(1)
Canberra, Aust Capital Terr, Australia
Scientific Name
Tanacetum parthenium (L.) Sch. Bip.
Synonyms
Aphanostephus pinulensis J.M. Coult., Chamaemelum parthenium (L.) E.H.L. Krause, Chrysanthemum parthenium (L.) Bernh., Chrysanthemum parthenium (L.) Pers. (illeg.), Chrysanthemum praelatum Vent., Dendranthema parthenium (L.) Des Moul., Leucanthemum odoratum (Lam.) Dulac, Leucanthemum parthenium (L.) Gren. & Godr., Matricaria parthenium L., Parthenium matricaria Gueldenst., Pontia matricaria Bubani, Pyrethrum buschianum Sosn., Pyrethrum demetrii Manden., Pyrethrum divaricatum (Sosn.) Sosn., Pyrethrum glanduliferum Sommier & Levier, Pyrethrum grossheimii Sosn., Pyrethrum sericeum var. divaricatum (Sosn.) Sosn., Pyrethrum sevanense Sosn. ex Grossh., Tanacetum grossheimii (Sosn.) Muradyan.
Family
Asteraceae
Common/English Names
Bachelor’s Button, Bridal Roses, Camphor Geranium, Common Feverfew, Double Feverfew, European Feverfew, Feather-Fully, Febrifuge Plant, Feather Foil, Fetter-Foe, Feverfew, Feverfew Chrysanthemum, Feverfew-Chamomile, Flirtroot, Flitwort, Golden Feather, Lesser Feverfew, Matricaria Parthenium, Midsummer Daisy, Mother Herb, Nosebleed Parthenium, Pellitory, Santa Maria, White-Wort, Wild Chamomile, Wild Quinine, Eddygen Fenyw, Grande Chamomile, Mutterkraut, Vetter-Voo, Feverfew, Featherfew, Altamisa, Bachelor’s Button, Featherfoil, Febrifuge Plant, Midsummer Daisy, Nosebleed, Wild Chamomile, Wild Quinine, Chamomile Grande, Chrysanthemum Atricaire, Federfoy, Flirtwort
Vernacular Names
Albanian: Karajpel Partenianr
Brazil: Crisântemo, Eston Com Febre (Portuguese)
Chile: Pireto De Jardin
Chinese: Xiao Bai Ju
Corsican: Martricaria
Croatian: Amarak, Droptiniče Koleno, Majčinski Vratić, Mater-Hćerka, Meta Gorka
Czech: Kopretina Řimbaba, Kopretima Řimbaba, Oimbaba obecná, Řimbaba obecná
Danish: Jomfruurt, Kvindeurt, Lugtefis, Matrem, Moderurt, Pigeurt
Dutch: Boerenwormkruid, Kamille sort, Moederkruid, Wormkruidsoort
Eastonian: Lõhnav Neitsikummel, Lõhnav püreeter
Esperanto: Krizantemo ara, Tanaceto ara
Finnish: Reunuspaeivaenkakkara, Tarhakakkara
French: Camomille grande, Espargoute, Grande Camomile, Herb À Vers, Malherbe, Mandiane, Matricaire, Pyrèthre Doré, Pyrèthre Mousse, Tanaisie Parthénium
Gaelic: Bossan Pheddyr, Lus deartán, Lus Y Chiassagh
German: Bertram, Die Wucherblume, Falsche Kamille, Fieberkraut, Jungfernkraut, Metram, Mutterkamille Mutterkraut, Mutterkraut, Römische Kamille, Zierkamille
Hungarian: Anyafű, Pirétrum, Őszi aranyvirág, Őszi margitvirág
Icelandic: Glitbrá
Italian: Amarella, Amareggiola, Erba-Amara Vera, Matricale, Matricale Marga, Partenio
Juan Fernandez Islands: Santa Maria (Spanish)
Latvian: Meiteņu Biškrēsliņš
Norwegian: Matre
Polish: Wrotycz Maruna, Złocień Maruna, Złocień zwyczajny
Portuguese: Tanaceto
Russian: Piretrum Devičij
Slovašcina: Beli vratič, Materine drobtinice, Vratič beli
Slovencina: Rimbaba Obyčajná
Spanish: Altamisa, Amargaza, Botón de plata, Camomila de Aragón, Erísimo, Hierba santa, Hierba de Santa María, Matricaria, Pelitre, Piretro
Swedish: Bertram, Mattram, Parthenion
Turkey: Gümüşdüğme
Welsh: Wermod Wen, Chweryn Gwyn, Chwerwyn yr Ardd, Llysiau’r Fam, Meddygon, Menyw, Tarfgryd, Tormwyth, Tormyth
Origin/Distribution
Feverfew is indigenous to Eurasia—Balkan Peninsula in Southeastern Europe, Anatolia and the Caucasus. It is adventive by introduction in most of Europe and the Mediterranean area, also in North America and Chile in South America. Feverfew is now grown in North and South America, Europe, North Africa, China, Japan and Australia.
Agroecology
A cool climate species, feverfew occurs in mountain scrub, rocky slopes, fields, grassland, riverbeds, forest edges, along roadsides, stream banks, disturbed sites, waste places, urban sites and even walls from 10 to 2,500 m altitude. It thrives in full sun or shade (40–80 %). It occurs as a weed in gardens, yards and rubbish tips.
Edible Plant Parts and Uses
Flowers are edible and dried flowers are used as herbal tea, in wine and in certain pastries (Uphof 1968; Facciola 1990; Rop et al. 2012). The plant is used in cooking to impart a deliciously aromatic bitter taste to certain foods (Chiej 1984).
Studies found that an extraction temperature of 80 °C was optimum to provide aqueous extracts rich in parthenolide content and phenolic content and, with a desirable colour, suitable for incorporation into a functional beverage (Marete et al. 2009).
Botany
A perennial or annual, pungent, aromatic erect herb, 20–50 (−70 cm) high with numerous puberulous, grooved, terete stem branching from a rootstock with fibrous roots. Leaves alternate, radical or cauline, punctate glandular on both surfaces, lime-green, glabrous or weakly pubescent on 2.5–8 cm long petioles; upper leaves smaller and sessile to subsessile. Lamina ovate-oblong, 2–8 cm × 1.5–5 cm, bipinnate or pinnatipartite into obtuse-subacute ultimate segments. Capitula radiate, heterogamous, few to several on 2.5–3.5 cm peduncle, formed in terminal dense corymbs. Involucre hemispherical, 3-seriate phyllaries imbricate, finely pubescent, green, outer lanceolate, inner oblong-oblanceolate, weakly pubescent to glabrescent. Receptacle convex of flattish. Ray florets, 5–12, female, with oblong, obtusely-3-lobed white ligules (Plate 1). Disc florets, numerous, yellow, tubular-infundibuliform with 5-toothed corolla tube, ovary of 2 fused carpels, stamens 5. Cypselas oblong, greyish brown, 1–1.5 mm, with 6–8 white ridges and sessile glands. Pappus membranous, coroniform with irregular lobes.


