with a radial incision. The endothelium can be left in place or peeled away in order to study its effect on the muscular layer. The resulting intact or denuded aortic strips were mounted between two distending hooks to measure their contractile force.
Application of norepinephrine induced contraction in strips with or without attached endothelium, as would be expected from the innervation pattern of the sympathetic nerve fibers. In contrast, norepinephrine-induced contraction could be reversed by acetylcholine only if the strip retained the endothelium (top) but not when the endothelium had been peeled away.
Interestingly, relaxation of a denuded muscular strip could be restored if the endothelial side of an intact strip was strapped to it (not illustrated). This observation showed that, in response to cholinergic stimulation, the endothelium releases a diffusible substance that enters the muscular layer and induces its relaxation. This “endothelium-derived relaxing factor” was subsequently isolated and identified as NO.
8.3 The nitric oxide synthase reaction
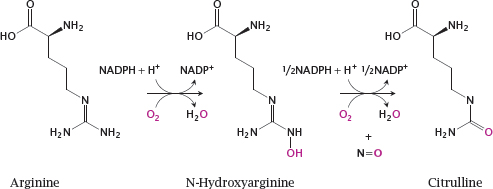
Notes: Nitric oxide is synthesized intracellularly by nitric oxide synthase (NOS). This reaction is rather complex and involves two successive monooxygenase steps. In the first step, arginine is converted to N-hydroxyarginine (NOHA), which is cleaved in the second step to NO and citrulline.
NOS occurs in several variations. Endothelial NOS (eNOS) and neuronal NOS (nNOS) are found in the cell types indicated by their names. Inducible NOS (iNOS) is found mainly in inflammatory cells. All these enzymes are homologous and perform the same reaction, but they differ in their regulatory properties.
8.3.1 Activation of NOS by calcium and calmodulin
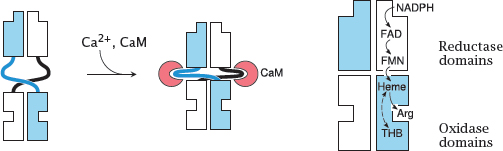
Notes: Nitric oxide synthase exists as a dimer. Each subunit contains a reductase domain and an oxidase domain. The reductase domain accepts electrons from NADPH and hands them over to the oxidase domain of the other subunit.
The reductase and oxidase domains are loosely connected by flexible hinges that, when extended, prevent the flow of electrons between them. The two domains are brought into close, productive interaction when calmodulin (CaM) binds to the hinges and changes their conformations. In the case of eNOS and nNOS, CaM binding only happens if the cytosolic level of Ca++ is elevated, which occurs only transiently through action potentials or through activation of GPCRs (for example the muscarinic ones in endothelial cells).
In contrast, inducible NOS binds calmodulin avidly and therefore is active even at the low resting level of Ca++. Its activity is regulated not by short-term calcium signals but instead by transcriptional induction.
8.4 NO activates soluble guanylate cyclase (sGC)
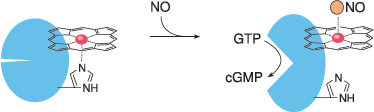
Notes: Between the last slide and this one, we have skipped an important step, namely the diffusion of NO from the cell of origin, for example an endothelial cell, to another one, such as a vascular smooth muscle cell. Like other small gas molecules, nitric oxide passes through cell membranes with ease, which enables it to directly and rapidly interact with intracellular targets.
Within its target cell, NO binds to the heme group of soluble guanylate cyclase (sGC), dislodging a strategic histidine residue. This causes a conformational change that activates the enzyme, which then begins to make cyclic GMP (cGMP). The production of cGMP as a second messenger is the most important signal downstream of NO.
8.4.1 Signaling effects of cGMP
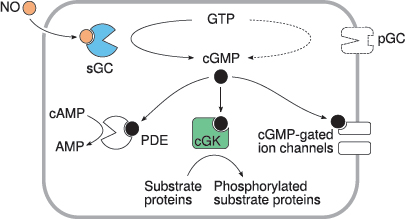
Notes: Like cAMP, cGMP targets multiple effector molecules inside the cell. Activation of cGMP-dependent protein kinases (cGK) is the most important single mechanism. Phosphodiesterase 5 (PDE) is activated, too, reducing the levels of both cAMP and cGMP. Actuation of cyclic nucleotide-gated cation channels affects the membrane potential and the cellular calcium level.
