INTRODUCTION
Even the most promising of pharmacologic therapies will fail in clinical trials if the drug is unable to reach its target organ at a concentration sufficient to have a therapeutic effect. Many of the characteristics that render the human body resistant to harm by foreign invaders and toxic substances also limit the ability of modern drugs to combat pathologic processes within a patient. An appreciation of the many factors that affect a drug’s ability to act within a patient and the dynamic nature of these factors over time is vitally important to the clinical practice of medicine.
All drugs must meet certain minimal requirements to achieve clinical effectiveness. A successful drug must be able to cross the physiologic barriers that limit the access of foreign substances to the body. Drug absorption may occur by a number of mechanisms that are designed either to exploit or to breach these barriers. After absorption, the drug uses distribution systems within the body, such as the blood and lymphatic vessels, to reach its target organ in an appropriate concentration. The drug’s ability to act on its target is also limited by several processes within the patient. These processes fall broadly into two categories: metabolism, in which the body typically inactivates the drug through enzymatic degradation (primarily in the liver), and excretion, in which the drug is eliminated from the body (primarily by the kidneys and liver, and in the feces). This chapter presents a broad overview of the pharmacokinetic processes of absorption, distribution, metabolism, and excretion (often abbreviated as ADME; Fig. 3-1), with a conceptual emphasis on basic principles that, when applied to an unfamiliar situation, should enable the student or physician to understand the pharmacokinetic basis of drug therapy.
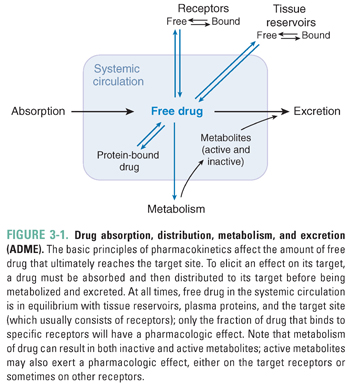
Mr. W is a 66-year-old technology consultant who makes frequent trips as part of his job in the telecommunications industry. His only medical problem is chronic atrial fibrillation, and his only chronic medication is warfarin. On the last night of a consulting trip abroad, he attends a large dinner featuring kebabs and other foods he does not often eat. The next day, he develops profuse, watery, foul-smelling diarrhea. A physician makes a diagnosis of traveler’s diarrhea and prescribes a 7-day course of trimethoprim-sulfamethoxazole.
Mr. W feels entirely well 2 days into the course of antibiotics, and 4 days later (while still taking his antibiotics), he meets with some clients at another lavish dinner. Mr. W and his guests become intoxicated at the dinner, and Mr. W stumbles and falls on the curb as he is leaving the restaurant. The next day, Mr. W has a markedly swollen right knee that requires evaluation in a local emergency department. Physical examination and imaging studies are consistent with a moderate-sized hemarthrosis of the right knee. Laboratory studies show a markedly elevated international normalized ratio (INR), which is a standardized measure of prothrombin time and, in this clinical setting, a surrogate marker for plasma warfarin level. The emergency physician advises Mr. W that his warfarin level is in the supratherapeutic (toxic) range and that this effect is likely due to adverse drug–drug interactions involving his warfarin, his antibiotics, and his recent alcohol intoxication.
Questions
1. How does a patient with well-established therapeutic levels of a chronic medication suddenly develop clinical manifestations of drug toxicity?
2. Could this situation have been avoided? If so, how?
A drug must overcome physical, chemical, and biological barriers to reach its molecular and cellular sites of action. The epithelial lining of the gastrointestinal tract and other mucous membranes is one sort of barrier; additional barriers are encountered after the drug enters the blood and lymphatics. Most drugs must distribute from the blood into local tissues, a process that may be impeded by structures such as the blood–brain barrier. Typically, drugs leave the intravascular compartment at the level of the postcapillary venules, where there are gaps between the endothelial cells through which the drug can pass. Drug distribution occurs mainly through passive diffusion, the rate of which is affected by local ionic and cellular conditions. This section describes the major physical, chemical, and biological barriers to drug transport in the body and the properties of drugs that affect their ability to overcome these barriers.