Plate 1
Feverfew flowers (Louis M Landry)
Nutritive/Medicinal Properties
Flower Phytochemicals
Rop et al. (2012) reported that edible flowers of Chrysanthemum parthenium (Tanacetum parthenium) had a dry matter content (%w/w) of 9.86 %, crude protein of 6.77 g/kg and the following elements (mg/kg fresh mass (FM)): P 501.29 mg, K 3600.34 mg, Ca 341.32 mg, Mg 159.17 mg, Na 113.31 mg, Fe 5.83 mg, Mn 7.33 mg, Cu 2.35 mg, Zn 5.94 mg and Mo 0.31 mg. The flowers had total antioxidant capacity of 4.21 g ascorbic acid equivalents/kg FM, total phenolic content of 2.72 g gallic acid/kg FM and total flavonoid content of 1.29 g rutin/kg FM. Flowers of field-grown plants had higher concentration of parthenolide (3.8 % w/w dw) than those from hydroponic plants (1.2 % w/w dw) (Pedneault et al. 2002). A hydroalcohol solution was found to extract more melatonin from the flowers than the hot water extract (Ansari et al. 2010).
The oil yield obtained by SFE (supercritical fluid extraction) of feverfew-flowering heads was similar to that resulting from hexane extraction (5.4 and 4.92 %, respectively) (Kery et al. 1999). The yield of essential oil obtained by steam distillation was only approximately one tenth that obtained by SFE or hexane extraction. Chemical analysis of the volatile constituents of feverfew revealed the presence of 17 major components, 13 of which were identified. The main constituents of each product were camphor and chrysanthenyl acetate. Other minor volatile components identified were α-pinene, camphene, β-pinene, limonene, p-cymene, eucalyptol, γ-terpinene, linalool, α-thujone, borneol and α-terpineol.
The essential oil contents of T. parthenium based on the dry weight of wild and cultivated plants from Iran were 0.1 and 0.4 % (w/w), respectively (Mirjalili et al. 2007). In total, 33 and 30 constituents were identified and quantified in wild and cultivated samples, representing 95.7 and 97.5 % of the total oil, respectively. In the oil of the wild sample, camphor (50.5 %), germacrene-D (9.2 %), camphene (7.7 %), (E)-sesquilavandulol (4.8 %) and (E)-myrtanol (4.7 %) were the major compounds; the minor components were tricylene (1.1 %), α-pinene (0.5 %), dihydrosabinene (0.2 %), sabinene (0.2 %), β-pinene (0.4 %), α-phellandrene (0.1 %), p-cymene (0.5 %), limonene (0.5 %), γ-terpinene (0.4 %), filifolone (1.4 %), chrysanthenone (1.3 %), pinocarvone (tr), borneol (0.6 %), 4-terpineol (0.5 %), lavandulyl acetate (0.1 %), bornyl acetate (1.5 %), α-capaene (0.3 %), β-elemene (0.1 %), (E)-caryophyllene (0.3 %), (Z)-β-farnesene (2.1 %), γ-gurjunene (0.1 %), (Z,E)-α-farnesene (1.5 %), bicyclogermacrene (1.1 %), δ-cadinene (0.2 %), spathulenol (0.4 %), viridflorol (0.6 %), α-cadinol (1.5 %), valerarone (0.1 %) and (Z)-nucliferol acetate (1.2 %). In the oil of cultivated plant, the major components were camphor (57.6 %), (E)-chrysanthenyl acetate (25.1 %), camphene (4.6 %) and bornyl angelate (2.2 %); the minor components were tricylene (0.2 %), α-pinene (0.3 %), sabinene (tr), β-pinene (tr), p-cymene (1.1 %), limonene (0.3 %), γ-terpinene (0.3 %), terpinolene (tr), linalool (0.2 %), α-campholenal (0.4 %), α-cyclocitral (0.1 %), pinocarvone (tr), borneol (0.5 %), 4-terpineol (0.5 %), α-terpineol (tr), (E)-carveol (tr), bornyl acetate (1.4 %), neryl acetate (tr), β-terpinyl acetate (0.1 %), α-copaene (tr), (E)-caryophyllene (0.5 %), (Z)-β-farnesene (1.2 %), germacrene D (0.2 %), bornyl angelate (2.2 %), caryophyllene oxide (0.4 %), viridiflorol (0.1 %) and α-cadinol (0.2 %). It was found that (E)-chrysanthenyl acetate and bornyl angelate were only observed in the oil of the cultivated sample, and (E)-sesquilavandulol (4.8 %) and (E)-myrtanol (4.7 %) were completely absent in the oil of this sample. Oxygenated monoterpenes predominated in the oil of cultivated (85.9 %) and wild (60.6 %) samples. The oil of wild plants contained primarily of ten monoterpene hydrocarbons (11.6 %), nine oxygenated monoterpenes (60.6 %), eight sesquiterpene hydrocarbons (14.9 %) and six oxygenated sesquiterpenes (8.6 %), while the oil of cultivated plants consisted mainly of nine monoterpene hydrocarbons (6.8 %), 13 oxygenated monoterpenes (85.9 %), four sesquiterpene hydrocarbons (1.9 %) and four oxygenated sesquiterpenes (2.9 %).
Thirty compounds were identified in the flower-head essential oil, and the major compounds were camphor 48.4 %, chrysanthenyl acetate 26.3 % and camphene 8.76 % (Rateb et al. 2007). Other minor components included cis-2-octene, butyl acetate, tricylene, α-pinene, camphene, sabinene, β-pinene, p-cymene, limonene, 1,8-cineole, γ-terpinene, p-cymenene, borneol, terpin-4-ol, α-terpineol, linalool acetate, thymol, E-pinocarvyl acetate, E-caryophyllene, germacrene D, germacrene D-4-ol, Z-chrysanthenyl angelate, bornyl angelate, β-caryophyllene oxide, globulol, E,E-farnesol and E,E,-farnesyl acetate.
Seed Phytochemicals
Seeds were found to be rich in parthenolide and to contain tanaparthin-α-peroxide (Kaplan et al. 2002). Majdi et al. (2011) found that the highest expression of germacrene A synthase (TpGAS) was in glandular trichomes which also contained the highest concentration of parthenolide, suggesting that glandular trichomes were the secretory tissues where parthenolide biosynthesis and accumulation occurred. Expressions of (E)-β-caryophyllene synthase (TpCarS), amorpha-4,11-diene synthase, were also observed.
