Penicillins, Cephalosporins, and Other β-Lactam Antibiotics
The β-lactam antibiotics—penicillins, cephalosporins, and carbapenems—share a common structure and mechanism of action, inhibition of the synthesis of the bacterial peptidoglycan cell wall. Bacterial resistance against the β-lactam antibiotics continues to increase at a dramatic rate. β-Lactamase inhibitors such as clavulanate can extend the utility of these drugs against β-lactamase-producing organisms. Unfortunately, resistance includes not only production of β-lactamases but also alterations in or acquisition of novel penicillin-binding proteins (PBPs) and decreased entry and/or active efflux of the antibiotic. To a dangerous degree, we are re-entering the pre-antibiotic era, with many nosocomial gram-negative bacterial infections resistant to all available antibiotics.
MECHANISM OF ACTION: INHIBITION OF PEPTIDOGLYCAN SYNTHESIS. Peptidoglycan is a heteropolymeric component of the cell wall that provides rigid mechanical stability. The β-lactam antibiotics inhibit the last step in peptidoglycan synthesis (Figure 53–1).
Figure 53–1 Action of β-lactam antibiotics in Staphylococcus aureus. The bacterial cell wall consists of glycopeptide polymers (a NAM-NAG amino-hexose backbone) linked via bridges between amino acid side chains. In S. aureus, the bridge is (Gly)5–D-Ala between lysines. The cross-linking is catalyzed by a transpeptidase, the enzyme that penicillins and cephalosporins inhibit.
In gram-positive microorganisms, the cell wall is 50-100 molecules thick; in gram-negative bacteria, it is only 1 or 2 molecules thick (Figure 53–2A). The peptidoglycan is composed of glycan chains, which are linear strands of 2 alternating amino sugars (N-acetylglucosamine and N-acetylmuramic acid) that are cross-linked by peptide chains. Peptidoglycan precursor formation takes place in the cytoplasm. The synthesis of UDP–acetylmuramyl-pentapeptide is completed with the addition of a dipeptide, D-alanyl-D-alanine (formed by racemization and condensation of L-alanine). UDP-acetylmuramyl-pentapeptide and UDP-acetylglucosamine are linked (with the release of the uridine nucleotides) to form a long polymer. The cross-link is completed by transpeptidation reaction that occurs outside the cell membrane (Figure 53–2B). The β-lactam antibiotics inhibit this last step in peptidoglycan synthesis (see Figure 53–1), presumably by acylating the transpeptidase via cleavage of the —CO—N— bond of the β-lactam ring. There are additional, related targets for the actions of penicillins and cephalosporins; these are collectively termed PBPs. The transpeptidase responsible for synthesis of the peptidoglycan is 1 of these PBPs. The lethality of penicillin for bacteria appears to involve both lytic and nonlytic mechanisms.
Figure 53–2 A. Structure and composition of gram-positive and gram-negative cell walls. (From Figure 4-11, p 83 of TORTORA, GERALD, MICROBIOLOGY: INTRODUCTION, 3rd Edition, © 1989. Reprinted by permission of Pearson Education, Inc., Upper Saddle River, NJ.) B. Penicillin binding protein 2 (PBP2) from S. aureus. PBP2 has 2 enzymatic activities that are crucial to synthesis of the peptidoglycan layers of bacterial cell walls: a transpeptidase (TP) that cross-links amino acid side chains, and a glycosyltransferase (GT) that links subunits of the glycopeptide polymer (see Figure 53–1). The transpeptidase and glycosyltransferase domains are separated by a linker region. The glycosyltransferase is thought to be partially embedded in the membrane.
MECHANISMS OF BACTERIAL RESISTANCE TO PENICILLINS AND CEPHALOSPORINS. Bacteria can be resistant to β-lactam antibiotics by myriad mechanisms.