All human cells are limited by a lipid bilayer membrane. The membrane lipids consist mainly of phospholipids, sterols (especially cholesterol), and glycolipids. The amphiphilic nature of the membrane lipids and the aqueous intracellular and extracellular environments cause the membrane to assume a structure with a hydrophobic core and two hydrophilic surfaces. In addition to lipid components, biological membranes contain proteins that may span the membrane (transmembrane proteins) or be exposed only at the extracellular or intracellular membrane surface. The membrane’s semipermeable lipid bilayer structure provides a barrier to the transport of molecules and has important implications for drug therapy.
The hydrophobic core of a biological membrane presents the major barrier to drug transport. Small nonpolar molecules, such as steroid hormones, are able to diffuse easily through membranes. However, passive diffusion is ineffective for the transport of many large polar molecules and drugs. Some transmembrane proteins in the human solute carrier (SLC) superfamily—which includes 52 families of proteins such as organic anion transporters, organic cation transporters, peptide transporters, and nucleoside transporters—allow transport of polar drugs and molecules across the membrane. Transmembrane carrier proteins may be specific for a drug and related endogenous molecules; upon binding of the drug to the extracellular surface of the protein, the protein undergoes a conformational change that may be energy-independent (facilitated diffusion) or require energy (active transport). This conformational change allows the bound drug access to the interior of the cell, where the drug molecule is released from the protein. Alternatively, some drugs bind to specific cell surface receptors and trigger endocytosis, a process in which the cell membrane involutes around the molecule to form a vesicle from which the drug is subsequently released into the cell interior.
In the absence of other factors, a drug will enter a cell until the intracellular and extracellular concentrations of the drug are equal. The rate of diffusion depends on the concentration gradient of the drug across the membrane and on the thickness, area, and permeability of the membrane. Fick’s law of diffusion states that the net drug flux across the membrane is:

where C1 and C2 are the intracellular and extracellular concentrations of the drug, respectively. This definition applies to an ideal situation where there is an absence of complicating factors such as ionic, pH, and charge gradients across the membrane. In vivo, however, these additional factors affect the ability of a drug to enter cells. For example, a higher concentration of drug outside the cell would ordinarily favor net drug entry into the cell, but if both the cell interior and the drug are negatively charged, then net drug entry into the cell may be impeded. In contrast, a negatively charged cell interior could favor entry of a positively charged drug.
Net diffusion of acidic and basic drugs across lipid bilayer membranes may also be affected by a charge-based phenomenon known as pH trapping, which depends on the drug’s acid dissociation constant (pKa) and the pH gradient across the membrane. For weakly acidic drugs, such as phenobarbital and aspirin, the protonated, electrically neutral form of the drug is predominant in the highly acidic environment of the stomach. The uncharged form of the drug can pass through the lipid bilayers of the gastric and duodenal mucosa, speeding the drug’s absorption (Fig. 3-2). The weakly acidic drug is then effectively trapped as it is deprotonated to its electrically charged form in the more basic environment of the plasma.
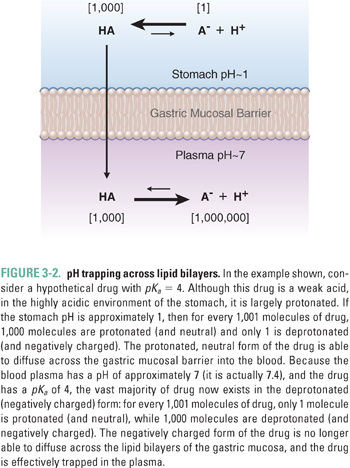
In quantitative terms, the pKa of a drug represents the pH value at which one-half of the drug is present in its ionic form. The Henderson–Hasselbalch equation describes the relationship between the pKa of an acidic or basic Drug A and the pH of the biological medium containing the drug:

where HA is the protonated form of Drug A. For example, consider the hypothetical case of a weakly acidic drug with a pKa of 4. In the stomach, which has a pH of approximately 1, Equation 3-2 becomes:

which simplifies to:

and finally:

The protonated form of the drug is present at 1,000 times the concentration of the deprotonated form, and 99.9% of the drug is in the neutral form. Conversely, in the plasma, where the pH is approximately 7.4, more than 99.9% of the drug is in the deprotonated form (see Fig. 3-2).