8.4.2 NO-induced relaxation of smooth muscle cells is mediated by cGK
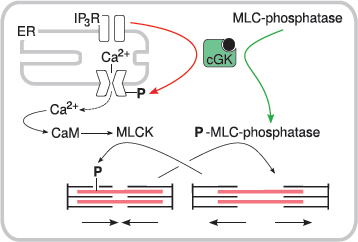
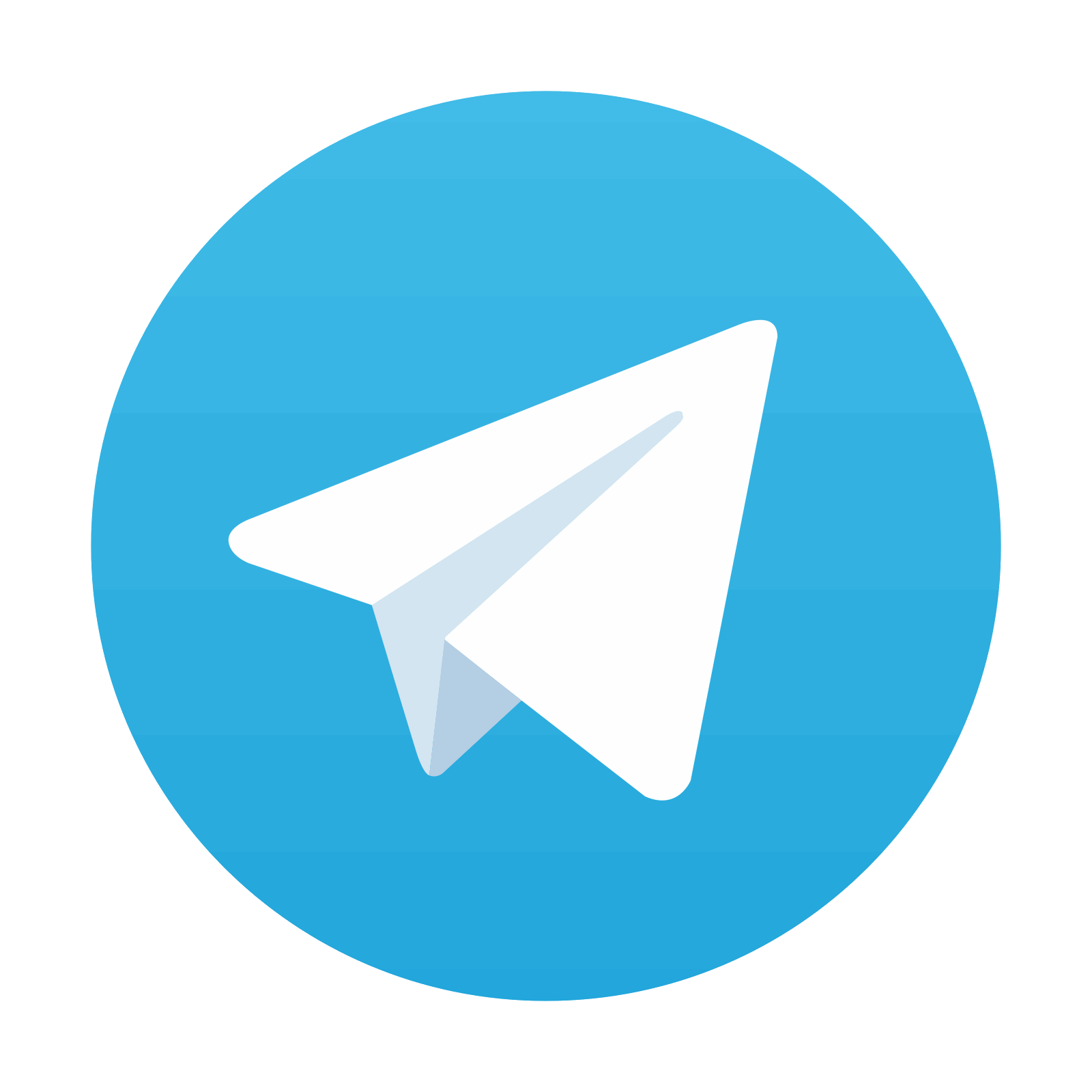