A sensitive strain may acquire resistance by mutations that decrease the affinity of PBPs for the antibiotic. Because the β-lactam antibiotics inhibit many different PBPs in a single bacterium, the affinity for β-lactam antibiotics of several PBPs must decrease for the organism to be resistant. Methicillin-resistant Staphylococcus aureus (MRSA) are resistant via acquisition of an additional high-molecular-weight PBP (via a transposon) with a very low affinity for all β-lactam antibiotics; this mechanism is responsible for methicillin resistance in the coagulase-negative staphylococci. Altered PBPs with decreased affinity for β-lactam antibiotics are acquired by homologous recombination between PBP genes of different bacterial species. Four of the 5 high-molecular-weight PBPs of the most highly penicillin-resistant Streptococcus pneumoniae isolates have decreased affinity for β-lactam antibiotics as a result of interspecies homologous recombination events (Figure 53–3). In contrast, isolates with high-level resistance to third-generation cephalosporins contain alterations of only 2 of the 5 high-molecular-weight PBPs because the other PBPs have inherently low affinity for the third-generation cephalosporins.
Figure 53–3 Mosaic penicillin binding protein 2B genes in penicillin-resistant pneumococci. The divergent regions in the PBP2B genes of 7 resistant pneumococci from different countries are shown. These regions have been introduced from at least 3 sources, 1 of which appears to be Streptococcus mitis. The approximate percent sequence divergence of the divergent regions from the PBP2B genes of susceptible pneumococci is shown. (From Spratt BG. Resistance to antibiotics mediated by target alterations. Science, 1994;264:388–393. Reprinted with permission from AAAS.)
Bacterial resistance to the β-lactam antibiotics also results from the inability of the agent to penetrate to its site of action (Figure 53–4). In gram-positive bacteria, the peptidoglycan polymer is very near the cell surface (see Figure 53–2) and small β-lactam antibiotic molecules can penetrate easily to the outer layer of the cytoplasmic membrane and the PBPs. In gram-negative bacteria, the inner membrane is covered by the outer membrane, lipopolysaccharide, and capsule (see Figure 53–2). The outer membrane functions as an impenetrable barrier for some antibiotics. Some small hydrophilic antibiotics, however, diffuse through aqueous channels in the outer membrane that are formed by proteins called porins. The number and size of pores in the outer membrane vary among different gram-negative bacteria, thereby providing greater or lesser access for antibiotics to the site of action. Active efflux pumps serve as another mechanism of resistance, removing the antibiotic from its site of action before it can act (see Figure 53–4).
Figure 53–4 Antibiotic efflux pumps of gram-negative bacteria. Multidrug efflux pumps traverse both the inner and outer membranes of gram-negative bacteria. The pumps are composed of a minimum of 3 proteins and are energized by the proton motive force. Increased expression of these pumps is an important cause of antibiotic resistance. (Reprinted with permission from Oxford University Press. Nikaido H. Antibiotic resistance caused by gram-negative multidrug efflux pumps. Clin Infect Dis, 1998;27[suppl I]:S32–S41. © 1998 by the Infectious Diseases Society of America. All rights reserved.)
Bacteria also can destroy β-lactam antibiotics enzymatically via the action of β-lactamases (Figures 53–2 and 53–5). β-Lactamases are grouped into four classes: A through D. The substrate specificities of some of these classes are relatively narrow; these often are described as either penicillinases or cephalosporinases. Other “extended-spectrum” enzymes are less discriminant and can hydrolyze a variety of β-lactam antibiotics. In general, gram-positive bacteria produce and secrete a large amount of β-lactamase (see Figure 53–2A). Most of these enzymes are penicillinases. The information for staphylococcal penicillinase is encoded in a plasmid; this may be transferred by bacteriophage to other bacteria and is inducible by substrates. In gram-negative bacteria, β-lactamases are found in relatively small amounts but are located in the periplasmic space between the inner and outer cell membranes (see Figure 53–2A) for maximal protection of the microbe. β-Lactamases of gram-negative bacteria are encoded either in chromosomes or in plasmids, and may be constitutive or inducible. The plasmids can be transferred between bacteria by conjugation. These enzymes can hydrolyze penicillins, cephalosporins, or both.
Figure 53–5 Structure of penicillins and products of their enzymatic hydrolysis.