The central nervous system (CNS) presents special challenges to pharmacologic therapy. Unlike most other anatomic regions, the CNS is particularly well insulated from foreign substances. The blood–brain barrier uses specialized tight junctions to prevent the passive diffusion of most drugs from the systemic to the cerebral circulation. Therefore, drugs designed to act in the CNS must either be sufficiently small and hydrophobic to traverse biological membranes easily or use existing transport proteins in the blood–brain barrier to penetrate central structures. Hydrophilic drugs that fail to target facilitated or active transport proteins in the blood–brain barrier cannot penetrate the CNS. The blood–brain barrier can be bypassed using intrathecal drug delivery, in which drugs are delivered directly into the cerebrospinal fluid (CSF). Although this approach can be used, for example, to treat infectious meningitis or to provide spinal anesthesia for a cesarean delivery, the intrathecal route is impractical for drugs that must be taken regularly by a patient.
The human body presents formidable obstacles to invasion by microorganisms. The integument has a keratinized outer layer and defensins in the epithelium. Mucous membranes are protected by mucociliary clearance in the trachea, lysozyme secretion from lacrimal ducts, acid in the stomach, and base in the duodenum. These nonspecific defense mechanisms present barriers to drug absorption and may limit the drug’s bioavailability at target organs. Bioavailability, or the fraction of administered drug that reaches the systemic circulation, may depend on the route by which the drug is administered, the chemical form of the drug, and a number of patient-specific factors such as gastrointestinal and hepatic transporters and enzymes.
Bioavailability is defined quantitatively as:
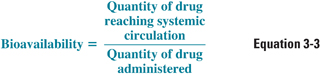
This definition of bioavailability is based on the fact that most drugs reach their molecular and cellular sites of action directly from the systemic circulation. Intravenously administered drugs are injected directly into the systemic circulation; for these drugs, the quantity administered is equivalent to the amount reaching the systemic circulation, and the bioavailability is, by definition, 1.0. In contrast, incomplete gastrointestinal absorption and “first-pass” hepatic metabolism (see below) typically cause the bioavailability of an orally administered drug to be less than 1.0 (Fig. 3-3).
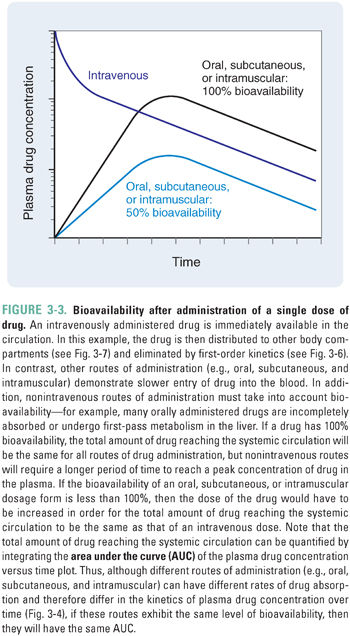
Administration Routes and Rationale
New drugs are designed and tested in a dosage form that is administered by a specific route. Routes of administration are often chosen to take advantage of transport molecules and other mechanisms that permit the drug to enter body tissues. This section discusses the advantages and disadvantages of drug administration by enteral (oral), parenteral, mucous membrane, and transdermal routes (Table 3-1).
