OBJECTIVES
1 Define open and closed fractures, dislocations, and subluxations.
2 Describe the clinical and radiologic features of fractures.
3 Outline the management priorities in treating fractures.
4 List the complications of cast immobilization of acute extremity injuries.
5 List and discuss the principles of physical rehabilitation of an extremity following immobilization for fracture healing.
6 List the vascular, neurologic, and musculoskeletal complications commonly associated with fractures.
7 Describe the clinical and radiologic features of subluxations and dislocations; outline their treatment.
8 Discuss common fractures and joint injuries; identify specific problems with their diagnoses and management.
9 Describe the indications and contraindications for replantation of an amputated appendage. Discuss the proper method of transporting the amputated part.
10 Define and list the signs, symptoms, and diagnostic criteria of a compartment syndrome; discuss its treatment.
11 Describe the pathophysiology of attritional sports-related injuries as they affect bone, muscle, and tendon.
12 Define the term sprain and its three gradations. Explain the methods of diagnosing the common sprains at the knee and ankle.
13 Demonstrate recognition of pediatric musculoskeletal problems, such as limb torsion and deformity, flat feet, developmental dysplasia of the hip, slipped femoral capital epiphysis, clubfoot, Legg-Calve-Perthes disease, and scoliosis.
14 Describe the signs and symptoms of an infectious process of the bone and joints.
15 List and discuss the diagnostic workup used in making a definitive diagnosis of a bone or joint infection.
16 Describe the signs and symptoms of an inflammatory (noninfectious) joint disease.
17 List and discuss the laboratory and radiologic techniques used in diagnosing osteoarthritis and rheumatoid arthritis.
18 Describe the pathophysiology of osteonecrosis.
19 List and discuss the nonsurgical and surgical treatment options of degenerative joint disease of the hip, knee, and spine.
20 List and discuss common causes of low back pain and cervical pain
21 Describe the signs and symptoms of lumbar or cervical disc herniation, and outline the diagnostic workup for these conditions
22 Define osteoporosis and osteomalacia and list the common etiologies of each
23 Discuss the pathophysiology, symptoms, and laboratory and radiographic findings of hyperparathyroidism and Paget’s disease.
24 List the common primary and secondary malignant neoplasms of bone
25 Outline the diagnostic workup for a patient with a suspected primary or secondary malignant neoplasm of bone
26 Describe the basic components of gait, and discuss common gait abnormalities in relation to mechanical or neurologic disorders
The musculoskeletal system is composed of connective tissue of mesodermal origin. Bones, joints, muscles, tendons, ligaments, and aponeurotic fascia constitute 70% of total body mass. Although disorders of the musculoskeletal system do not usually affect longevity, they frequently interfere with the quality of life. Musculoskeletal problems are the second most frequent cause of visits to a physician and are second in the consumption of health care dollars. Forty percent of emergency room visits are related to musculoskeletal problems. It is estimated that osteoporosis affects more than 20 million postmenopausal women and that associated hip fractures occupy almost 20% of surgical hospital beds. Back pain is the most common cause of time lost from work and disability in patients younger than 45 years of age. The annual cost of treatment and compensation for back conditions is greater than 14 billion dollars. Based on these statistics, it is clear that a working understanding of the musculoskeletal system is necessary to all physicians, especially those who practice primary care.
TRAUMA
Fractures, Subluxations, and Dislocations
A fracture is a break or loss of structural continuity in a bone. In a subluxation, the normally apposing joint surfaces are partially out of contact (Fig. 6-1A). In a dislocation, those surfaces are completely out of contact (see Fig. 6-1B). Joint subluxation may be a transient phenomenon in which the joint surfaces approach dislocation but reduces themselves.
Figure 6-1 A, Subluxation is a partial displacement of apposing joint surfaces. This phenomenon may be transient and reduce itself. B, Dislocation is complete displacement of apposing joint surfaces. A reduction maneuver is often needed to restore joint alignment. (Modified from Rockwood C, Green D. Fractures in Adults, 3rd ed. Philadelphia: JB Lippincott; 1982:1193.)
Fractures
Description
Knowledge of the accepted fracture nomenclature allows for communication between medical colleagues and may affect decision making. Therefore, it is essential to describe fractures in a precise and detailed manner. Fractures are described according to type, site, pattern, and degree of displacement. In children, growth plate fractures are described according to the Salter-Harris classification of growth plate injuries (discussed below).
