Figure 52-1. A–D: Schematic drawings of the successive stages in the development of the pancreas from the fifth through the eighth weeks. E–G: Diagrammatic transverse sections through the duodenum and the developing pancreas. Growth and rotations (arrows) of the duodenum bring the ventral pancreatic bud toward the dorsal bud and they subsequently fuse. The bile duct initially attaches to the ventral aspect of the duodenum and is carried around to the dorsal aspect as the duodenum rotates. The main pancreatic duct is formed by the union of the distal part of the dorsal pancreatic duct and the entire ventral pancreatic duct.
The endocrine function of the pancreas begins during gestation, whereas the exocrine function does not begin until after birth. The first glucagon-producing cells are seen in 3-week-old embryos and the first islets appear at approximately 10 weeks. During this early developmental period, predominantly glucagon-positive islet cells initially appear in the tail of the pancreas. Early glucagon-positive endocrine cells convert to nonepithelial cells and lose connection with the lumen and tight junctions. Subsequently, there is a major amplification of endocrine cell numbers, particularly B cells, which produce insulin.
Figure 52-2. Anatomic configuration of the intrapancreatic ductal system. A: The classic anatomy is present in 60% of cases, where the accessory duct drains into the minor papilla and the main duct drains into the ampulla of Vater. B: The accessory pancreatic duct is blind and does not drain into the duodenum in 30% of cases. C: A lack of communication between the two ducts, which occurs in 10% of cases, is referred to as pancreas divisum. When this occurs the main pancreatic duct drains into the duodenum through the minor papilla. D: Pancreaticogram obtained on ERCP through cannulation of the minor papilla in a patient with pancreas divisum; the main duct drains into the minor papilla. E: MRCP on the same patient. The main pancreatic duct drains into the minor papilla and the common bile duct drains into the ampulla of Vater.
Surgical Significance of Congenital Pancreatic Abnormalities
4 Abnormalities in the rotation and fusion of the pancreas during embryonic development can result in specific congenital anomalies that have surgical significance. In approximately 60% of people, rotation and fusion occur normally resulting in the classic anatomy seen in Figure 52-2A. The dorsal and ventral ducts fuse to form the main pancreatic duct which drains the majority of the pancreas into the ampulla of Vater. The lesser duct, formed from the proximal duct of the dorsal bud, drains into the duodenum at the minor papilla.
In approximately 30% of cases, the ventral and dorsal ducts fuse and drain normally into the duodenum at the ampulla of Vater. However, there is atrophy of the accessory or minor duct with a blind end and drainage into the duodenum (Fig. 52-2B). As this blind duct still communicates with the main pancreatic duct, this is of little to no clinical significance and most often found only at autopsy.
In 5% to 14% of cases, the fusion of the ventral and dorsal pancreatic ducts is incomplete (Fig. 52-2C–E).1–3 As a result of the incomplete fusion, the majority of the pancreas is drained into the duodenum through the minor papilla. This is called pancreas divisum. Only the small remnant duct of the ventral bud drains the uncinate process into the duodenum via the ampulla of Vater. Whether or not pancreas divisum causes pancreatitis and abdominal pain is unclear.1,4,5 It is often asymptomatic. However, mucosal stenosis at the minor papilla may lead to cystic dilatation of the dorsal duct resulting in pancreatitis and pain.1,6–8 Pancreatitis or abdominal pain due to pancreas divisum is a diagnosis of exclusion, and other etiologies for the pancreatitis should be thoroughly investigated. If no other causes of pancreatitis are identified in the setting of abdominal pain, elevated amylase levels, and pancreas divisum, the anomaly is considered causative and an endoscopic or operative papillotomy of the minor papilla and accessory duct is indicated. Recurrent acute pancreatitis or chronic pancreatitis with chronic pain attributed to pancreas divisum is most often seen in young females.