Aerial Parts/Plant Phytochemicals
Three pinene derivatives, two spiroketal enol ether polyines, four germacranolides and six guaianolides, two of them being endoperoxides and two secoguaianolides, were isolated from the aerial parts: germacranolide—parthenolide; eudesmolides reynosin and santamarin; guaianolides canin and artecanin; and non-lactone terpenes including camphor, β-farmesene and germacrene D, chrysanthenyl acetate, bornyl acetate, bornyl angelate, α-pinene derivatives and spiroketal enol ether polyines (Bohlmann and Zdero 1982). Compounds isolated from feverfew included sesquiterpene lactones, parthenolide, 3-β-hydroxyparthenolide, secotanapartholide A, canin and artecanin (Groenewegen et al. 1986); guaianolides, tanaparthin-α-peroxide, canin and secotanapartholide-A [major group] and the corresponding ‘β’ series [β-peroxide, artecanin and the seco-B derivative] (Begley et al. 1989); significant amounts of parthenolide, camphor and chrysanthenol acetate (Smith and Burford 1992); guaianolide 3,4-epoxy-8-deoxycumambrin B and the eudesmanolide epoxysantamarine (Milbrodt et al. 1997); flavonoids, 3,6,4′-trimethyl ether and quercetagetin 3,6,3′-trimethyl ether, methyl ethers of scutellarein and 6-hydroxyluteolin, chrysoeriol 7-glucuronide, quercetin 7-glucuronide and apigenin and luteolin 7-glucuronides (Williams et al. 1999a); (2-glyceryl)-O-coniferaldehyde as the major constituent of T. parthenium tissue culture (Laiking and Brown 1999); and flavonoids santin 3, jaceidin 2 and centaureidin 1 (Long et al. 2003). The lipophilic flavonol, 6-hydroxykaempferol 3,7,4′-trimethyl ether, called tanetin, was found in the leaf, flower and seed of feverfew together with the known 6-hydroxykaempferol 3,7-dimethyl ether, quercetagetin 3,7-dimethyl ether and quercetagetin 3,7,3′-trimethyl ether (Williams et al. 1995). The lipophilic flavonoids in leaf and flower were found to be methyl ethers of the flavonols 6-hydroxykaempferol and quercetagetin (Williams et al. 1999b). Apigenin and two flavone glucuronides were present in glandular trichomes on the lower epidermis of the ray florets. The structures of the four 6-hydroxyflavonol methyl ethers of T. parthenium were found to be 6- rather than 7-O-methylated, and tanetin was revised as 6-hydroxykaempferol 3,6,4′-trimethyl ether. The vacuolar flavonoids of feverfew were dominated by the presence of apigenin and luteolin 7-glucuronides; nine other glycosides were also present. Some rare compounds found in feverfew included guaianolides, 8α-hydroxystafiatin, endoperoxide tanaparthin-α-peroxide, canin, 10-epi-canin and secotanapartholide A and B (Todorova and Evstatieva 2001).
Three flavones, 3-hydroxyflavone, kaempferol (3,4′,5,7-tetrahydroxyflavone) and fisetin (3,3′,4′,7-tetrahydroxyflavone), and a flavanone naringenin (4′,5′,7-trihydroxyflavanone) were isolated from the aerial parts (Shafaghat and Salimi 2008).
Antioxidant phenolic acid compounds 3,5-, 4,5- and 3,4-di-O-caffeoylquinic acids (DCQAs) were purified from feverfew (Wu et al. 2007). Polyphenolic compounds (g/kg dry matter) in the aerial feverfew plant parts were determined as follows: chlorogenic acid 6.45 g, 3,5-DCQA (dicaffeoylquinic acid) 30.08 g, 4,5, DCQA 5.61 g, total caffeoyl derivatives 42.14 g, total dihydroxycinnamic acid derivatives 57.21 g, total flavonoids 13.97 g, total dihydroxycinnamic acid derivatives + flavonoids 71.18 g and total polyphenolic compounds 81.12 g (Fraisse et al. 2011).
Feverfew essential oil was found to increase from the beginning of stem formation (0.30 %, v/w) until full bloom (0.83 %, v/w) (Hendriks et al. 1996). During the development of the plant, the content of camphor rose from 28 to 48 %, whereas the amount of chrysanthenyl acetate decreased from 30 to 22 %. In none of the oil samples investigated could the potentially toxic monoterpenes α- or β-thujone be detected. They found that the yield of parthenolide per individual plant gradually increased from about 10 mg at study commencement to about 20 mg when the plant was in full bloom (Hendriks et al. 1997). Parthenolide was present in the leaves and flower heads, but not in the stems. Drying at ambient temperature and lyophilization had no negative influence on the yield of parthenolide per individual plant.
Twenty components were identified in the essential oil of aerial parts of T. parthenium in the eastern region of Kosova, constituting 885 of the oil (Haziri et al. 2009). The major components were camphor 63 % and camphene 9.6 %, indicating the occurrence of camphor/camphene chemotype in Kosova. Other components included p-cymene 3.3 %, bornyl acetate 3 %, chrysanthenone 1.3 % and the remainder all <1 %: tricylene, α-thujene, α-pinene, β-pinene, α-phellandrene, α-terpinene, pinocarvone, borneol, terpinene-4-ol, p-cymen-8-ol, α-terpineol, myrtenal, eugenol, trans-myrtenolacetate, isobornyl-2-methylbutanoate and caryophyllene oxide. The volatile fraction of the essential oil of feverfew grown in Belgium was characterized by high camphor (44 %) and trans-chrysanthenyl acetate (23 %) contents (de Pooter et al. 1989).
Twenty-three components were identified in the essential oil of T. parthenium aerial plant parts from Turkey representing 90.1 % of the oil (Akpulat et al. 2005). The main constituents were camphor (56.9 %), camphene (12.7 %), p-cymene (5.2 %) and bornyl acetate (4.6 %). Other components identified include tricylene, α-thujene, α-pinene, β-pinene, α-phellandrene, α-terpinene, γ-terpinene, chrysantheone, pinocarvone, borneol, terpinen-4-ol, ρ-cymen-8-ol, α-terpineol, myrtenal, carvacrol, eugenol, trans-myrtenol acetate, isobornyl 2-methyl butanoate and caryophyllene oxide. Twenty-nine components were identified in feverfew essential oil from Iran (Mohsenzadeh et al. 2011). The highest yield was extracted at the flowering stage, and the major components were camphor (18.94 %), bornyl acetate (18.35 %), camphene (13.74 %), bornyl isovalerate (3.15 %), borneol (10.93 %), juniper camphor (6.23 %) and β-eudesmol (2.65 %).
Tissue cultures of T. parthenium afforded significant amounts of two coumarins, scopoletin and isofraxidin together with stigmasterol and sitosterol (Banthorpe and Brown 1989). These two coumarins were either absent or were very minor compounds in the parent plant.
Leaf Phytochemicals
Water-soluble flavone glycosides detected in the leaves were identified as apigenin 7-glucuronide, luteolin 7-glucuronide, luteolin 7-glucoside and chrysoeriol 7-glucuronide (Williams et al. 1995). Murch et al. (1997) reported minute amount up to 2.45 μg/g of melatonin (N-acetyl-5-methoxytryptamine) in the leaves which was lower than that found in the flowers of St. John’s wort (4.39 μg/g) and Scutellaria baicalensis (7.11 μg/g). The following flavonoids were detected in feverfew leaves: flavones, luteolin, scutellarein-6-methyl ether (hispidulin), scutellarein-6-4′-dimethyl ether (pectolinarigenin), 6-hydroxyluteolin 6-methyl ether (nepetin), 6-hydroxyluteolin 6,3′ -dimethyl ether (jaceosidin); flavonol, quercetagetin 3,6-dimethoxy ether (axillarin) (Ivancheva et al. 1998).
