Chapter 16
Pain, Temperature Regulation, Sleep, and Sensory Function
Sue E. Huether, George Rodway and Curtis DeFriez
Pain
Pain is one of the body’s most important adaptive and protective mechanisms and all definitions suggest it is a complex phenomenon and cannot be characterized as only a response to injury. A widely accepted definition of pain is that drafted by the International Association for the Study of Pain (IASP) and accepted by the American Pain Society and the World Health Organization: “Pain is an unpleasant sensory and emotional experience associated with actual or potential tissue damage or described in terms of such damage.”1 Waddell defines pain as “…a symptom, not a clinical sign, diagnosis or disease.…”2 A clear understanding of the complexities of the pain experience—specifically one that encompasses an individual’s emotions, cognition, motivation, prior history, and even issues of secondary gain—is needed to manage pain and to further understand the pain processes. “The inability to communicate verbally does not negate the possibility that an individual is experiencing pain and is in need of appropriate pain-relieving treatment. Pain is always subjective.”2
Theories of Pain
Specificity theory proposes that injury activates specific pain receptors and fibers that project to the brain. Intensity of pain is directly related to the amount of associated tissue injury (i.e., pricking one’s finger with a needle would cause minimal pain, whereas cutting one’s hand with a knife would produce more pain). The theory is useful when applied to specific injuries and the acute pain associated with them. It does not account for chronic pain or cognitive and emotional elements that contribute to more complex types of pain.3
Pattern theory describes the role of impulse intensity and the repatterning of the central nervous system (CNS). The pattern theory is limited because it does not account for all types of pain experiences.4,5
Gate control theory (GCT) integrates and builds upon features of the other theories to explain the complex multidimensional aspects of pain perception and pain modulation.4 Pain transmission is modulated by a balance of impulses conducted to the spinal cord where cells in the substantia gelatinosa function as a “gate.” The spinal gate regulates pain transmission to higher centers in the CNS. Large myelinated A-delta fibers and small unmyelinated C fibers respond to a broad range of painful stimuli (mechanical, thermal, and chemical). These fibers terminate on interneurons in the substantia gelatinosa (laminae in the dorsal horn of the spinal cord). Nociceptive transmissions on these fibers “open” the spinal gate and increase the perception of pain. Closure or partial closure of the spinal gates can occur from non-nociceptive stimulation (i.e., from touch sensors in the skin). These signals are carried on non-nociceptive larger A-beta fibers and decrease pain perception. This is why rubbing a painful area may alleviate some of the discomfort. Other efferent CNS pathways descend to the spinal cord and may close, partially close, or open the gate modulating the pain experience. The gate control theory, bolstered by progress in understanding neuronal pathways in the peripheral and central nervous systems, has greatly advanced our understanding of pain. As good as the GCT has been, however, there are observations about pain in paraplegics that “do not fit the theory.”
Neuromatrix theory proposes that the brain produces patterns of nerve impulses drawn from various inputs, including genetic, psychologic, and cognitive experiences.6 The qualities we normally feel from the body, including pain, also can be felt in the absence of inputs from the body (as noted with phantom limb pain). In other words, stimuli may trigger the patterns but do not produce them. Neuromatrix patterns are normally activated by sensory inputs from the periphery, but may originate independently in the brain with no external input.7 The neuromatrix theory illustrates the plasticity (adaptable change in structure and function) of the brain. It does not supplant our understanding of the gate theory, and what we have learned about peripheral inflammation, spinal modulation, and midbrain descending control of pain. The neuromatrix theory expounds upon the gate control theory by explicating a body-self that provides a holistic, integrated, dynamic consideration of pain. However, there are many different kinds of pain and no single theory is adequate to explain the complex dynamics of the pain experience. Continuing research is advancing our understanding of the neural mechanisms of pain.8,9
Neuroanatomy of Pain
1. The afferent pathways, which begin in the peripheral nervous system (PNS), travel to the spinal gate in the dorsal horn and then ascend to higher centers in the central nervous system (CNS).