Annular pancreas is a rare congenital anomaly of the pancreas first recognized in 1818. Early autopsy and surgical series estimate the incidence to be approximately 3 in 20,000.9,10 However, with better imaging modalities such as computed tomography (CT), magnetic resonance cholangiopancreatography (MRCP), and endoscopy, the incidence is thought to be closer to 1 in 1,000.11–13 Annular pancreas is thought to result from abnormal fusion of the ventral pancreatic bud to the duodenum, leading to improper rotation of the ventral bud around the duodenum.14 This failure of rotation leads to a thin band of normal pancreatic parenchyma completely surrounding the second portion of the duodenum (Fig. 52-3A–C). This band is in continuity with the head of the pancreas and causes variable degrees of duodenal compression and stenosis. This abnormal ring of pancreatic tissue may contain a pancreatic duct. Therefore, the surgeon must be aware of this anomaly; if annular pancreas is incidentally encountered during an operation, it should not be divided. Division of the abnormal ring can result in pancreatic fistula or obstruction of pancreatic ductal drainage.
Figure 52-3. Annular pancreas. A: CT scan of a patient with annular pancreas. There is a ring of pancreatic tissue surrounding the duodenum, with a narrowed duodenal lumen. B: EGD image in the same patient. Note narrowing of the duodenal lumen with no mucosal lesion consistent with external compression by the annular pancreas. C: Intraoperative photo of an annular pancreas. Note the dilated duodenum after the pylorus, the ring of pancreatic tissue surrounding the duodenum just distal to the dilation, and the decompressed distal duodenum.
Annular pancreas can present at varying time points from in utero to adulthood.13 When diagnosed in utero, the most common presentation is polyhydramnios due to duodenal obstruction. Newborns present most commonly with duodenal obstruction shortly after birth, as evidenced by low birth weight and feeding intolerance. In people who present in utero or in childhood, it is more commonly associated with other congenital anomalies including Down syndrome, cardiac anomalies, and other intestinal anomalies. Duodenal bypass (duodenoduodenostomy or gastrojejunostomy) is the treatment of choice in children.
Fifty percent of cases of annular pancreas occur in adults. Adults are less likely to present significant obstruction and less likely to require surgical intervention. If they do present with obstruction, treatment is similar to that in children. In adults, annular pancreas is more commonly associated with pancreas divisum and pancreatic neoplasia than in children.13 Heterotopic pancreas is pancreatic tissue outside the bounds of the normal pancreas without anatomic or vascular connections to the pancreas itself. Heterotopic pancreas occurs in 0.5% to 14% of autopsy series. The heterotopic pancreatic tissue is functional and can occur in a variety of sites including the stomach, duodenum, ileum, umbilicus, colon, appendix, gallbladder, and even within a Meckel diverticulum. This tissue is usually submucosal and uniformly contains acini and ducts. Up to one-third contains islet cells. Heterotopic pancreas is usually an incidental finding, but can present with ulceration, obstruction, or intussusception, in which case treatment is directed at the presenting symptoms and may require resection. In incidental and asymptomatic cases, no treatment is required. The heterotopic pancreas is susceptible to the same diseases as normal pancreas and can even undergo malignant transformation.15,16
EXOCRINE AND ENDOCRINE STRUCTURE
The exocrine structures of the pancreas account for 80% to 90% of the pancreatic mass while the endocrine structure accounts for approximately 2% of the pancreatic mass. The remainder of the pancreas comprises extracellular matrix, blood vessels, and major ductal structures.
Exocrine Structure
The exocrine structure of the pancreas is composed of two main components: the acinar cells and the ductal network. The acinar cells produce and secrete the enzymes and zymogens responsible for digestion. The acinar cells are pyramidal cells with an apex that faces the pancreatic ductal network. Approximately 20 to 40 acinar cells cluster together to form the functional unit called an acinus (Fig. 52-4A,B). Zymogen granules within the acinar cells contain the digestive enzymes for secretion into the ductal system. Located more centrally within the acinus, the centroacinar cell secretes alkaline fluid (pH 8.0) into the pancreatic ductal system. The acinus drains initially into small intercalated ducts, which join to form interlobular ducts that also secrete fluid and electrolytes (Fig. 52-4A). These interlobular ducts form secondary ducts that drain into the main pancreatic ductal system and eventually the duodenum at the ampulla of Vater.