Type. Fractures are either open or closed. A fracture is open when there is a break in the surrounding skin or mucosa that allows the fracture to communicate with the external environment. Although most open fractures are obvious to cursory inspection, others, such as pelvic fractures, may communicate with the rectum or vagina and are discovered only in the course of a thorough physical examination. All open fractures are by definition contaminated and require emergency treatment to prevent infection. A fracture is closed when the skin or overlying mucosa is intact.
Fractures usually are the result of a single forceful impact. However, repeated submaximal stress can produce microscopic fractures, which, if not allowed to heal, will coalesce into a stress fracture (discussed below). Stress fractures are frequently seen in army recruits or in insufficiently conditioned patients who participate in vigorous athletic training routines. A fracture produced by minimal trauma through abnormal bone is termed a pathologic fracture. Pathologic fractures occur in bone that is weakened by metabolic bone diseases (e.g., osteoporosis) or in bone harboring primary or metastatic tumors.
Site. When describing the location of a fracture, the bone affected is identified, as well as the specific site involved, such as the proximal or distal epiphysis, metaphysis, or diaphysis (Fig. 6-2). A fracture in the epiphyseal region suggests intra-articular fracture extension that would violate the joint surface and could result in traumatic arthritis. By convention, the diaphysis of a long bone is described in thirds: proximal, middle, or distal (Fig. 6-2). Fracture location has implications for healing. Fractures of metaphyseal or cancellous (spongy) bone with a rich blood supply and high bone turnover rates usually heal quite rapidly. In contrast, cortical, diaphyseal bone heals more slowly. Diaphyseal fractures, therefore, require lengthier periods of stress protection by immobilization or protection from weight bearing.
Figure 6-2 Anatomic regions of a long tubular bone.
Pattern. The fracture pattern suggests the type and amount of kinetic energy imparted to the bone. A transverse fracture (Fig. 6-3A) is a low-energy injury, usually the result of either a direct blow to a long bone or a ligament avulsion. A “nightstick” fracture is a transverse fracture of the ulna that occurs when the forearm is used to fend off a blow. Stress and pathologic fractures usually have a transverse pattern. Spiral or oblique fractures (see Fig. 6-3B,C) result from a rotatory, twisting injury. These fractures have a tendency to displace and shorten after reduction and immobilization. A fracture with more than two fragments is termed comminuted or multifragmented. The middle fragment may be triangular and is called a butterfly fragment (Fig. 6-4A); when cylindrical in configuration, it is described as segmental (Fig. 6-4B). Comminuted fractures occur as a result of larger forces and imply greater degrees of damage to the intramedullary blood supply to the bone and surrounding soft tissues, which may compromise the healing of one or both fracture sites. An impacted fracture (Fig. 6-5A; also see Fig. 6-15) is commonly seen in metaphyseal bone, such as with femoral neck, distal radius, or tibial plateau fractures. These are low-energy injuries in which two bone fragments are jammed together. A compression fracture signifies that trabecular or cancellous bone is crushed; it often occurs in vertebral bodies (Fig. 6-5B). Although most bone fractures are complete, an incomplete buckling of only one cortex is seen in children and is known as a greenstick fracture (Fig. 6-6).
Figure 6-3 Common fracture patterns. A, Transverse fracture. B, Spiral fracture. C, Oblique fracture.
Figure 6-4 Comminuted fractures. A, Triangular butterfly fragment. B, Cylindrical segmental fracture.
Figure 6-5 A, Impacted femoral neck fracture. B, Anterior compression fracture of vertebral body.
Figure 6-6 Greenstick fracture of the ulna in which only one cortex is broken (arrow) and the bone is bowed with its apex anterior. The fracture must be completed (the other cortex broken) to prevent angular deformity. Greenstick fractures typically occur in children whose bones are more plastic and less brittle than those of adults.