Parthenolide, tanaparthin-α-peroxide, tanaparthin-β-peroxide, 3-β-hydroxycostunolide and canin (Kaplan et al. 2002); parthenolide and epoxyartemorin (Sumner et al. 1992) were isolated from the leaves. Leaves of field-grown plants had higher concentration of parthenolide (1.85 % w/w dw) than those from hydroponic plants (0.38 % w/w dw) (Pedneault et al. 2002). From the leaves, isolated sesquiterpene lactones were identified as parthenolide, epoxysantamarin, 3β-hydroxyparthenolide and secotanapartholide A, and flavonoids identified as santin, apigenin, luteolin and quercetin (Rateb et al. 2007). Forty compounds were identified in the leaf essential oil, and the major compounds were camphor 37.7 %, chrysanthenyl acetate 33.8 % and terpin-1-ol 5.14 % (Rateb et al. 2007). Other minor components included butyl acetate, tricylene, α-pinene, camphene, sabinene, β-pinene, β-myrcene, p-cymene, limonene, β-phellandrene, 1,8-cineole, γ-terpinene, terpinolene, p-cymenene, pinocarvone, borneol, terpin-4-ol, α-terpineol, linalool acetate, bornyl acetate, citronellal hydrate, thymol, E-pinocarvyl acetate, carvacrol, α-copaene, E-caryophyllene, α-humulene, E-β-farnesene, germacrene D, Ar-curcumene, isobornyl-2-methyl butyrate, Σ-cadinene, Z-chrysanthenyl angelate, bornyl angelate, β-caryophyllene oxide, globulol, viridiflorol, E,E-farnesol and E,E,-farnesyl acetate. Shafaghat et al. (2009) found that feverfew leaf oil was characterized by a high amount of camphor (56.4 %), whereas in the stem oil, camphor (26.0 %), trans-β-ocimene (23.6 %) and germacrene-D (15.0 %) were the major constituents.
Root Phytochemicals
The roots of Tanacetum parthenium transformed with Agrobacterium rhizogenes afforded, in addition to the known coumarin isofraxidin, a new isofraxidin drimenyl ether which was elucidated as 9-epipectachol B (Kisiel and Stojakowska 1997). Twenty components (92 % of essential oil) were identified in the essential oil of T. parthenium roots and rhizomes (Mojab et al. 2007). Camphor (30.2 %), (Z)-chrysanthenyl acetate (26.5 %), α-farnesene (11.1 %) and spathulenol (8.2 %) were the major components. Other components included bornyl angelate (3.3 %), bornyl acetate (3.1 %), ethyl myristate (1.8 %), fenchone (1.5 %),1-hexanol (1.7 %), 7-benzofuranol (1.3 %), hexanal (1.1 %), β-myrcene (0.8 %), β-caryophyllene (0.4 %), α-pinene (0.3 %), p-cymene (0.3 %), carvacrol (0.2 %), borneol (0.1 %), isobornyl formate (0.1 %), isothujyl acetate (0.1 %) and β-bisabolene (0.1 %). The main components of the root oil were α-pinene (50.0 %), trans-β-farnesene (13.8 %) and bicyclogermacrene (11.0 %) (Shafaghat et al. 2009).
In feverfew hairy root cultures treated with methyl jasmonate (MJ) increased accumulation of spiroketal enol ether type diacetylenes of known deterrent activity about twofolds (Stojakowska et al. 2002). Salicylic acid (SA) transiently reduced a content of constitutive spiroketal enol ethers, common for all root clones, but selectively enhanced accumulation of cis-C13-spiroketal enol ether epoxide ((E)-3,4-epoxy-2-(2,4-hexadiynylidene)-1,6-dioxaspiro[4.4]nonane) (2), present in clone M2. The maximum total acetylene content (≈2.5 and 1.1 % dry weight in clones M2 and I2, respectively) was observed after 72–96 hours MJ treatment. Simultaneous addition of SA and MJ to the culture medium increased accumulation of the epoxide in clone M2 (up to sixfold increase compared with the control).
Chemicals in Feverfew Extracts and Products
In the European Patent Specification EP 1 367 993 B1 titled ‘Use of a feverfew extract for regulating skin ageing factors’, Martin and Salio (2002) the inventors stated that to-date, the chemical constituents of whole feverfew extract include, but are not limited to apigenin-7-glucoside, apigenin-7-glucuronide, 1-β-hydroxyarbusculin, 6-hydroxykaempferol-3,7-4′-trimethylether (tanetin), 6-hydroxykaempferol-3,7-dimethyl ether, 8-β-reynosin, 10-epicanin, ascorbic acid, β-carotene, calcium, chromium, chrysanthemolide, cosmosine, chrysanthemum, chrysarten-A, chrysarten-C, chrysoeriol-7-glucuronide, cobalt, β-costunolide, epoxyartemorin, luteolin-7-glucoside, luteolin-7-glucuronide, mangnoliolide, tanaparthin, parthenolide, reynosin, quercetagentin-3,7,3′-trimethylether, quercetagetin-3′7- dimethylether, 3,7,3′-trimethoxyquercetagetin, tanaparthin-1α,4α-epoxide and tanaparthin-1β,4β-epoxide3-β-hydroxyparthenolide. In the US Patent US 6224875 B1, entitled ‘Tanacetum parthenium extract and method of obtaining same’, the inventors Bombardelli and Morazzoni (2001) stated that Tanacetum parthenium contained various volatile oils having mono- and/or sesquiterpene components, flavonoids, tannins and pyrethrin, as well as terpenoids of the family of sesquiterpene lactones known as germacranolides, guaianolides and eudesmanolides. These latter compounds were found to be characterized by an α-unsaturated γ-lactone structure and to comprise in particular the compounds known as parthenolide, 3-β-hydroxy-parthenoide, costunolide, 3-β-hydroxy-costunolide, artemorin, 8-α-hydroxy-estafiatin and chrysanthemum. The presence of these sesquiterpene lactones had been considered necessary for the extracts to achieve pharmacological activity in commercial and authenticated feverfew products by (Heptinstall et al. 1992). Heptinsatll et al. (1992) found authenticated Tanacetum parthenium grown in the United Kingdom contained a high level of parthenolide in leaves, flowering tops and seeds but a low level in stalks and roots; the level of parthenolide in powdered leaf material declined during storage and purported feverfew products varied widely in their parthenolide content and in some products parthenolide was not detected.
Antioxidant Activity
Major polyphenolic acid compounds in feverfew with potent DPPH* scavenging activities were characterized as 3,5-, 4,5- and 3,4-di-O-caffeoylquinic acids (DCQAs) (Wu et al. 2007). Total antioxidant capacity (%) (DPPH scavenging activity) of feverfew aerial plant parts was 7.89 %, and contribution from the main caffeoyl derivatives was as follows: chlorogenic acid 8.49 %, 3,5- DCQA (dicaffeoylquinic acid) 48.92 %, 4,5-DCQA 9.12 % and total caffeoyl derivatives 66.53 % (Fraisse et al. 2011).