OTHER FACTORS THAT INFLUENCE THE ACTIVITY OF β-LACTAM ANTIBIOTICS. Microorganisms adhering to implanted prosthetic devices (e.g., catheters, artificial joints, prosthetic heart valves) produce biofilms. Bacteria in biofilms produce extracellular polysaccharides and, in part owing to decreased growth rates, are much less sensitive to antibiotic therapy. The β-lactam antibiotics are most active against bacteria in the logarithmic phase of growth and have little effect on microorganisms in the stationary phase. Similarly, bacteria that survive inside viable cells of the host generally are protected from the action of the β-lactam antibiotics.
THE PENICILLINS
Despite the emergence of microbial resistance, the penicillins are currently the drugs of choice for a large number of infectious diseases. Penicillins (Figure 53–5) consist of a thiazolidine ring (A) connected to a β-lactam ring (B) to which is attached a side chain (R). The penicillin nucleus itself is the chief structural requirement for biological activity. Side chains can be added that alter the susceptibility of the resulting compounds to inactivating enzymes (β-lactamases) and that change the antibacterial activity and the pharmacological properties of the drug (Table 53–1).
Table 53–1
Chemical Structures of Selected Penicillins
UNITAGE OF PENICILLIN. The international unit of penicillin is the specific penicillin activity contained in 0.6 μg of the crystalline sodium salt of penicillin G. One milligram of pure penicillin G sodium thus equals 1667 units; 1.0 mg of pure penicillin G potassium represents 1595 units. The dosage and the antibacterial potency of the semisynthetic penicillins are expressed in terms of weight.
CLASSIFICATION OF THE PENICILLINS AND SUMMARY OF THEIR PHARMACOLOGICAL PROPERTIES
Penicillins are classified according to their spectra of antimicrobial activity.
• Penicillin G and its close congener penicillin V are highly active against sensitive strains of gram-positive cocci, but they are readily hydrolyzed by penicillinase. Thus, they are ineffective against most strains of S. aureus.
• The penicillinase-resistant penicillins (methicillin, discontinued in U.S.), nafcillin, oxacillin, cloxacillin (not currently marketed in the U.S.), and dicloxacillin have less potent antimicrobial activity against microorganisms that are sensitive to penicillin G, but they are the agents of first choice for treatment of penicillinase-producing S. aureus and Staphylococcus epidermidis that are not methicillin-resistant.
• Ampicillin, amoxicillin, and others make up a group of penicillins whose antimicrobial activity is extended to include gram-negative microorganisms (e.g., Haemophilus influenzae, Escherichia coli, and Proteus mirabilis). These drugs are frequently administered with a β-lactamase inhibitor such as clavulanate or sulbactam to prevent hydrolysis by class A β-lactamases.
• Agents with extended antimicrobial activity that includes Pseudomonas, Enterobacter, and Proteus spp. [carbenicillin (discontinued in U.S.), its indanyl ester (carbenicillin indanyl), and ticarcillin (marketed with clavulanate in U.S.)] These agents are inferior to ampicillin against gram-positive cocci and Listeria monocytogenes and are less active than piperacillin against Pseudomonas.
• Mezlocillin, azlocillin (both discontinued in U.S.), and piperacillin have excellent antimicrobial activity against many isolates of Pseudomonas, Klebsiella, and certain other gram-negative microorganisms. Piperacillin retains the activity of ampicillin against gram-positive cocci and L. monocytogenes.
General common properties: Following absorption of an oral dose, penicillins are distributed widely throughout the body. Therapeutic concentrations of penicillins are achieved readily in tissues and in secretions such as joint fluid, pleural fluid, pericardial fluid, and bile. Penicillins do not penetrate living phagocytic cells to a significant extent, and only low concentrations of these drugs are found in prostatic secretions, brain tissue, and intraocular fluid. Concentrations of penicillins in cerebrospinal fluid (CSF) are variable but are <1% of those in plasma when the meninges are normal. When there is inflammation, concentrations in CSF may increase to as much as 5% of the plasma value. Penicillins are eliminated rapidly, particularly by glomerular filtration and renal tubular secretion, such that their half-lives in the body are short, typically 30-90 min. As a consequence, concentrations of these drugs in urine are high.