Figure 52-4. Histologic anatomy of the acinus. A: Low-magnification view of a portion of the pancreas. B: High-magnification view of a single acinus. The acinar cells, containing zymogen granules, are pyramidal cells with an apex that faces the pancreatic ductal network. Twenty to 40 acinar cells that cluster together to form the functional unit called an acinus. The centroacinar cell, also present within the acinus, functions to secrete fluid and electrolytes of the correct pH into the pancreatic ductal system. The acinus drains into small intercalated ducts, which join to form interlobular ducts that also secrete fluid and electrolytes. These interlobular ducts form secondary ducts that drain into the main pancreatic duct.
Endocrine Structure
5 The pancreatic islet cells are of neural crest origin and part of the family of amine precursor uptake and decarboxylation (APUD) cells. Each pancreatic islet is approximately 40 to 900 mm and contains an average of 3,000 cells. The islets are composed of four cell types. These cell types are differentially distributed both throughout the pancreas and within the islets. Table 52-1 describes the cell types, their hormonal products, and their location within the islet and the pancreas.
B (or beta) cells are located centrally within the islets. They constitute approximately 70% of the islet cell mass. The main secretory product of B cells is insulin, but they also excrete amylin and cholecystokinin. A (or alpha) cells and F cells are located peripherally within the islets and constitute 10% and 15% of the islet cell mass, respectively. Glucagon is secreted by A cells, which is the major counter-regulatory hormone to insulin in glucose homeostasis. F cells secrete pancreatic polypeptide. D cells are evenly distributed throughout the islet and constitute 5% of the islet cell mass.17 They can be further divided into D cells which secrete somatostatin and D2 cells which secrete vasoactive intestinal peptide (VIP). E (or epsilon cells) and C cells comprise <1% of the islet mass and secrete substance P and serotonin. They are of minimal clinical significance.
6 The distribution of endocrine cell types is not uniform throughout the pancreas. B and D cells are concentrated in the tail of the pancreas, whereas A cells (as well as C and E cells) are evenly distributed and F cells are concentrated in the pancreatic head and uncinate process. As such, resection of the pancreatic body and tail removes more of the insulin-producing cells and is more likely to cause endocrine insufficiency (diabetes). This distribution also has clinical relevance with regard to the location of endocrine neoplasms when they occur.
The islet cells have a rich blood supply supporting their endocrine role. The afferent arteriole enters the islet in an area of discontinuity in the non-B cells surrounding the periphery. The afferent arteriole then breaks into a capillary bed within the islet. Blood exits the islet through an efferent collecting venule. The hormones from the islet cells are secreted directly into this rich capillary network within the islet.
7 The most critical role of the pancreatic islet cells is the secretion of insulin and glucagon to maintain glucose homeostasis. Pancreatic endocrine secretion also regulates pancreatic exocrine secretion. Insulin stimulates pancreatic exocrine secretion, amino acid transport, and synthesis of protein and enzymes, whereas glucagon acts in a counter-regulatory fashion, inhibiting the same processes. The role of somatostatin is controversial. Somatostatin may have a direct inhibitory effect on pancreatic acinar cells, which possess somatostatin receptors. It may also act through an inhibitory effect on islet B cells.
PANCREATIC PHYSIOLOGY
Exocrine Function
The pancreas secretes 1.5 to 3 L of a pancreatic fluid daily. The enzymes and zymogens play a major role in the digestive activity of the gastrointestinal tract. Pancreatic fluid is alkaline (pH 7.6 to 9.0) and carries over 20 proteolytic enzymes and zymogens to the duodenum. The enzymes are released into the duodenum in their inactive state; the fluid serves to neutralize gastric acid and provides an optimal milieu for the function of these enzymes.