Displacement. Fractured bone fragments may be displaced by the force of an injury, gravity, or muscle pull. Displacement is described in terms of anterior-posterior (AP), medial-lateral, andlength (either shortening or distraction). Displacement is described in both the mediolateral (coronal) and the anteroposterior (sagittal) planes. The position of the distal fragment is always named relative to the proximal fragment. This naming convention is helpful, because most fractures are aligned by reducing the displaced distal fragment to the proximal one. Fracture displacement is customarily quantified as a percentage (Fig. 6-7). This description can be misleading because 50% posterior (Fig. 6-7B) and 50% lateral displacement (Fig. 6-7A), may, when viewed in three dimensions, represent only 25% bone apposition (Fig. 6-7C). Angulation is the relationship between the long axis of the distal fragment to the long axis of the proximal fragment. It may be described by one of two conventions. In the first convention, the direction to which the distal fragment is inclined is identified (Fig. 6-8). In the second convention, the location of the fracture angle apex is described. Reference to either the distal fragment or apical angulation should be mentioned in reports of fracture alignment. For example, in Figure 6-8, the distal fragment is inclined in the posterior and lateral directions, or the fracture apex is angled anteromedially.
Figure 6-7 Fracture displacement. A, The anterior-posterior (AP) view shows approximately 50% lateral displacement of the distal fragment. B, The lateral view shows 50% posterior displacement of the distal femur. C, Additively in three dimensions, the amount of bone apposition is approximately 25%, an amount that is underestimated by either view (A or B) in isolation.
Figure 6-8 A, On the anterior-posterior view (coronal plane), the distal fragment is angulated laterally and the fracture angle apex is medial. B, On the lateral view (sagittal plane), the distal fragment is angulated posteriorly and the angle apex is anterior.
The terms varus and valgus are also used in the descriptions of fractures and postural deformity. These terms refer to the direction of an angular deformity in relation to the midline of the body. If the deformity apex is pointed away from the midline (Fig. 6-9A), the term varus is used. If the deformity apex is directed toward the midline (Fig. 6-9B), it is called valgus. Thus, bowlegs in which the deformity apex at the knee (genu) is away from the midline are called genu varum, whereas knock-knees are called genu valgum.
Figure 6-9 The terms varus and valgus refer to the relation of a deformity to the midline of the body. A, Genu varum, or bowlegs, occurs when the deformity apex is pointing away from the midline. B, Genu valgum, or knock-knees, occurs when the deformity apex is directed toward the midline; it is called valgus. In knock-knees, the knee resembles the letter L, which is a helpful mnemonic in distinguishing between these confusing terms. (Modified from American Orthopaedic Association. Manual of Orthopaedic Surgery, 6th ed. Park Ridge, IL: American Orthopaedic Association; 1985.)
Fracture apposition, angulation, and shortening are quantified in percentage, degrees, and centimeters, respectively, from radiographs. Rotation describes angular shifts around the long axis of the bone. It is best judged clinically. Rotational deformity is expressed by identifying the position of the distal fragment as it relates to the proximal one. For example, if the foot is twisted outward, the fracture is externally rotated.
The Salter-Harris Classification of Growth Plate Fractures. In children, the growth plate (physis) is a zone of cartilage situated between the epiphysis and the metaphysis of long bones. Cartilage is weaker than bone and thus it is a common site of fracture. The Salter-Harris classification of growth plate injuries is descriptive, is generally recognized, and has important prognostic implications (Fig. 6-10).
Figure 6-10 Salter-Harris classification of pediatric growth plate fractures. The greater the classification number, the more violent is the injury to the epiphyseal plate and the greater the risk of long bone growth and healing problems. (Reprinted with permission from Rang MC. Children’s Fractures, 2nd ed. Philadelphia: JB Lippincott; 1983.)
The Salter-Harris type I fracture is a separation of the epiphysis from the metaphysis (Fig. 6-10). If the periosteum is not torn, the fracture remains undisplaced. In this injury, there is tenderness over the growth plate. The Salter-Harris type II fracture passes through the growth plate and exits through the metaphysis. This fracture is due to a bending movement that tears periosteum on the side opposite the triangular metaphyseal fragment. In a Salter-Harris type III injury, the fracture extends from the growth plate through the epiphysis to enter the joint. This fracture is intra-articular and requires a perfect reduction to avoid arthritic sequelae. The Salter-Harris type IV fracture line extends from the metaphysis through the growth plate cartilage into the epiphysis. This fracture pattern is also intra-articular. These fractures must be operatively fixed to prevent nonunion and joint surface incongruity. Salter-Harris types III and IV fractures have the highest incidence of growth disturbance if not properly managed. A Salter-Harris type V fracture involves a crushing of the epiphyseal growth plate. These fractures may appear innocuous on radiographs and therefore are difficult to identify prospectively. The type V injury causes a bony bar to replace the growth plate, which will result in asymmetrical, angular growth.