2. The interpretive centers located in the brainstem, midbrain, diencephalon, and cerebral cortex.
3. The efferent pathways that descend from the CNS to the dorsal horn of the spinal cord modulate pain.
Nociceptors
Nociceptors are free nerve endings in the afferent peripheral nervous system that selectively respond to different chemical, mechanical, and thermal stimuli. Nociceptors are located throughout the body (Table 16-1) but are not evenly distributed so the relative sensitivity to pain differs according to their location. The variable nature and distribution of nociceptors affects relative sensitivity to pain in different areas of the body. For example, fingertips have more nociceptors than the skin of the back, and all skin has many more nociceptors than the internal organs. Unlike sensory neurons of the special senses of vision, gustation, and olfaction (discussed later), which are required to detect only one type of sensory stimulus (e.g., light for the sense of vision), primary nociceptive afferents have the remarkable ability to detect a wide range of stimuli. To do this, nociceptors are equipped with an array of transduction channels that can sense different forms of noxious stimulation and at different intensities. In addition to the previously well studied voltage-gated potassium, sodium, and calcium channels, there are multiple types of transmembrane receptors (called transient receptor potential [TRP] channels), which reside on “naked nerve endings” and respond to a variety of physical, chemical, and thermal stimuli.10
TABLE 16-1
STIMULI THAT ACTIVATE NOCICEPTORS (PAIN RECEPTORS)
LOCATION OF RECEPTOR | PROVOKING STIMULI |
Skin | Pricking, cutting, crushing, burning, freezing |
Gastrointestinal tract | Engorged or inflamed mucosa, distention or spasm of smooth muscle, traction on mesenteric attachment |
Skeletal muscle | Ischemia, injuries of connective tissue sheaths, necrosis, hemorrhage, prolonged contraction, injection of irritating solutions |
Bone | Periosteal injury, inflammation, fractures, tumors |
Joints | Synovial membrane inflammation |
Arteries | Piercing, inflammation |
Head | Traction, inflammation, or displacement of arteries, meningeal structures, and sinuses; prolonged muscle contraction |
Heart | Ischemia and inflammation |
Nociceptors (primary order neurons) are categorized according to the stimulus to which they respond and by the properties of the axons associated with them. A-delta (Aδ) fibers are lightly myelinated, medium-sized fibers that are stimulated by severe mechanical deformation (mechanonociceptors) or by mechanical deformation and/or extremes of temperature (mechanothermal nociceptors). Aδ fibers rapidly transmit sharp, well-localized “fast” pain sensations. These fibers are responsible for causing reflex withdrawal of the affected body part from the stimulus before a pain sensation is perceived. The smaller unmyelinated C fibers are polymodal and are stimulated by mechanical, thermal, and chemical nociceptors. The unmyelinated C fibers slowly transmit dull, aching, or burning sensations that are poorly localized and longer lasting. A-beta (Aβ) fibers are large myelinated fibers that transmit touch and vibration sensations. They do not normally transmit pain but play a role in pain modulation.11
Pain transmission is the conduction of pain impulses along the Aδ and C fibers into the dorsal horn of the spinal cord and to the brainstem, thalamus, and cortex (Figure 16-1).
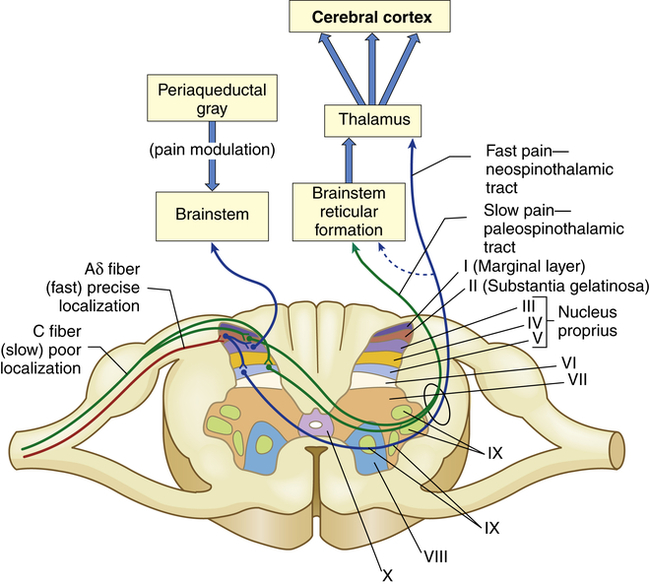
The myelinated Aδ fibers (fast localized pain) synapse on a second set of neurons that carry the signal to the thalamus via the neospinothalamic tracts. The C fibers (slow pain) synapse on laminae II and V interneurons that connect with neurons in laminae II, IV, and V and carry the pain signal to the reticular formation and midbrain via the paleospinothalamic tract. The axons of the spinothalamic tracts cross over the spinal cord to ascend in the anterior and lateral spinal cord white matter.