Pancreatic secretion is regulated via an intimate interaction of both hormonal and neural pathways that integrate the function of the pancreas, biliary tract, and small intestine. Vagal (parasympathetic) afferent and efferent pathways strongly affect pancreatic secretion. The secretion of enzyme-rich fluid is largely dependent on the vagal stimulation, whereas fluid and electrolyte secretion are more dependent on the direct hormonal effects of the secretin and cholecystokinin (CCK). Parasympathetic stimulation also causes the release of VIP, which also serves to stimulate secretin secretion.18
Table 52-1 Pancreatic Endocrine Cell Types
Many neuropeptides also influence pancreatic secretion in an inhibitory fashion. These include somatostatin, pancreatic polypeptide, peptide YY, calcitonin gene–related peptides, neuropeptide Y, pancreastatin, enkephalin, glucagon, and galanin. While these neuropeptides are known to play a role in regulation of pancreatic secretion, the mechanisms of action and the intricate interplay between the neuropeptides is not fully understood.18
Bicarbonate Secretion
Bicarbonate is the most physiologically important ion secreted by the pancreas. Bicarbonate is formed from carbonic acid by the enzyme carbonic anhydrase. The secretion of water and electrolytes originates in the centroacinar and intercalated duct cells (Fig. 52-4). These cells secrete 20 mmol of bicarbonate per liter in the basal state and up to 150 mmol/L in the maximally stimulated state.18 The bicarbonate secreted from the ductal cells is primarily derived from the plasma. Chloride efflux through the cystic fibrosis transmembrane conductance regulator (CFTR) leads to depolarization and bicarbonate entry through the sodium bicarbonate cotransporter.18 As a result, chloride secretion varies inversely with bicarbonate secretion; the sum of these two anions balances the sodium and potassium cations and remaining constant and equal to that of the plasma.
Both secretin and VIP stimulate bicarbonate secretion by increasing intracellular cyclic AMP, which acts on the CFTR.18 Gastric acid is the primary stimulus for release of secretin. Secretin is released from the duodenal mucosa in response to a duodenal lumen pH of less than 3.0 due to gastric acid.
The duodenum and jejunum release CCK in response to the presence of long-chain fatty acids, some essential amino acids (methionine, valine, phenylalanine, and tryptophan), and gastric acid. CCK is weak direct stimulator of bicarbonate secretion, but it acts as a neuromodulator and potentiates the stimulatory effects of secretin. Gastrin and acetylcholine are also weak stimulators of bicarbonate secretion.19 Bicarbonate secretion is inhibited by atropine (vagal stimulation) and can be reduced by 50% after truncal vagotomy.20 Islet cell peptides including somatostatin, pancreatic polypeptide, glucagon, galanin, and pancreastatin are thought to inhibit exocrine secretion.
Enzyme Secretion
Pancreatic enzymes originate in the acinar cells, which are highly compartmentalized. Proteins are synthesized in the rough endoplasmic reticulum, processed in the Golgi apparatus, and then targeted to the appropriate cell compartment (zymogen granules, lysosomes, etc.). The acinar cells secrete enzymes that fall into three major enzyme groups: amylolytic enzymes, lipolytic enzymes, and proteolytic enzymes. Amylolytic enzymes such as amylase hydrolyze starch to oligosaccharides and the disaccharide maltose. Lipolytic enzymes such as lipase, phospholipase A, and cholesterol esterase function work in conjunction with bile salts to digest fats and cholesterol. Proteolytic enzymes include endopeptidases (trypsin and chymotrypsin) and exopeptidases (carboxypeptidase). Endopeptidases act on the internal peptide bonds of proteins and polypeptides and exopeptidases act on the free carboxy- and amino-terminal ends of proteins. Proteolytic enzymes are secreted as inactive precursors. Enterokinase cleaves the lysine–isoleucine bond in trypsinogen to create the active enzyme trypsin. Trypsin then activates the other proteolytic enzyme precursors.18
The different pancreatic enzymes are not secreted in fixed ratios. They change in response to dietary alterations and stimuli such as gastric acid, hormones, and neuropeptides. When enzyme secretion is absent or impaired, malabsorption or incomplete digestion occurs, leading to fat and protein loss through the gastrointestinal tract. This is seen in patients with acute and chronic pancreatitis (who have destruction of the exocrine pancreas) and in patients who have undergone surgical resection of all or part of the pancreas. These patients often present with weight loss and steatorrhea secondary to malabsorption of nutrients. These signs and symptoms can be corrected by oral replacement of pancreatic enzymes with meals.