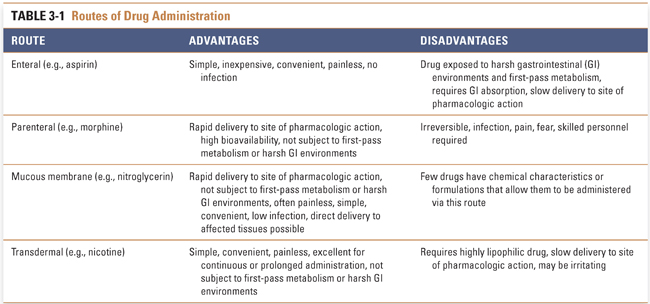
Enteral drug administration, or the administration of a drug by mouth, is the simplest of drug routes. The enteral route of administration exploits existing weaknesses in human barrier defenses, but it exposes the drug to harsh acidic (stomach) and basic (duodenum) environments that could limit its absorption. This route provides many advantages for the patient: oral drugs are easily and conveniently self-administered, and these dosage forms are less likely than other methods to introduce systemic infection as a complication of treatment.
An orally administered drug must be stable during its absorption across the gastrointestinal tract epithelium. Gastrointestinal epithelial cell junctions make paracellular transport across an intact epithelium difficult. Instead, ingested substances (such as drugs) must usually traverse the cell membrane at both apical and basal surfaces before entering the blood. The efficiency of this process is determined by drug size and hydrophobicity and sometimes by the presence of carriers through which the drug may enter and/or exit the cell. In general, hydrophobic and neutral drugs cross cell membranes more efficiently than hydrophilic or charged drugs, unless the membrane contains a carrier molecule that facilitates the transport of hydrophilic substances.
Upon traversing the gastrointestinal epithelium, drugs are carried by the portal system to the liver before entering the systemic circulation. While the portal circulation protects the body from the systemic effects of ingested toxins by delivering these substances to the liver for detoxification, this system may complicate drug delivery. All orally administered drugs are subjected to first-pass metabolism in the liver. In this process, liver enzymes may inactivate a fraction of the ingested drug. Any drug that exhibits significant first-pass metabolism must be administered in a quantity sufficient to ensure that an effective concentration of active drug exits the liver into the systemic circulation, from which it can reach the target organ. Drugs administered by nonenteral routes are not subjected to first-pass liver metabolism.
Parenteral administration, in which a drug is introduced directly into the systemic circulation, cerebrospinal fluid, vascularized tissue, or some other tissue space, immediately overcomes barriers that can limit the effectiveness of orally administered drugs (Table 3-2). Tissue administration results in a rate of onset of drug action that differs among the various body tissues, depending on the rate of blood flow to the tissue. Subcutaneous (SC) administration of a drug into poorly vascularized adipose tissue results in a slower onset of action than injection into well-vascularized intramuscular (IM) spaces. Drugs that are soluble only in oil-based solutions are often administered intramuscularly. Direct introduction of a drug into the venous (intravenous [IV]) or arterial (intra-arterial [IA]) circulation or into the cerebrospinal fluid (intrathecal [IT]) results in the drug reaching its target organ the fastest. Unlike subcutaneous and intramuscular injections, intravenous injection is not typically limited in the amount of drug that can be delivered. Continuous intravenous infusions can allow tight control over peak and steady-state plasma concentrations during drug delivery.
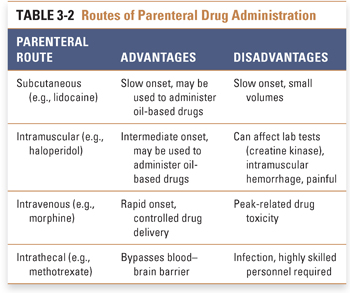
Parenteral administration may be associated with several potential disadvantages, including an increased risk of infection and the requirement for administration by a health care professional. The onset of action of parenterally administered drugs is often rapid, potentially resulting in increased toxicity when such drugs are administered too rapidly or in incorrect doses. These disadvantages must be weighed against the advantages of parenteral administration (such as speed of onset and control of the delivered dose) and the urgency of the indication for pharmacologic therapy.