Pathways of Nociception
The cell bodies of primary-order neurons or pain-transmitting neurons reside in the dorsal root ganglia just lateral to the spine along the sensory pathways that penetrate the posterior part of the cord. Once the axons of the Aδ and C fibers enter the cord they synapse with interneurons (second-order neurons) that may branch into ascending or descending collaterals for one or two cord segments in neuronal projections called the dorsolateral tract. Eventually all of the primary afferents terminate on interneurons in the marginal layer (laminae I) or substantia gelatinosa (laminae II) of the spinal cord (Figure 16-2).
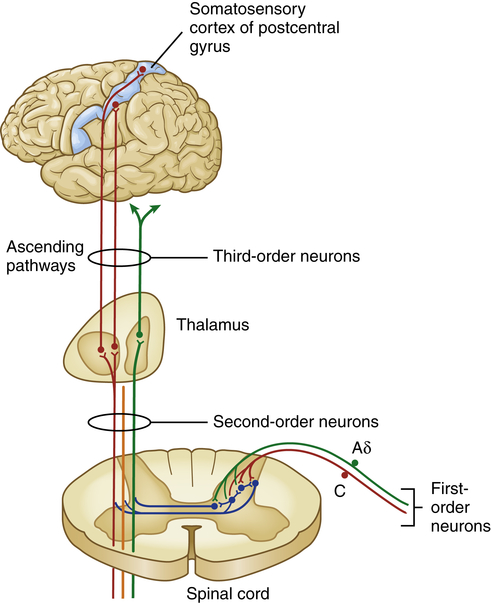
Aδ and C fibers comprise the primary, first-order sensory afferents coming into the gate at the dorsal horn of the spinal cord. Second-order neurons cross the cord (“decussate”) and ascend to the thalamus as part of the spinothalamic tract. Third-order afferents project to higher brain centers of the limbic system, the frontal cortex, and the primary sensory cortex of the postcentral gyrus of the parietal lobe.
Two classes of interneurons or second-order neurons are found in the dorsal horn: (1) excitatory interneurons, which relay nociceptive transmissions to projection cells, to other interneurons, or to motor cells concerned with local reflexes such as the pain withdrawal reflex; and (2) inhibitory interneurons, which modulate nociceptive transmission. The synaptic connections between cells of primary and second-order neurons located in the substantia gelatinosa and other spinal laminae function as a “pain gate.” The “gate” in the spinal cord regulates the transmission of pain impulses that ascend to the brain for further processing and interpretation (see gate control theory, p. 485).
From the dorsal horn, nociception continues on the axons of projection neurons as they cross the midline of the cord and ascend to various areas of the brain. These ascending fibers are organized into tracts or funiculi that are found in the white matter of the spinal cord, and are named according to their location in the cord and to where they project—either to the higher cord and brainstem or to the diencephalon (thalamus and hypothalamus) and limbic structures. Most nociceptive information travels by means of ascending columns in the lateral spinothalamic tract (also called the anterolateral funiculus) (Figure 16-3).