Growth plate injuries, no matter how trivial, have the potential to cause growth disturbance of the involved long bone. The larger Salter-Harris numbers represent greater degrees of injury to the growth plate. Consequently, the type IV fracture has a poorer prognosis and higher incidence of growth disturbance than the type I injury. The possibility of a growth disturbance requires that all growth plate fractures be followed radiographically for at least 1 year after injury.
Evaluation of Patients with Musculoskeletal Trauma
The patient usually has a history of injury, although in pathologic fractures the injury may be minimal. In children, either a limp or the refusal to use an extremity suggests a possible fracture. Symptoms of musculoskeletal injury include pain, swelling, and deformity. Bony tenderness, crepitus, or deformity strongly suggests a fracture. The examiner should inspect the extremity circumferentially for small puncture wounds. Vascular integrity (pulses, capillary return) and neurologic status (sensory, motor, and reflex functions) must be assessed and documented. Active motion of articulations distal to the fracture site implies an element of soft tissue integrity and neurologic function. The two most important clinical features of the fracture (whether it is open or not and whether the neurovascular status is compromised) are determined by clinical examination before x-ray films are obtained.
A complete radiologic evaluation includes the following:
1 Two views of the affected bone or joint at right angles to each other must be taken. Because fractures occur in three dimensions, a single radiographic view will not permit an accurate description (i.e., displacement and angulation) of the injury (Fig. 6-7). Two views taken perpendicular to each other, usually an AP and a lateral view meet these requirements.
2 The joint above and the joint below the injured area must be visualized. It is not uncommon for a knee injury or hip fracture to be associated with a fracture of the femoral shaft.
3 Known injury associations warrant special radiographic examination. Cervical spine radiographs are mandatory in all patients with facial and head injuries; hip dislocations are associated with injuries to the knee. Fractures of the axial skeleton (spine and pelvis) occur with injuries to the thoracic, abdominal, or pelvic viscera, as well as neural structures.
4 If a fracture is not evident radiographically but is suspected clinically (e.g., scaphoid fracture), the patient will not be harmed if the extremity is immobilized and reassessed. Repeated radiographs, stress views, or other imaging techniques—such as polytomography, bone scan, computed tomography (CT), or magnetic resonance imaging (MRI)—may be needed to establish the diagnosis.
Trauma patients with long bone fractures are prone to fat embolism. Clinical examination should watch for petechiae in conjunction with alteration of mental status. Patients are often dyspneic and have low oxygen saturation. Chest x-ray films reveal diffuse opacification without focal findings. Fat embolism syndrome is a clinical diagnosis because there is no specific laboratory finding. Treatment is supportive and in severe cases may require intubation and positive pressure ventilation with up to 100% O2.
Principles of Fracture Management
A patient with a fracture should be managed as a traumatized patient (see Chapter 10 in Lawrence’s Essentials of General Surgery, 4th ed). Life-threatening conditions are always treated first. It is essential to check the integrity of neural and vascular structures distal to any fracture site. All musculoskeletal injuries must be splinted in the field. Splints are to remain in place whenever the patient is transported. Splinting prevents fracture motion, thus minimizing further damage to the surrounding soft tissues (nerves, blood vessels, and muscle; shown in Fig. 6-16), limits blood loss, and decreases the pain of injury. Proper splinting requires that the joint above and the one below the fracture site be immobilized. Similarly, with a dislocation, the bone above and the bone below the joint should be splinted.
All open fractures are considered contaminated with bacteria. Treatment is aimed at preventing subsequent infection. After the extremity has been splinted, a culture is taken from the wound and the wound is covered with a sterile dressing. Tetanus prophylaxis is administered if necessary (see Chapter 3) and antibiotic treatment initiated. Intravenous first-generation cephalosporin is used for mildly contaminated wounds. Extensive open wounds or those that occurred in barnyards should receive an aminoglycoside and metronidazole (Flagyl) in addition. The patient is prepared for surgery, preferably under general anesthesia. A 1-mm margin of devitalized skin is excised, with care being taken not to sacrifice viable skin. The wound is extended as needed to expose the bone ends and debride all foreign material and necrotic tissue. The wound is irrigated with copious amounts of normal saline (≥6 L). Only after thorough irrigation and surgical debridement is an open fracture reduced and immobilized. The wound is reexplored 48 to 72 hours later for debridement of tissue that was marginally viable at first surgery and has subsequently necrosed. Delayed wound closure or the need for special soft tissue coverage techniques (e.g., a myocutaneous or a free microvascular flap) are planned at the “second look” procedure (see Chapter 3).