Administration of drugs across mucous membranes can potentially provide rapid absorption, low incidence of infection, convenience of administration, and avoidance of harsh gastrointestinal environments and first-pass metabolism. Sublingual, ocular, pulmonary, nasal, rectal, urinary, and reproductive tract epithelia have all been used to deliver drugs in the form of liquid drops, rapidly dissolving tablets, aerosols, and suppositories (among other dosage forms). The mucous membranes are highly vascular, permitting the drug to enter the systemic circulation rapidly and to reach its target organ with minimal delay. Drugs may also be administered directly into the target organ, resulting in virtually instantaneous onset of action. This is advantageous in critical conditions such as acute asthma, where drugs such as β-adrenergic agonists are administered via aerosol directly into the airways.
A limited number of drugs have sufficiently high lipophilicity that passive diffusion across the skin is a viable route of administration. Transcutaneously administered drugs are absorbed from the skin and subcutaneous tissues directly into the blood. This route of administration is ideal for a drug that must be slowly and continuously administered over extended periods. There is no associated risk of infection, and drug administration is simple and convenient. The success of transdermal nicotine, estrogen, and scopolamine patches demonstrates the utility of this route of administration (see Chapter 55, Drug Delivery Modalities, for more details on transdermal drug delivery).
Local, Regional, and Systemic Factors Affecting Absorption