The nervous system initiates pancreatic enzyme secretion. This involves extrinsic innervation by the vagus nerve and subsequent innervation by the intrapancreatic cholinergic fibers. The neurotransmitters, acetylcholine and gastrin-releasing peptide activate calcium-dependent release of zymogen granules.18 In addition, CCK is a predominant regulator of enzyme secretion, doing so through activation of specific membrane-bound receptors and calcium-dependent second messenger pathways. Secretin and VIP weakly stimulate acinar cell secretion directly, but also potentiate the effect of CCK on acinar cells (Fig. 52-5). Insulin is required locally and serves in a permissive role for secretin and CCK to promote exocrine secretion.18
Through the secretion of the three classes of enzymes, the pancreas regulates complete digestion of carbohydrates, fats, and proteins. Autodigestion of the pancreas by these proteolytic enzymes is prevented by packaging of proteases in an inactive precursor form and by the synthesis of protease inhibitors including pancreatic secretory trypsin inhibitor (PSTI), serine protease inhibitor, kazal type 1 (SPINK1), and protease serine 1 (PRSS1). These enzymes are found in the acinar cell and loss of these protective mechanisms can lead to activation, autodigestion, and acute pancreatitis. Mutations in the SPINK1 and PRSS1 genes are known to cause one of the aggressive familial forms of chronic pancreatitis, leading to recurrent episodes of pancreatitis, with associated exocrine and endocrine insufficiency.21,22
Figure 52-5. Schematic diagram of the acinar cell, demonstrating receptors for exocrine secretagogues and their intracellular bases of action. Six distinct classes of receptors are known, with principal ligands shown. CCK, cholecystokinin; VIP, vasoactive intestinal peptide; CRGP, calcitonin gene–related peptide; DAG, diacylglycerol.
Table 52-2 Characteristic Results of Secretin Testing: Volume, Bicarbonate Concentration, and Enzyme Secretion Changes in Pancreatic Disease Processes
Tests of Pancreatic Exocrine Function
8 Several tests are useful in the assessment of pancreatic exocrine function. Such tests are useful in both diagnosing and determining the etiology of exocrine insufficiency (chronic pancreatitis, malnutrition, cancer, and Zollinger–Ellison syndrome) (Table 52-2). Steatorrhea from pancreatic exocrine dysfunction is the result of lipase deficiency and is usually not present until lipase secretion is reduced by 90%. The secretin test, the dimethadione test (DMO) and the Lundh test require duodenal intubation. The classic test of pancreatic exocrine function is the secretin test.23 A patient fasts overnight. A double-lumen tube is then placed in the duodenum. Basal collections are performed for 20 minutes and analyzed for total volume, bicarbonate output, and enzyme secretion. An intravenous bolus of 2 units of secretin per kilogram is given and four collections every 20 minutes are analyzed for volume, bicarbonate levels, and enzyme levels.
Normal values for the standard secretin stimulation test are 2.0 mL of pancreatic fluid per kilogram per hour, bicarbonate concentration of 80 mmol/L, bicarbonate output of >10 mmol/L in 1 hour, and amylase secretion of 6 to 18 International Units/kg. The maximal bicarbonate concentration provides the greatest discrimination between normal subjects and patients with chronic pancreatitis.24 The results of the secretin stimulation test for different pancreatic disease processes is shown in Table 52-2.