Fracture management requires knowledge of the stages of fracture healing (Table 6-1). The two principles of fracture care are obtaining a reduction and maintaining the reduction.
Reduction. Fracture deformity is reduced to restore bone apposition and alignment. Reduction can be achieved by closed or open methods. Closed reduction involves the manipulation of the fracture into a functional position. Generally, in a closed reduction, traction is applied to the distal segment to separate the impacted fragments. Force is applied to realign the bone fragments. Whenclosed reduction has been unsuccessful,an open reduction may be required. The fracture is surgically exposed, and bone fragments are manipulated directly. Open reduction is indicated when closed reduction methods fail or with intra-articular fractures in which the joint surface must be perfectly restored to prevent the development of post-traumatic arthritis.
Maintenance of Reduction. Once the fracture has been reduced, alignment must be maintained until the process of bone healing is completed. Maintaining alignment requires some form of fracture immobilization, which may include casting, traction, functional bracing, and internal or external fixation. The type of immobilization employed depends on fracture stability or its propensity for displacement. A circumferential plaster or fiberglass cast is the traditional method of immobilization. A cast protects and maintains fracture alignment until healing occurs. Early clinical and radiographic follow up is necessary to ensure that fracture reduction is not lost as swelling diminishes.
Continuous traction applied through the skin, the skeleton, or by gravity is a technique that can both effect and maintain reduction. With skin traction, a foam rubber boot or sleeve is wrapped directly against the skin and traction is applied via friction of the foam–skin interface. The risk of skin breakdown limits the amount of traction that can be used to no more than 7 lb. Therefore, skin traction is useful in small children or to temporarily splint an adult with a hip fracture before surgery. Skeletal traction requires that a pin be inserted through bone distal to the fracture site. Large distraction forces can then be applied directly to the bone and can overcome the contractile forces of large muscles in patients with pelvic, femoral, or tibial fractures (Fig. 6-11). A common site for skeletal traction pin placement is the proximal tibia (occasionally, the distal femur or calcaneus); in the upper extremity the pin placement site is often through the olecranon process of the ulna. Gravity acting through a dependent extremity can also act as a traction force. In humeral fractures, the weight of the distal arm applies traction if the body is kept upright (Fig. 6-12A). Application of a forearm cast can augment this type of traction (Fig. 6-12B).
Figure 6-11 Skeletal traction applied through a pin placed in the tibia is useful for treating femur or pelvic fractures. The leg is supported in a suspension apparatus, and the foot of the bed is raised to permit body weight and gravity to act as countertraction.
Figure 6-12 Gravity traction. A, With the weight of the arm supported by a collar and cuff. B, With a hanging cast.
Several complications are associated with casts and traction. Circumferential bandages may cause circulatory impairment in acutely traumatized limbs in which further swelling is expected. A cast or dressing that is too tight must be completely released to the level of skin. Excessive traction can cause nonunion and peripheral nerve injury. Ulcerative skin problems may occur with both skin and skeletal traction. Skeletal traction causes frictional shearing forces between the patient’s sacrum and the bed, which can result in a sacral decubitus ulcer. A poorly applied cast can cause a pressure ulcer over an inadequately padded bony prominence or a displaced bone end. Joint stiffness and muscle atrophy are common problems after prolonged immobilization.
Functional braces allow for early joint motion while maintaining fracture alignment through a compressive hydraulic effect on the soft tissues. Conversion from a cast or splint to a functional brace after early evidence of fracture healing hastens both healing and rehabilitation.
Internal fixation devices include pins, screws, plates (Fig. 6-13), circumferential wires or bands, and intramedullary rods (see Fig. 6-19). Indications for internal fixation are listed in Table 6-2. Metallic fracture fixation implants may appear sturdy on radiographs. However, like a cast, they simply position the fracture until healing is complete. Fracture fixation hardware should be considered an internal splint that must respect the biology of fracture healing. The mere presence of an internal fixation device does not guarantee fracture healing. If the fracture does not unite, repetitive (cyclic) loading of a fracture implant will ultimately lead to its loosening or to metal fatigue and breakage. Whenever internal fixation is employed, there is a race between fracture healing and implant failure. Although internal fixation enhances early patient mobility, it has a number of potential complications. Internal fixation requires a surgical exposure that itself can devitalize tissue and adds to the risk of infection and nonunion. A second surgical procedure may be needed if the implant is to be removed. Finally, after hardware removal, the bone can refracture through screw holes, especially when they are in cortical, diaphyseal bone.