PENICILLIN G AND PENICILLIN V
ANTIMICROBIAL ACTIVITY. The antimicrobial spectra of penicillin G (benzylpenicillin) and penicillin V (the phenoxymethyl derivative) are very similar for aerobic gram-positive microorganisms. However, penicillin G is 5-10 times more active than penicillin V against Neisseria spp. and certain anaerobes. Most streptococci (but not enterococci) are very susceptible. However, penicillin-resistant viridans streptococci and S. pneumoniae are becoming more common. Penicillin-resistant pneumococci are especially common in pediatric populations. Many penicillin-resistant pneumococci also are resistant to third-generation cephalosporins. More than 90% of strains of staphylococci isolates are now resistant to penicillin G, (and nearly half are methicillin-resistant). Most strains of S. epidermidis and many strains of gonococci are also resistant. With rare exceptions, meningococci are quite sensitive to penicillin G.
Most anaerobic microorganisms, including Clostridium spp., are highly sensitive. Bacteroides fragilis is an exception, displaying resistance to penicillins and cephalosporins by virtue of expressing a broad-spectrum cephalosporinase. Some strains of Prevotella melaninogenicus also have acquired this trait. Actinomyces israelii, Streptobacillus moniliformis, Pasteurella multocida, and L. monocytogenes are inhibited by penicillin G. Most species of Leptospira are moderately susceptible to the drug. One of the most sensitive microorganisms is Treponema pallidum. Borrelia burgdorferi, the organism responsible for Lyme disease, also is susceptible. None of the penicillins is effective against amebae, plasmodia, rickettsiae, fungi, or viruses.
ADME
Oral Administration of Penicillin G. About one-third of an orally administered dose of penicillin G is absorbed from the GI tract. Gastric juice at pH 2 rapidly destroys the antibiotic. Absorption is rapid, and maximal concentrations in blood are attained in 30-60 min. Ingestion of food may interfere with enteric absorption of all penicillins. Thus, oral penicillin G should be administered at least 30 min before a meal or 2 h after. Despite the convenience of oral administration of penicillin G, this route should be used only in infections in which clinical experience has proven its efficacy.
Oral Administration of Penicillin V. The virtue of penicillin V in comparison with penicillin G is that it is more stable in an acidic medium and therefore is better absorbed from the GI tract, yielding plasma concentrations 2-5 times those provided by penicillin G.
Parenteral Administration of Penicillin G. After intramuscular injection, peak concentrations in plasma are reached within 15-30 min, declining rapidly thereafter (t1/2 ~ 30 min). Repository preparations of penicillin G increase the duration of the effect. The compound currently favored is penicillin G benzathine (BICILLIN L-A, PERMAPEN), which releases penicillin G slowly from the area in which it is injected and produces relatively low but persistent concentrations in the blood. The average duration of demonstrable antimicrobial activity in the plasma is ~26 days. It is administered once monthly for rheumatic fever prophylaxis and can be given in a single injection to treat streptococcal pharyngitis. The persistence of penicillin in the blood after a suitable intramuscular dose of penicillin G benzathine reduces cost, need for repeated injections, and local trauma. The local anesthetic effect of penicillin G benzathine is comparable with that of penicillin G procaine.
Distribution. Penicillin G is distributed widely throughout the body, but the concentrations in various fluids and tissues differ widely. Its apparent volume of distribution is ~0.35 L/kg. Approximately 60% of the penicillin G in plasma is reversibly bound to albumin. Significant amounts appear in liver, bile, kidney, semen, joint fluid, lymph, and intestine. Probenecid markedly decreases the tubular secretion of the penicillins and also produces a significant decrease in the apparent volume of distribution of the penicillins.
Penetration into Cerebrospinal Fluid. Penicillin does not readily enter the CSF but penetrates more easily when the meninges are inflamed. The concentrations are usually in the range of 5% of the value in plasma and are therapeutically effective against susceptible microorganisms. Penicillin and other organic acids are secreted rapidly from the CSF into the bloodstream by an active transport process. Probenecid competitively inhibits this transport and thus elevates the concentration of penicillin in CSF. In uremia, other organic acids accumulate in the CSF and compete with penicillin for secretion; the drug occasionally reaches toxic concentrations in the brain and can produce convulsions.