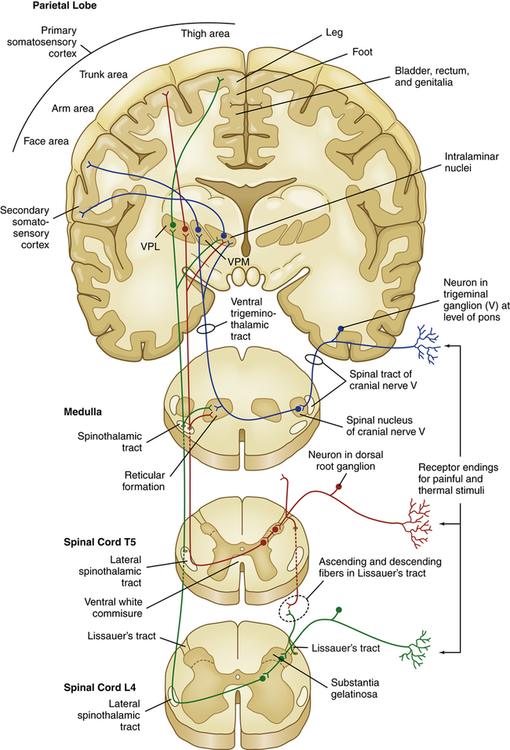
VPL, Ventral posterior lateral thalamic nuclei; VPM, ventral posterior medial thalamic nuclei.
From the thalamus, brainstem, and midbrain, third-order neurons project to portions of the CNS and cortex involved in the processing and interpretation of pain and neuroendocrine responses (e.g., the response to fright or to surgical stress).12 The extensive cortical networks that detect and react to pain are referred to as the “pain matrix.”13,14
Pain Modulation
Pain modulation is the physiologic process of suppressing or facilitating pain. Pain modulation involves many different mechanisms that increase or decrease the transmission of pain signals throughout the nervous system. Depending on the mechanism, modulation can occur before, during, or after pain is perceived.15
Pathways of Modulation
Several mechanisms modulate pain both at the level of the spinal cord and in the brain (Figure 16-4). Segmental inhibition of pain occurs when Aβ fibers (which synapse in the dorsal horn along with their nociceptive Aδ and C fiber counterparts) close the pain gates through an inhibitory interneuron. These afferent Aβ fibers carry non-noxious low-threshold mechanical information gained by touch, vibration, and pressure. For example, hitting your thumb with a hammer and holding the thumb or putting it into your mouth provides distractive input that lessens the pain.
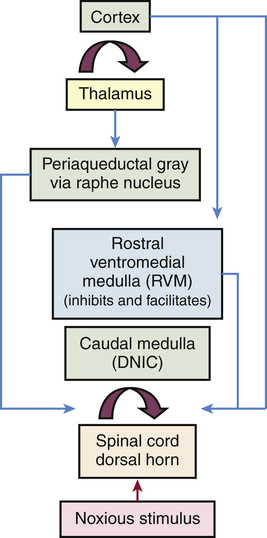
A noxious peripheral stimulus activates both segmental and top-down (corticofugal and bulbospinal heterosegmental) modulatory mechanisms, which either accentuate or inhibit afferent pain transmission to the brain. The most important and widespread source of top-down (corticofugal) modulation arises from the cortex. Both thalamic and prethalamic nociceptive relays are under the influence of this top-down control. The dorsal horn of the spine is also under the influence of the caudal medulla through diffuse noxious inhibitory control (DNIC). (Modified from Villanueva L, Fields HL: The pain system in normal and pathological states: a primer for clinicians, Seattle, 2004, IASP Press.)