A parthenolide-depleted extract of feverfew (PD-Feverfew), which was free of sensitization potential, was found to possess free radical scavenging activity against a wide range of reactive oxygen species and with greater activity than vitamin C (Martin et al. 2008). PD-Feverfew had a fivefold greater scavenging activity for oxygen and hydroxyl radicals than ascorbic acid and threefold greater scavenging activity for ferric radicals than ascorbic acid. PD-Feverfew had the greatest scavenging activity against ferric radicals followed by oxygen, hydroxyl and peroxynitrate radicals, respectively. PD-Feverfew was also effective in scavenging superoxide anion as measured by using a superoxide dismutase (SOD) assay kit. The IC50 of SOD scavenging activity for PD-Feverfew was 2.74 μg/ml and that for ascorbic acid was 34.8 μg/ml. Thus, the SOD activity of PD-Feverfew was 13-fold greater than that of ascorbic acid. In-vitro, PD-Feverfew restored cigarette smoke-mediated depletion of cellular thiols, attenuated the formation of UV-induced hydrogen peroxide and reduced proinflammatory cytokine release. PD-Feverfew was also found to protect keratinocytes from cigarette smoke-induced ROS formation. Cellular toxicity as measured by lactate dehydrogenase (LDH) release was also dose-dependently reversed by PD-Feverfew treatment. In-vivo, topical PD-Feverfew reduced UV-induced epidermal hyperplasia, DNA damage and apoptosis. In a clinical study PD-Feverfew treatment significantly reduced erythema versus placebo 24 hours post-UV exposure. The study showed that parthenolide-depleted extract of feverfew may protect skin from the numerous external aggressions encountered daily by the skin and reduce the damage to oxidatively challenged skin.
Anticancer/Cytotoxicity Activity
In-Vitro Studies
Parthenolide, a bioactive constituent from feverfew, induced mitochondrial dysfunction and apoptosis of human colorectal cancer cell line COLO205 (Zhang et al. 2004b). This action was found to be mediated by proapoptotic Bcl-2 family members relaying the cell death signalling elicited by parthenolide from caspase 8 to downstream effector caspases such as caspase 3 and eventually to cell death. It was also demonstrated that intracellular thiols and calcium equilibrium played a critical role in parthenolide-induced apoptotic cell death (Zhang et al. 2004a). Parthenolide rapidly depleted intracellular thiols, including both free glutathione (GSH) and protein thiols and concomitantly increased intracellular reactive oxygen species (ROS) and calcium levels in a dose- and time-dependent manner.
The alcoholic feverfew flower extract and parthenolide were the most potent, showing 100 % inhibition of Ehrlich ascites carcinoma cell viability at their highest concentration, but the alcoholic flower extract was slightly more potent than parthenolide at lower concentrations (Rateb et al. 2007). The aqueous leaf and flower extracts showed little in-vitro cytotoxic activity (60 and 65 %), respectively, at their high concentration. In an earlier study, at concentrations above 5.0 μM and an exposure time of 24 hours, parthenolide from feverfew inhibited cell growth of mouse fibrosarcoma (MN-11) and human lymphoma (TK6) cell lines in an irreversible fashion (Ross et al. 1999). However, at lower concentrations, i.e. 2.5 μM, the effect was reversible; parthenolide acted in a cytostatic fashion over multiple cell generations for both cell lines. After 24 hours exposure to 2.5 μM parthenolide, approx. 85 % of cells were able to continue cell cycling on removal of the chemical. The mechanism of the reversible growth inhibition was uncertain.
Parthenolide, from feverfew, was shown too preferentially induce acute myelogenous leukaemia stem cells to undergo apoptosis and importantly displayed no discernable effect on normal blood cells (Guzman and Jordan 2005). Anderson and Bejcek (2008) demonstrated that treatment of glioblastoma cells (brain tumour cells) with parthenolide resulted in rapid apoptosis through caspase 3/7 without a suppression of NF-kappaB activity. Studies showed parthenolide to be a promising metabolic inhibitor to retard tumorigenesis and to suppress tumour growth of cancer cells without harming normal cells (Pajak et al. 2008; Mathema et al. 2012). Parthenolide was shown to inhibit NF-kappaB- and STATs-mediated antiapoptotic gene transcription and amplified the apoptotic signal through the sensitization of cancer cells to extrinsic apoptosis, induced by TNF-alpha (Pajak et al. 2008; Mathema et al. 2012).
Kang et al. (2001) found that parthenolide potently inhibited the lipopolysaccharide (LPS)-induced interleukin IL-12 production in mouse macrophages in a dose-dependent manner. Parthenolide decreased significantly the binding activity to the kappaB site in the macrophage. In further studies they (Kang et al. 2002) found that parthenolide strongly potentiated 1 alpha,25-dihydroxyvitamin D(3)-induced differentiation of human leukaemia HL-60 cells into monocytes via the inhibition of NF-kappa B activity and provided evidence that inhibition of NF-kappa B activation could be a prerequisite to the efficient entry of promyelocytic leukaemia cells into a differentiation pathway. In another study, parthenolide, from feverfew, induced apoptosis in pre-B acute lymphoblastic leukaemia (ALL) lines, including cells carrying the t(4;11)(q21;q23) chromosomal translocation (Zunino et al. 2007). Parthenolide induced rapid apoptotic cell death distinguished by loss of nuclear DNA, externalization of cell membrane phosphatidylserine and depolarization of mitochondrial membranes at concentrations ranging from 5 to 100 μM and production of reactive oxygen species (ROS)-specific dyes, such as nitric oxide and superoxide anion. Parthenolide-induced elevation of hypochlorite anion was observed only in the two t(4;11) lines. The data suggested parthenolide may have potential as a potent and novel therapeutic agent against pre-B ALLs. In subsequent studies, they showed that parthenolide treatment activated stress-signalling proteins in high-risk acute lymphoblastic leukaemia cells with chromosomal translocation t(4;11) (Zunino et al. 2010). Parthenolide was shown to be a potent anti-multiple myeloma cancer stem cell (MM-CSC) agent (Gunn et al. 2011). It demonstrated preferential toxicity towards MM-CSCs over non-tumorigenic MM cells. In a review of parthenolide Mathema et al. (2012) reported that parthenolide induced reactive oxygen species (ROS) exclusively in tumour cells leading to proapoptotic action; parthenolide actively interfered with microtubule formation by reducing impaired control of spindle positioning, a factor favouring tumour invasiveness in cancer cells and parthenolide-mediated STAT proteins, transcriptional factors responding to extracellular ligands that broadly mediate diverse biological functions such as cell proliferation, differentiation, transformation and apoptosis.