Excretion. Approximately 60-90% of an intramuscular dose of penicillin G in aqueous solution is eliminated in the urine, largely within the first hour after injection. The remainder is metabolized to penicilloic acid (see Figure 53–5). The t1/2 for elimination of penicillin G is ~30 min in normal adults. Approximately 10% of the drug is eliminated by glomerular filtration and 90% by tubular secretion. Renal clearance approximates the total renal plasma flow. Clearance values are considerably lower in neonates and infants; as a result penicillin persists in the blood several times longer in premature infants than in children and adults. The t1/2 of the antibiotic in children <1 week of age is 3 h; by 14 days of age it is 1.4 h. After renal function is fully established in young children, the rate of renal excretion of penicillin G is considerably more rapid than in adults. Anuria increases the t1/2 of penicillin G from 0.5 h to ~10 h. When renal function is impaired, 7-10% of the antibiotic may be inactivated each hour by the liver. The dose of the drug must be readjusted during dialysis and the period of progressive recovery of renal function. If hepatic insufficiency also is present, the t1/2 will be prolonged even further.
THERAPEUTIC USES
Pneumococcal Infections. Penicillin G remains the agent of choice for the management of infections caused by sensitive strains of S. pneumoniae, but resistance is an increasing problem.
Pneumococcal Pneumonia. Pneumococcal pneumonia should be treated with a third-generation cephalosporin or with 20-24 million units of penicillin G daily by constant intravenous infusion. If the organism is sensitive to penicillin, then the dose can be reduced. For parenteral therapy of sensitive isolates of pneumococci, penicillin G is favored. Therapy should be continued for 7-10 days, including 3-5 days after the patient’s temperature has returned to normal.
Pneumococcal Meningitis. Pneumococcal meningitis should be treated with a combination of vancomycin and a third-generation cephalosporin until it is established that the infecting pneumococcus is penicillin-sensitive. Dexamethasone given at the same time as antibiotics is associated with an improved outcome. The recommended therapy is 20-24 million units of penicillin G daily by constant intravenous infusion or divided into boluses given every 2-3 h for 14 days.
Streptococcal Infections. Streptococcal pharyngitis (including scarlet fever) is the most common disease produced by Streptococcus pyogenes (group A β-hemolytic streptococcus). Penicillin-resistant isolates have yet to be observed. The preferred oral therapy is with penicillin V, 500 mg every 6 h for 10 days. Penicillin therapy of streptococcal pharyngitis reduces the risk of subsequent acute rheumatic fever; however, current evidence suggests that the incidence of glomerulonephritis that follows streptococcal infections is not reduced to a significant degree by treatment with penicillin.
Streptococcal Toxic Shock and Necrotizing Fascitis. These are life-threatening infections associated with toxin production and are treated optimally with penicillin plus clindamycin (to decrease toxin synthesis).
Streptococcal Pneumonia, Arthritis, Meningitis, and Endocarditis. These uncommon conditions should be treated with penicillin G when they are caused by S. pyogenes; daily doses of 12-20 million units are administered intravenously for 2-4 weeks (4 weeks for endocarditis).
Infections Caused by Other Streptococci. The viridans group of streptococci is the most common cause of infectious endocarditis. These are nongroupable α-hemolytic microorganisms that are increasingly resistant to penicillin G. It is important to determine quantitative microbial sensitivities to penicillin G in patients with endocarditis. Patients with penicillin-sensitive viridans group streptococcal endocarditis can be treated successfully with daily doses of 12-20 million units of intravenous penicillin G for 2 weeks in combination with gentamicin 1 mg/kg every 8 h. The recommended therapy for penicillin- and aminoglycoside-sensitive enterococcal endocarditis is 20 million units of penicillin G or 12 g ampicillin daily administered intravenously in combination with a low dose of gentamicin. Therapy usually should be continued for 6 weeks.