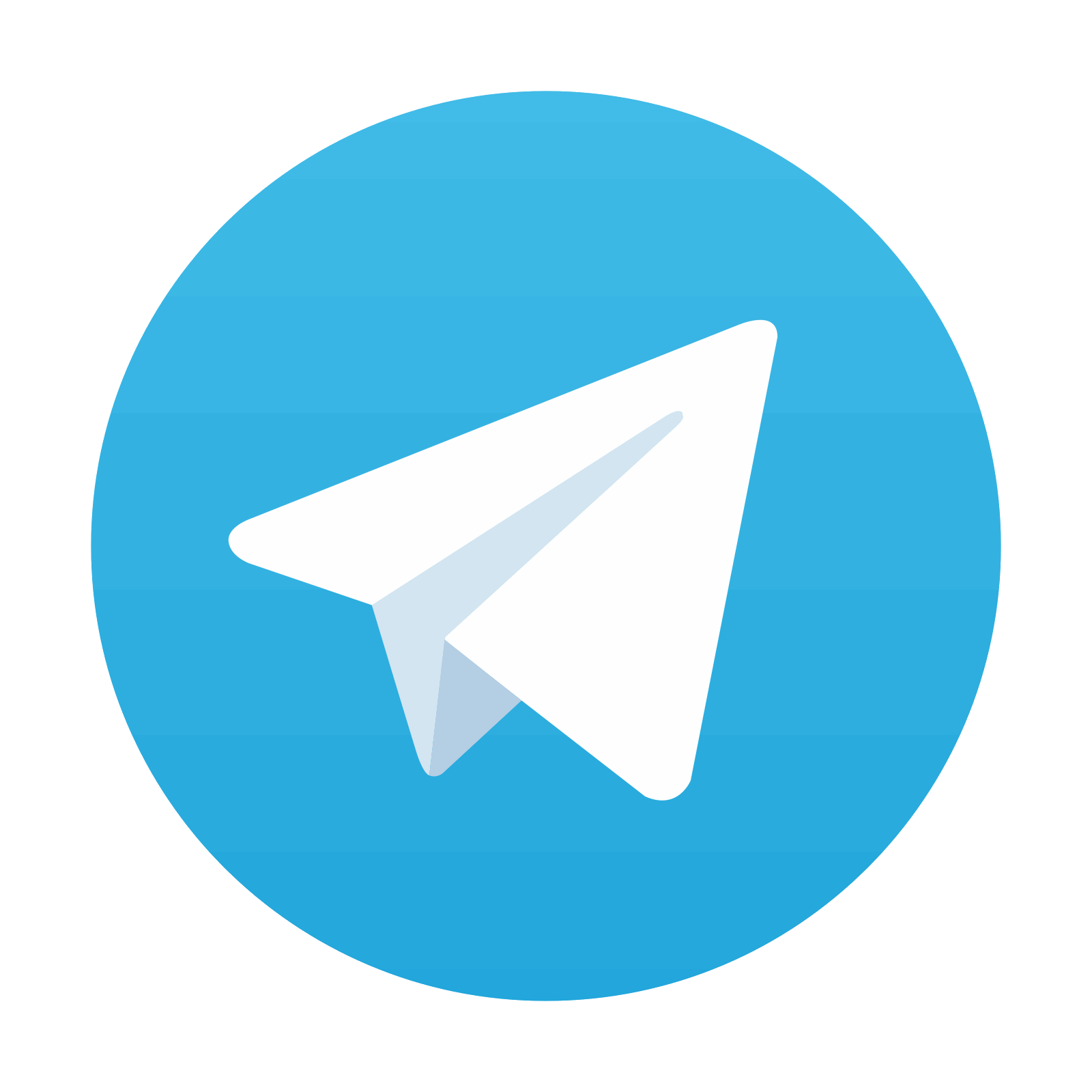
Stay updated, free articles. Join our Telegram channel

Full access? Get Clinical Tree
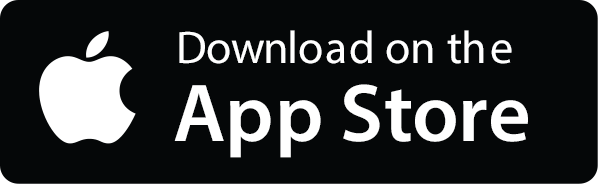
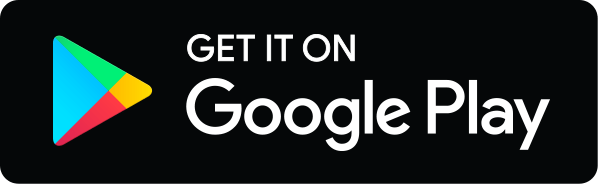