The rate and extent of absorption of a drug are affected by local, regional, and systemic factors. In general, a large or rapidly administered dose creates a high local concentration of a drug. A large concentration gradient between the site of administration and the surrounding tissue drives the distribution of the drug into the nearby tissue and/or vasculature. Any factor that decreases the concentration gradient at the site of administration will diminish the driving force of the gradient and may reduce the amount of drug that is distributed into the local tissues. Regional blood flow has the greatest effect in this regard; in a highly perfused region, drug molecules crossing into that compartment are rapidly removed. This effect maintains the drug concentration at a low level in the compartment, allowing the driving force for entry of new drug molecules into the compartment to remain high (see Equation 3-1). For example, volatile general anesthetics are administered via inhalation. The lungs are highly perfused, and the anesthetic is removed rapidly from the lungs into the systemic circulation. Anesthetic does not accumulate in the local circulation, and a concentration gradient promoting diffusion of anesthetic into the blood is maintained (see Chapter 17, General Anesthetic Pharmacology, for more details). In an individual with greater body mass, both the increased surface area for absorption and the larger tissue volumes available for distribution tend to remove a drug from the site of administration faster and increase the rate and extent of drug absorption. The rate of drug absorption affects both the local concentration of a drug (including its plasma concentration) and its duration of action (Fig. 3-4).
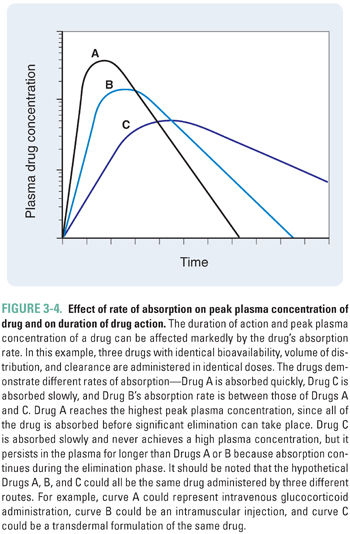
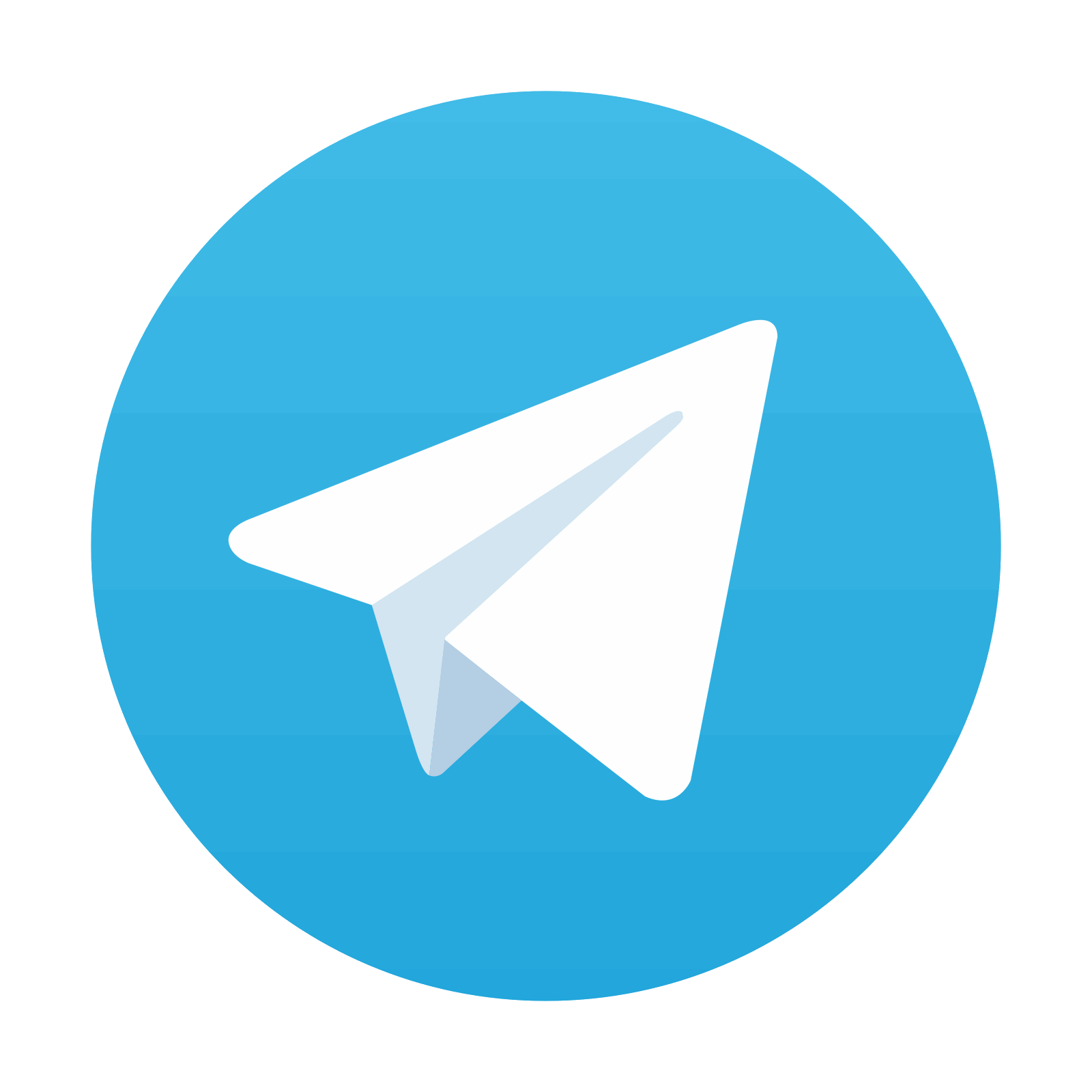
Stay updated, free articles. Join our Telegram channel

Full access? Get Clinical Tree
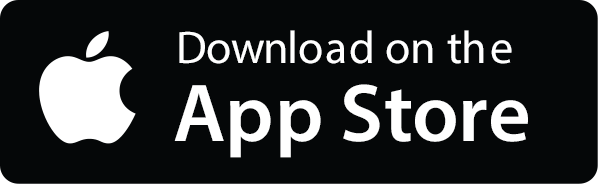
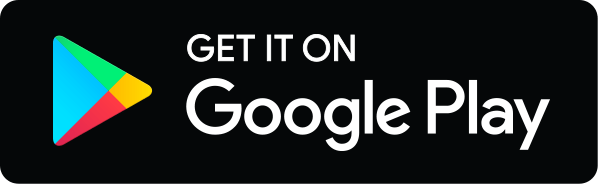