Parthenolide was found to inhibit proliferation of metastatic human lung cancer subline, PGCL3 cells, after 24 hours treatment the IC50 value was 17.60 μmol/l (Zhong et al. 2005). Parthenolide reduced significantly the activity of urokinase-type plasminogen activator secreted by PGCL3 cells and downregulated the expression level of urokinase-type plasminogen activator protein. The authors concluded that the antitumour activity of parthenolide involved the activity and expression of urokinase-type plasminogen activator. Parthenolide inhibited proliferation of human lung carcinoma (A549), human medulloblastoma (TE671), human colon adenocarcinoma (HT-29) and human umbilical vein endothelial cells (HUVEC) in-vitro with the following IC50 values: 4.3, 6.5, 7.0 and 2.8 μM, respectively (Parada-Turska et al. 2007). In another study, parthenolide inhibited proliferation of rabbit synoviocytes cell line HIG-82 and human rheumatoid arthritis fibroblast-like synoviocytes (RA-FLS) in-vitro (Parada-Turska et al. 2008). The proliferation of human skin fibroblasts (HSF) was inhibited less effectively.
Parthenolide exerted in-vitro stimulatory activity on tubulin assembly by inducing the formation of well-organized microtubule polymers in human breast cancer MCF-7 cells (Miglietta et al. 2004). It was found that parthenolide-induced alterations of microtubule network and nuclear morphology occurred only after combined exposures to paclitaxel. Further, the growth of MCF-7 cells was significantly inhibited by parthenolide, which enhanced paclitaxel effectiveness. The authors concluded that the antimicrotubular and antiproliferative effects of parthenolide, well-known microtubule-stabilizing anticancer agent, may influence paclitaxel activity and may be utilized in developing new combinational anticancer strategies. In separate earlier studies, Patel et al. (2000) found that parthenolide mimicked the effects of IkappaBalpha by inhibiting NF-kappaB DNA binding activity and manganese superoxide dismutase (Mn-SOD) expression and increasing paclitaxel-induced apoptosis of breast cancer cells. In other studies, feverfew ethanol extract inhibited the growth of two human breast cancer cell lines (Hs605T and MCF-7) and one human cervical cancer cell line (SiHa) with a half-effective concentration (EC50) of 1.5 mg/ml against Hs605T, 2.1 mg/ml against MCF-7 and 0.6 mg/ml against SiHa (Wu et al. 2006). Among the tested constituents of feverfew (i.e. parthenolide, camphor, luteolin and apigenin), parthenolide showed the highest inhibitory effect with an EC50 against Hs605T, MCF-7 and SiHa of 2.6, 2.8 and 2.7 μg/ml, respectively. Also it was found that apigenin and luteolin might have moderate to weak synergistic effects with parthenolide on the inhibition of cancer cell growth of Hs605T, MCF-7 and SiHa. In a more recent study, parthenolide and a synthetic compound, 3-isopropyl-2-methyl-4-methyleneisoxazolidin-5-one, with the same α-methylene-γ-lactone motif as in parthenolide, were found to reduce the number of viable breast cancer MCF-7 and MDA-MB-231 cells, with half maximal inhibitory concentration values between 6 and 9 μM (Wyrębska et al. 2013). Both compounds dose-dependently inhibited incorporation of [(3)H]thymidine, upregulated Bax and downregulated Bcl-2 mRNA. In MCF-7 cells, MZ-6 induced early apoptosis and cell cycle arrest in G0/G1 phase, and its effect was much stronger compared with parthenolide. In MDA-MB-231 cells, both tested compounds had similar effect and induced mostly late apoptosis.
Treatment of sesquiterpene lactones isolated from Calea urticifolia and Tanacetum parthenium at a low concentration (1 μM) significantly blocked 3-isobutyl-1-methylxanthin (IBMX)-induced melanogenesis, but did not induce the inhibitory activity of B16 melanoma cell growth (Ohguchi et al. 2009). In another study, parthenolide reduced the number of viable adherent cells in melanoma cultures with an MIC value of 4 μmol/l (Lesiak et al. 2010). Melanoma cell death accompanied by mitochondrial membrane depolarization and caspase-3 activation was observed as the result of parthenolide application. Reactive oxygen species level was not significantly increased in melanoma cells. However, preincubation of parthenolide with the thiol nucleophile N-acetyl-cysteine protected melanoma cells from parthenolide-induced cell death, suggesting the reaction with intracellular thiols as the mechanism responsible for parthenolide activity. Thus, parthenolide could have potential as a drug candidate for further testing in melanoma therapy.
Treatment of bladder cancer cells with parthenolide resulted in a significant decrease in cell viability (Cheng and Xie 2011). Parthenolide induced apoptosis through the modulation of Bcl-2 family proteins and poly (ADP-ribose) polymerase degradation. Treatment with parthenolide led to G1 phase cell cycle arrest in 5637 cells by modulation of cyclin D1 and phosphorylated cyclin-dependent kinase 2. Parthenolide also inhibited the invasive ability of bladder cancer cells.
Animal Studies
Mice fed with parthenolide (1 mg/day) showed a delayed onset of skin papilloma incidence induced by UVB, a significant reduction in papilloma multiplicity (papilloma/mouse) and sizes when compared with the UVB-only group (Won et al. 2004). It was found that noncytotoxic concentrations of parthenolide inhibited UVB-induced activator protein-1 DNA binding and transcriptional activity. In addition, parthenolide pretreatment also inhibited c-Jun-N-terminal kinase (JNK) and p38 kinase activation leading to the sensitization of JB6 cells to UVB-induced apoptosis. In subsequent studies, they demonstrated that parthenolide sensitized UVB-induced apoptosis via protein kinase C (PKC) pathways (Won et al. 2005). Parthenolide pretreatment of JB6 murine epidermal cells enhanced membrane translocation of PKCdelta, but inhibited the translocation of PKCzeta. Further, pretreatment with a specific PKC delta inhibitor, rotterlin, completely diminished the sensitization effect of parthenolide on UVB-induced apoptosis. Similarly when cells were transiently transfected with dominant negative PKCdelta or wild-type PKCzeta, the sensitization effect of parthenolide on UVB-induced apoptosis was also drastically reduced.
Antiviral Activity
Feverfew was one of 32 plant herbs that exhibited inhibitory activity against Epstein–Barr virus early antigen (EBV-EA) activation in Raji cells promoted by phorbol ester, 12-O-tetradecanoylphorbol-13-acetate (TPA) (Kapadia et al. 2002). Studies showed that parthenolide inhibited the growth of the Epstein–Barr virus (EBV)-positive Burkitt lymphoma cell line, Raji, and activated the transcription of BZLF1 and BRLF1 by inhibiting NF-κB activity (Li et al. 2012). Further, when parthenolide was used in combination with ganciclovir (GCV), the cytotoxic effect of parthenolide was amplified, suggesting that the induction of lytic EBV infection with parthenolide in combination with GCV may be a viral-targeted therapy for EBV-associated Burkitt lymphoma.