The pancreas metabolizes the anticonvulsant drug trimethadione to its metabolite, DMO. After placing a double-lumen tube in the duodenum, patients are given 0.45 g of trimethadione three times daily for 3 days. Secretin is given through the double-lumen tube to maximally stimulate pancreatic secretion. To measure pancreatic exocrine function, the duodenal output of DMO is analyzed.25
The Lundh test directly measures pancreatic enzyme secretion in response to a meal of carbohydrate, fat, and protein. A patient fasts overnight, then has a double-lumen duodenal tube placed. After basal duodenal fluid collection, patients are given a meal consisting of 18 g of corn oil, 15 g of casein, and 40 g of glucose in 300 mL of water. Duodenal fluid is collected every 30 minutes for 2 hours and analyzed for trypsin, amylase, and lipase. This test relies on endogenous secretin and CCK secretion and may be abnormal in diseases involving the intestinal mucosa.
N-benzoyl-1-tyrosyl paraaminobenzoic acid (BT-PABA) is cleaved by chymotrypsin to form paraaminobenzoic acid (PABA), which is then excreted in the urine. The PABA test is performed by administering 1 g of BT-PABA in 300 mL of water orally. Urine is then collected for 6 hours. Patients with chronic pancreatitis excrete less than 60% of the ingested dose of PT-PABA.
Suspected pancreatic exocrine dysfunction can also be confirmed giving patients a test meal and measuring serum levels of the islet cell hormone pancreatic polypeptide (PP). Basal and meal-stimulated levels of serum PP are reduced in severe chronic pancreatitis and after extensive pancreatic resection. After an overnight fast, a test meal of 20% protein, 40% fat, and 40% carbohydrate is ingested. The normal basal range of PP is 100 to 250 pg/mL. In severe chronic pancreatitis, the basal levels are often less than 50 pg/mL. The normal response to a meal is a rise in PP levels to 700 to 1,000 pg/mL for 2 to 3 hours after the meal. In severe disease, this response is decreased to less than 250 pg/mL. PP release depends on intact pancreatic innervation and can also be decreased after truncal vagotomy, antrectomy, or in the setting of diabetic autonomic neuropathy.
The triolein breath test is a noninvasive test of pancreatic exocrine insufficiency or malabsorption, but does not differentiate between the two.26 25 g of 14C-labeled corn oil (triglycerides) are given to the patient orally. The metabolite, 14C-carbon dioxide, can be measured in the breath 4 hours after administration. Patients with disorders of fat digestion or malabsorption exhale less than 3% of the dose per hour. The test can be repeated after pancreatic enzyme replacement. Patients with pancreatic insufficiency will achieve a normal rate of excretion of 14C-carbon dioxide, whereas patients with enteric disorders (malabsorption) show no improvement.
Many tests can help differentiate between steatorrhea caused by pancreatic exocrine insufficiency versus malabsorption (Table 52-3). The secretin test, the PABA test, and PP will be normal in intestinal malabsorption and abnormal in pancreatic insufficiency. The fecal fat test measures intraluminal digestion products. Fecal fat content is measured over a 24-hour time period. If the fecal fat is elevated to more than 20 g this indicates pancreatic insufficiency, whereas steatorrhea in the presence of low levels of fecal fat (<20 g) indicates intestinal dysfunction. A reduction of fecal fat can be used to demonstrate adequate replacement of pancreatic enzymes in patients with exocrine insufficiency. However, this test is time consuming and disliked by patients and pancreatic enzyme replacement is often titrated based on symptom relief if the clinical situation leads to a high index of suspicion for pancreatic exocrine insufficiency (i.e., long-standing chronic pancreatitis) or once the diagnosis of pancreatic insufficiency is made.