Infections with Anaerobes. Many anaerobic infections are caused by mixtures of microorganisms. Most are sensitive to penicillin G. An exception is the B. fragilis group, in which up to 75% of strains may be resistant. Pulmonary and periodontal infections usually respond well to penicillin G; clindamycin may be more effective than penicillin for therapy of lung abscess. Mild-to-moderate infections at these sites may be treated with oral medication (either penicillin G or penicillin V 400,000 units [250 mg] 4 times daily). More severe infections should be treated with 12-20 million units of penicillin G intravenously. Brain abscesses also frequently contain several species of anaerobes, and most authorities recommend high doses of penicillin G (20 million units per day) plus metronidazole or chloramphenicol.
Staphylococcal Infections. Most staphylococcal infections are caused by microorganisms that produce penicillinase. Hospital-acquired methicillin-resistant staphylococci are resistant to penicillin G, all the penicillinase-resistant penicillins, and the cephalosporins. Isolates occasionally may appear to be sensitive to various cephalosporins in vitro, but resistant populations arise during therapy and lead to failure. Vancomycin, linezolid, quinupristin-dalfopristin, and daptomycin are active for infections caused by these bacteria, although reduced susceptibility to vancomycin has been observed. Community-acquired MRSA in many cases retains susceptibility to trimethoprim-sulfamethoxazole, doxycycline, and clindamycin.
Meningococcal Infections. Penicillin G remains the drug of choice for meningococcal disease. Patients should be treated with high doses of penicillin given intravenously (see above). The occurrence of penicillin-resistant strains should be considered in patients who are slow to respond to treatment. Penicillin G does not eliminate the meningococcal carrier state, and its administration thus is ineffective as a prophylactic measure.
Gonococcal Infections. Gonococci gradually have become more resistant to penicillin G, and penicillins are no longer the therapy of choice. For uncomplicated gonococcal urethritis, a single intramuscular injection of 250 mg ceftriaxone is the recommended treatment. Gonococcal arthritis, disseminated gonococcal infections with skin lesions, and gonococcemia should be treated with ceftriaxone 1 g daily given either intramuscularly or intravenously for 7-10 days. Ophthalmia neonatorum also should be treated with ceftriaxone for 7-10 days (25-50 mg/kg/day intramuscularly or intravenously).
Syphilis. Therapy of syphilis with penicillin G is highly effective. Primary, secondary, and latent syphilis of <1-year duration may be treated with penicillin G procaine (2.4 million units per day intramuscularly) plus probenecid (1.0 g/day orally) for 10 days or with 1-3 weekly intramuscular doses of 2.4 million units of penicillin G benzathine (3 doses in patients with HIV infection). Patients with neurosyphilis, or cardiovascular syphilis typically receive intensive therapy with 20 million units of penicillin G daily for 10 days. There are no proven alternatives for treating syphilis in pregnant women, so penicillin-allergic individuals must be acutely desensitized to prevent anaphylaxis. Infants with congenital syphilis discovered at birth or during the postnatal period should be treated for at least 10 days with 50,000 units/kg daily of aqueous penicillin G in 2 divided doses or 50,000 units/kg of procaine penicillin G in a single daily dose.
Most patients with secondary syphilis develop the Jarisch-Herxheimer reaction, including chills, fever, headache, myalgias, and arthralgias occurring several hours after the first dose of penicillin. This reaction is thought to be due to release of spirochetal antigens with subsequent host reactions to the products. Aspirin gives symptomatic relief, and therapy with penicillin should not be discontinued.
Actinomycosis. Penicillin G is the agent of choice for the treatment of all forms of actinomycosis (10-20 million units of penicillin G intravenously per day for 6 weeks). Surgical drainage or excision of the lesion may be necessary before cure is accomplished.
Diphtheria.