Neurological/Migraine Prophylactic Activity
The exact mechanism of migraine pathophysiology is still uncertain. Based on therapeutic and triggered migraine studies, serotonin receptors, nitric oxide, calcitonin gene-related peptide, pituitary adenylate cyclase-activating polypeptide and prostanoids have been demonstrated to be specific chemical mediators of migraine Charles (2013). Numerous mechanisms have been proposed for the action of feverfew in migraine and include inhibition of inflammation, inhibition of serotonin release, inhibition of prostaglandin synthesis, inhibition of platelet aggregation and secretion, inhibition of polymorphonuclear leucocyte degranulation, inhibition of phagocytosis of human neutrophils, inhibition of mast cell release of histamine minimizing damage to endothelium and inhibition of VSMC and modulating vasoconstriction (Biggs et al. 1992; Kemper 1999; Colodny et al. 2003). According to Rodriguez-Sainz et al. (2013) migraine, epilepsy and stroke are highly prevalent neurological disorders, often comorbid. They share diverse pathophysiological mechanisms that explain the use of similar drugs on certain occasions.
In-Vitro Studies
Sesquiterpene lactones, parthenolide, 3-β-hydroxyparthenolide, secotanapartholide A, canin and artecanin isolated from feverfew inhibited secretion of granular contents from platelets and neutrophils (Groenewegen et al. 1986). All the active compounds contained a α-methylene butyrolactone unit that could have been responsible for the antisecretory activity in extracts of feverfew. This may be relevant to the therapeutic value of feverfew in migraine and other conditions. T. parthenium samples established from seeds from 10 different regions were found to vary significantly within and between samples in migraine prophylactic activity in-vitro (Marles et al. 1992). Serotonin release inhibition from bovine platelets was found to correlate significantly with the content of germacranolide sesquiterpene lactone, parthenolide, although other sesquiterpene lactones from this plant and other Asteraceae species (including T. vulgare) were also shown to be active. Feverfew powder was found to be more potent than any of its extract or parthenolide alone in its antiserotonergic activity (Mittra et al. 2000). Feverfew extract potently and directly blocked 5-HT2B and 5-HT2A receptors and neuronally released 5-hydroxytryptamine (%-HT). Both parthenolide and Feverfew extract showed a time dependency in their action. The extract when degraded thermally up to 10 % could significantly block the 5-HT receptors and neuronal release of 5-HT; however, on further degradation it lost its inhibitory capacity markedly. Similar results were observed in rats fed orally with undergraded and degraded feverfew powder and injected i.p. with parthenolide. Feverfew powder was more effective than any of its extracts or pure parthenolide. Degraded feverfew extracts showed a marked decrease in their antiserotonergic activity.
The anti-migraine mechanism of feverfew supercritical extract was investigated in-vitro using the mouse macrophage cell line (RAW 264.7) by Aviram et al. (2012). Feverfew extract was found to inhibit both nitric oxide (NO) and TNF-α production in a dose-dependent manner with complete inhibition of NO occurring at 5 μg/ml. Feverfew inhibition of NO was attributed to the downregulation of both eNOS and iNOS enzymes at the translational and/or posttranslational level. Fukuda et al. (2000) demonstrated that parthenolide exerted potent inhibitory effects on the promoter activity of the iNOS gene in human monocyte cell line THP-1 cells in a dose-dependent manner. The increase in iNOS promoter-dependent reporter gene activity induced by the tumour-promoting phorbol ester, 12-O-tetradecanoylphorbol-13-acetate (TPA), was effectively suppressed by parthenolide, with an IC50 of 2 μM.
In cultured lipopolysaccharide (LPS)-stimulated BV-2 microglia pretreatment with parthenolide caused a dose-dependent reduction of interleukin-6 (IL-6) secretion; at 5 μm, the highest concentration tested, it also reduced the secretion of TNF-α (54 %) (Magni et al. 2012). The reduction of microglial activation by inhibition of proinflammatory agents may help attenuate the onset and intensity of acute migraine attacks. The in-vitro results provided an additional explanation for the efficacy of orally administered T. parthenium as an anti-migraine agent.
Crude chloroform extracts of fresh feverfew leaves (rich in sesquiterpene lactones) and of commercially available powdered leaves (lactone-free) produced dose-dependent inhibition of the generation of thromboxane B2 (TXB2) and leukotriene B4 (LTB4) by ionophore- and chemoattractant-stimulated rat peritoneal leucocytes and human polymorphonuclear leucocytes (Sumner et al. 1992). Approximate IC50 values were in the range of 5–50 μg/ml. Isolated lactones (parthenolide, epoxyartemorin) were also inhibitory, with approximate IC50 values in the range of 1–5 μg/ml. They concluded that feverfew contained a complex mixture of sesquiterpene lactone and non-sesquiterpene lactone inhibitors of eicosanoid synthesis of high potency and that these biochemical actions may be relevant to the claimed therapeutic actions of the herb for fever, arthritis and migraine. The major flavonol and flavone methyl ethers of both Tanacetum parthenium and T. vulgare variously inhibited the major pathways of arachidonate metabolism in leucocytes (Williams et al. 1999b). There were significant differences in potency, with the tansy 6-hydroxyflavones less active than the feverfew 6-hydroxyflavonols as inhibitors of cyclooxygenase and 5-lipoxygenase. An ethanol extract of T. parthenium aerial parts showed high affinity for the GABA(A)-benzodiazepine site, and the bioactive apigenin isolated may be responsible for CNS (central nervous system) effects of T. parthenium extracts which were also used for epilepsy and convulsions in Holland (Jäger et al. 2006, 2009).
Animal Studies
Tanacetum parthenium extract enriched in parthenolide significantly reduced nitroglycerin-induced Fos expression in the nucleus trigeminalis caudalis in an animal model of migraine (Tassorelli et al. 2005). Purified parthenolide inhibited nitroglycerin-induced neuronal activation in additional brain nuclei and, significantly, the activity of nuclear factor kappaB. The findings strongly suggested parthenolide to be the component responsible for the biological activity of Tanacetum parthenium as regards its anti-migraine effect.
Clinical Studies
In a double-blind, placebo-controlled trial wherein eight patients received capsules containing freeze-dried feverfew leaf powder and nine placebo, those who received placebo had a significant increase in the frequency and severity of headache, nausea and vomiting with the emergence of untoward effects during the early months of treatment (Johnson et al. 1985). The group given capsules of feverfew showed no changes in the frequency or severity of symptoms of migraine. The authors concluded that feverfew taken prophylactically prevented attacks of migraine. In another randomized, double-blind, placebo-controlled crossover study involving 72 volunteers with each treatment limb lasting 4 months before crossover, treatment with feverfew was associated with a reduction in the mean number and severity of migraine attacks in each 2-month period and in the degree of vomiting; duration of individual attacks was unaltered (Murphy et al. 1988). Visual analogue scores also indicated a significant improvement with feverfew. There were no serious side effects. In a double-blind placebo-controlled crossover trial of 57 migraine suffers, it was found that feverfew caused a significant reduction in pain intensity compared with the placebo treatment (Palevitch et al. 1997). Further, a marked reduction was recorded concerning the severity of the typical symptoms that were usually linked to migraine attacks, such as vomiting, nausea, sensitivity to noise and sensitivity to light. Transferring the feverfew-treated group to the placebo treatment resulted in an augmentation of the pain intensity as well as an increase in the severity of the linked symptoms. In contrast, shifting the placebo group to feverfew therapy resulted in a reduction of the pain intensity as well as in the severity of the linked symptoms. In another study of 24 patients (women 19–61 years old), administration of feverfew once daily (5 ml of the sap) for 30–60 days was found to significantly reduced migration index in 8 patients and less significantly in 5 patients, suggesting that feverfew may be beneficial in migraine prophylaxis as an additive drug (Prusiński et al. 1999).