Table 52-3 Differential Diagnosis of Intestinal and Pancreatic Steatorrhea
Serum amylase, lipase, and trypsinogen are not elevated in the physiologic state. As amylase is found in organs other than the pancreas (salivary glands, liver, small intestine, kidneys, and fallopian tubes), serum amylase levels may be elevated in both pancreatic and nonpancreatic diseases (Table 52-4). When pancreatic disease is suspected, the measurement of serum amylase and lipase levels serve as a useful screening test. Assays for the isoenzymes of amylase can be performed, but are unreliable in determining whether the source of amylase is pancreatic or nonpancreatic and are not widely used. It has been shown that serum lipase is a more accurate biomarker of acute pancreatitis than serum amylase, with 19% of patients with acute pancreatitis having normal serum amylase levels, but only 3% having normal serum lipase levels.27 Unlike amylase and lipase, serum trypsinogen is made only by the pancreas and may serve as a better marker for acute pancreatitis. Trypsinogen can also be measured in the urine and serves as a specific screening test for acute pancreatitis.28,29 All three markers are cleared by the kidneys so in the setting of acute renal failure, all may be falsely elevated. These levels do not measure actual endocrine function, but are commonly used to diagnose acute pancreatitis and monitor its resolution.
In the case of acute pancreatitis, serum amylase and lipase levels are usually elevated and peak within 24 hours of the onset of symptoms. They return to normal within 2 to 4 days if the inflammation resolves. In cases of severe necrotizing pancreatitis, pancreatic ductal obstruction, and pseudocyst formation, amylase and lipase levels can remain elevated for much longer periods of time. In this case, elevated levels often reflect amylase-rich fluid within peripancreatic collections and not ongoing acute inflammation.
While amylase and lipase levels are elevated in over 85% of patients with acute pancreatitis, their elevation is far less common in chronic pancreatitis, where the exocrine function of the pancreas may be impaired. Amylase levels may be normal in patients with acute pancreatitis if there is a delay in their diagnosis or if their pancreatitis is related to hypertriglyceridemia, which can falsely decrease the serum amylase levels.24
Endocrine Function
The primary function of the endocrine pancreas is regulation of carbohydrate metabolism, primarily through regulation of insulin and glucagon secretion through a variety of feedback and regulatory mechanisms. Insulin promotes glucose transport into cells, inhibits glycogenolysis and fatty acid breakdown, and stimulates protein synthesis. Glucagon is the major counter-regulatory hormone to insulin. Glucagon secretion leads to elevated blood glucose levels through stimulation of glycogenolysis and gluconeogenesis.
Insulin Synthesis, Secretion, and Action
Insulin is a 56-amino-acid polypeptide with a molecular weight of 6 kD. It consists of two polypeptide chains (A and B) joined by two disulfide bridges. Insulin is synthesized by the B cells within the islets of Langerhans. Insulin is an anabolic hormone, promoting glucose transport into all cells except B cells, hepatocytes, and central nervous system cells. It also inhibits glycogenolysis and fatty acid breakdown but stimulates protein synthesis.