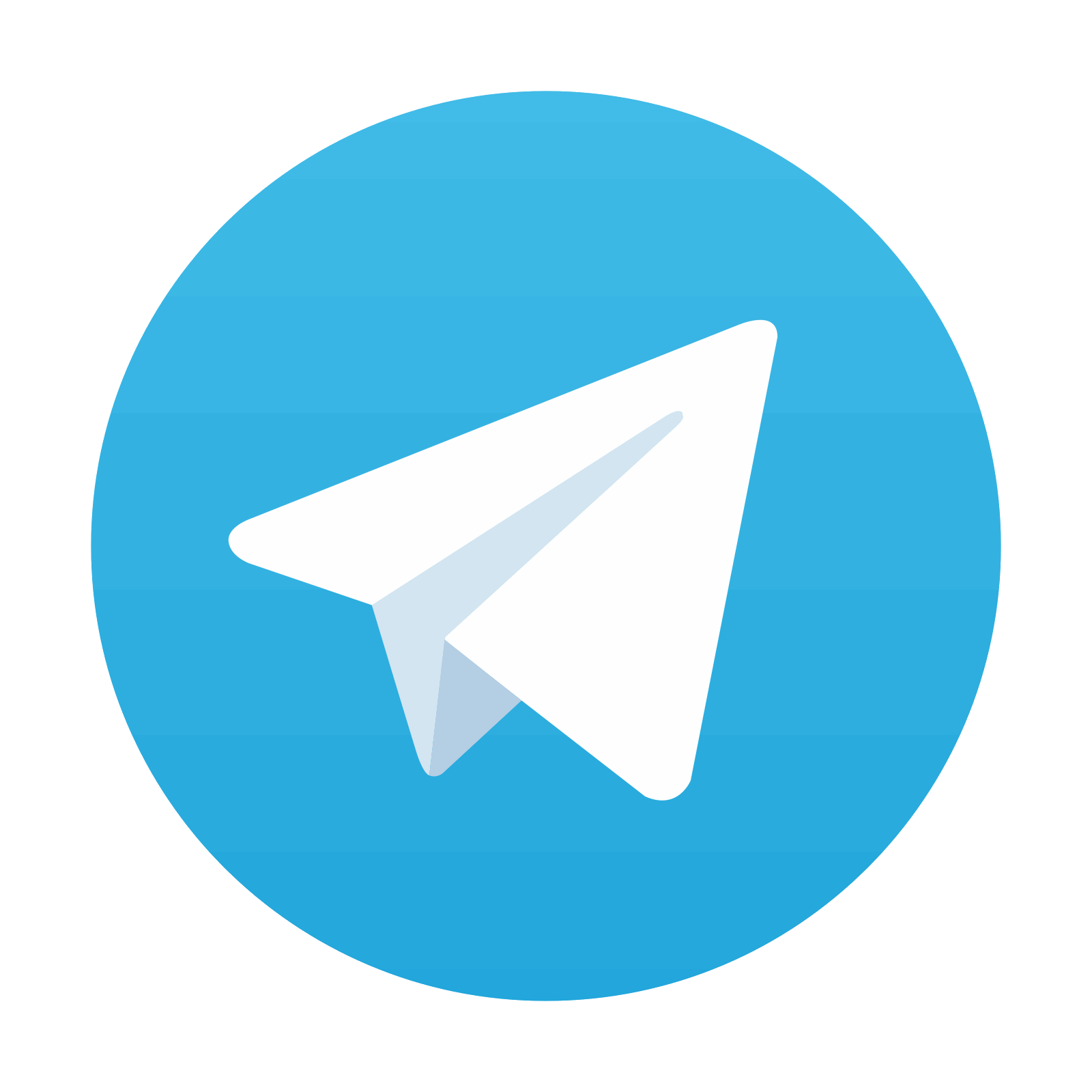
Stay updated, free articles. Join our Telegram channel

Full access? Get Clinical Tree
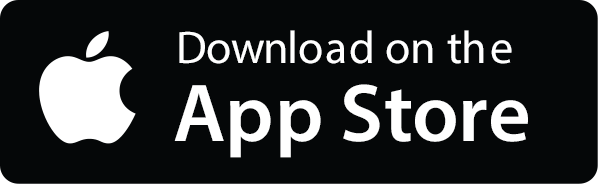
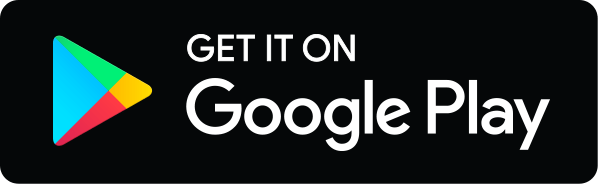