In a randomized, double-blind, placebo-controlled, multicenter, parallel-group study involving 170 patients suffering from migraine, treatment of 89 patients with CO(2) extract of feverfew (MIG-99, 6.25 mg three times daily) and 81 with placebo showed that MIG-99 was effective and had a favourable benefit–risk ratio (Diener et al. 2005). The migraine frequency decreased from 4.76 by 1.9 attacks per month in the MIG-99 group and by 1.3 attacks in the placebo group. Logistic regression of responder rates showed an odds ratio of 3.4 in favour of MIG-99. Adverse events possibly related to study medication were 9/107 (8.4 %) with MIG-99 and 11/108 (10.2 %) with placebo (P = 0.654). Earlier in a double-blind, multicenter, randomized placebo-controlled dose–response study, they found that MIG-99 was effective only in a small predefined subgroup of patients with at least four attacks during the 28-day baseline period where the most favourable benefit–risk ratio was observed with a dosage of three capsules of 6.25 mg MIG-99 extract per day (Pfaffenrath et al. 2002). Because of the low number of patients, they asserted that the findings needed to be verified in a larger sample. In a prospective, open-label study in 12 patients diagnosed with migraine without aura, 12-week treatment with T. parthenium 300 mg plus Salix alba 300 mg (Mig-RL) twice daily was found to reduce the frequency of migraine attacks and their pain intensity and duration (Shrivastava et al. 2006). In a multicenter pilot study, 60 subjects treated 208 evaluable attacks of migraine over a 1-month period, 45 subjects treated 163 attacks with sublingual feverfew/ginger and 15 subjects treated 58 attacks with a sublingual placebo preparation (Cady et al. 2011). The study showed that sublingual feverfew/ginger appeared safe and effective as a first-line abortive treatment for a population of migraineurs who frequently experience mild headache prior to the onset of moderate to severe headache. In a recent randomized study of 69 women volunteers with chronic migraine, conducted over 10 weeks, an improvement of quality of life and better analgesic effect was found with acupuncture combined with feverfew compared with acupuncture or feverfew alone (Ferro et al. 2012).
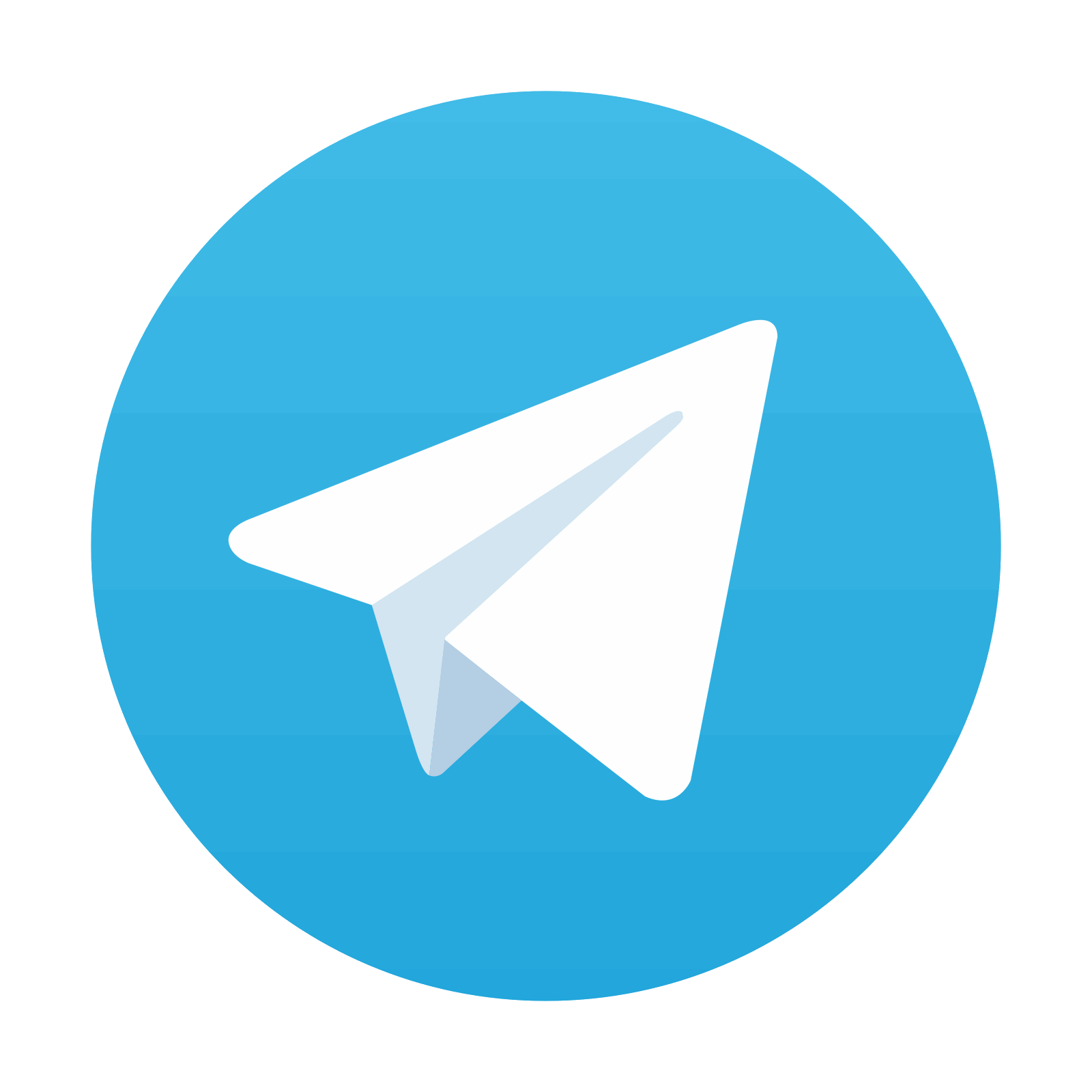
Stay updated, free articles. Join our Telegram channel

Full access? Get Clinical Tree
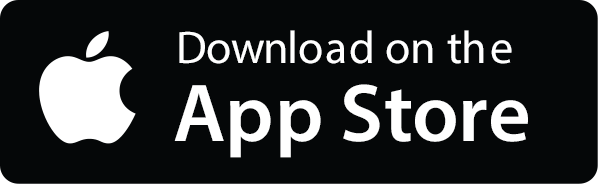
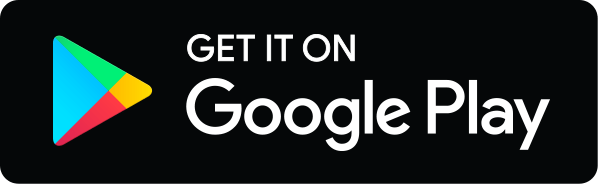