Table 52-4 Pancreatic and Nonpancreatic Causes of Hyperamylasemia
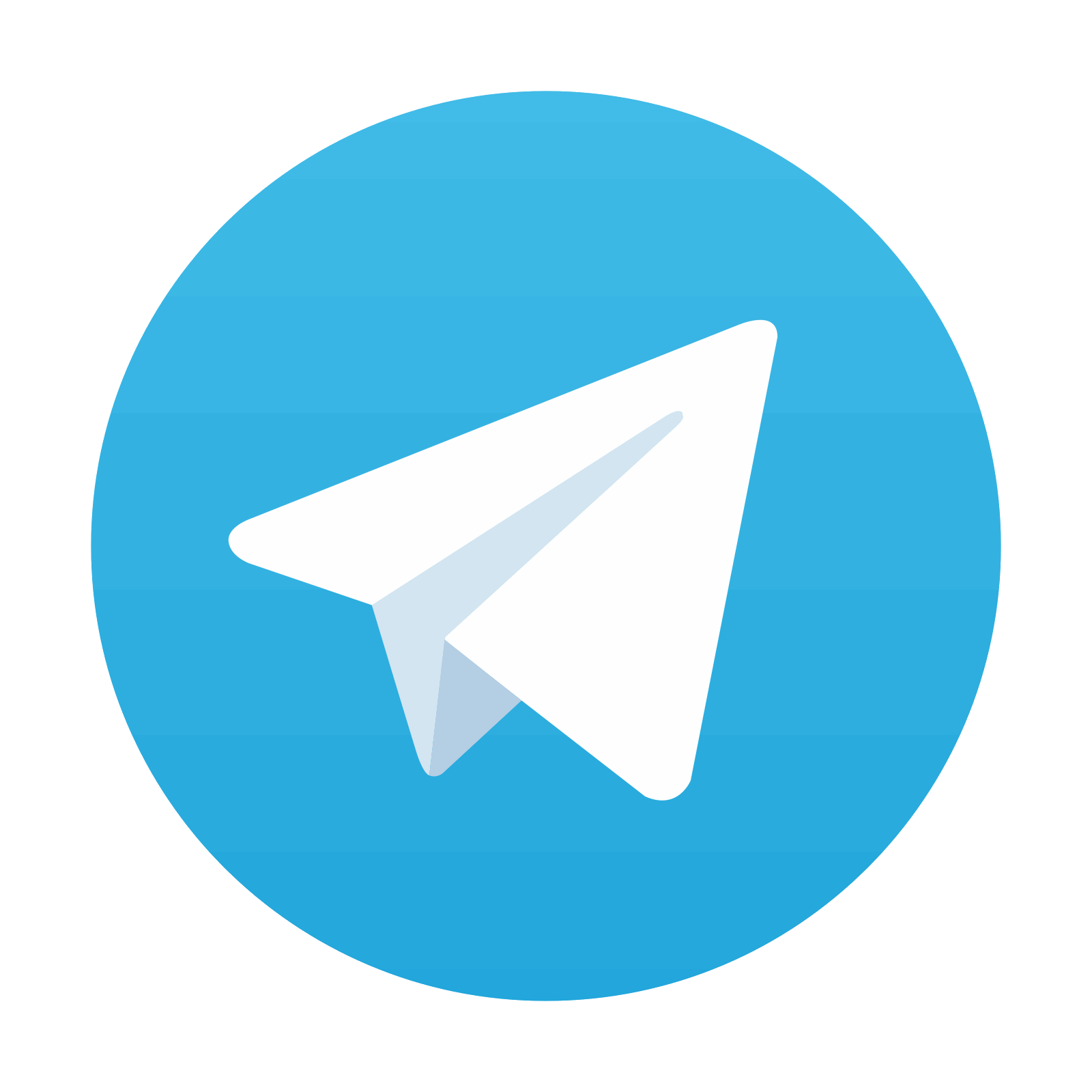
Stay updated, free articles. Join our Telegram channel

Full access? Get Clinical Tree
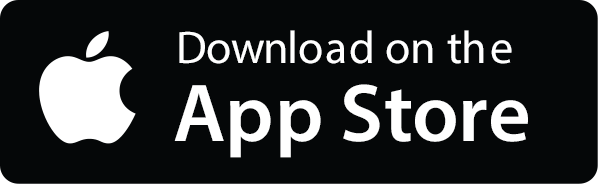
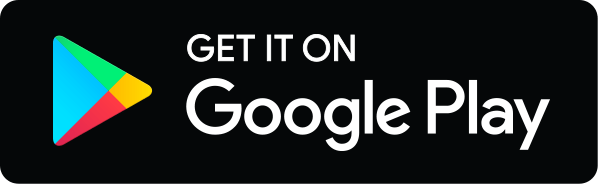