Figure 6-13 Radiographs of a comminuted ankle fracture. A, Anterior-posterior radiograph. B, Lateral radiograph. C, Intra-articular fracture treated with a complex array of internal fixation plates and screws.
External fixation is a minimally invasive method of maintaining fracture alignment. Threaded pins are placed into the bone above and below the fracture site and are attached to an external frame to immobilize the fracture (Fig. 6-14). It functions as a portable traction device. Indications for external fixation are listed in Table 6-3. Complications include pin track infection and delayed union.
Figure 6-14 Severely comminuted open tibia fracture treated with an external fixator. This form of fixation immobilizes the fracture while permitting access to the wound for observation and care.
Rehabilitation of Function. Rehabilitation planning begins with the initial phases of fracture management. To avoid joint stiffness common to periarticular and intra-articular fractures, the limb is immobilized in a position of maximum function. Isometric exercises of immobilized muscles are started to avoid excessive atrophy. Range-of-motion exercises for adjacent joints that are not immobilized are encouraged from the onset of care. After a cast or brace is removed, active range-of-motion and resistive muscle strengthening exercises are initiated.
The speed of rehabilitation depends on the rate and quality of fracture healing. Exuberant rehabilitative activities or exercises may result in delayed healing, implant failure, and loss of reduction. A rational rehabilitation plan incorporates those factors that influence the speed and success of fracture healing. These factors include the amount of energy imparted to the bone during injury (open, multifragmented, and displaced fractures heal slowly), the type of bone involved (cancellous or cortical), the integrity of the soft tissue envelope, and the patient’s general health and age (children heal more rapidly than adults).
Bone healing is evaluated clinically and radiologically. Clinically, healing is evident when the fracture is no longer tender to palpation or stress. Radiographically, healing is evident when distinct bony trabeculae are seen crossing the fracture site on radiographic images.
Complications of Fracture Healing
Local Complications
Local complications of fracture healing include infection, delayed union, nonunion, malunion, avascular necrosis, and, in children, growth disturbances. Fractures that are open, either from injury or surgical intervention, have a higher incidence of infection than closed fractures. Delayed union is characterized by fracture healing that appears to be taking place, but slower than the usual. Nonunion is characterized by incomplete and nonprogressive fracture healing. The nonunited fracture gap may be filled with fibrous tissue or, if subjected to significant motion, may form a synovial membrane with joint fluid called a pseudarthrosis (a “false joint”). Delayed unions and nonunions are caused by fracture separation, soft tissue interposition, excessive fracture motion, inadequate vascularization of the fracture segments, or infection. When a fracture heals with a deformity that causes cosmetic or functional impairment, it is called a malunion. Malunited fractures can be shortened, angulated, or rotated. A corrective osteotomy may be required to regain alignment and function.
Avascular necrosis occurs when the blood supply to a bone is injured by the traumatic event (see Bone Necrosis later in this chapter). Bones that are extensively covered by articular cartilage and have a minimal muscular envelope are particularly vulnerable to osteonecrosis (e.g., the femoral head, the scaphoid, or the talus).
Growth disturbance is a fracture complication specific to children. The epiphyseal plate is composed of cartilage and is the site of longitudinal growth in bones. Because cartilage is weaker than bone, the growth plate is often involved in pediatric fractures. Fractures in children may damage the growth plate, especially by compressive or shearing mechanisms. When the entire growth plate is damaged, growth will cease and the affected limb will be shorter than the unaffected limb by the end of growth. If only part of the epiphyseal plate is damaged, the bone may grow asymmetrically and cause an angular deformity. A growth plate injury can be detected only by serial radiographs. Most growth plate problems can be identified by radiographs taken at the 1-year anniversary of the injury, a date that parents should mark down so they are reminded to present for follow-up radiographs. Lower limb shortening of less than 1 cm is well tolerated; shortening between 1 and 2 cm can be managed by a shoe lift. A leg length discrepancy of more than 2 cm can be corrected by fusion of the opposite growth plate, a procedure known as epiphysiodesis. The timing of this procedure is calculated from growth tables. Angular deformity from a partial growth plate arrest is managed surgically and is best handled when diagnosed early.
Post-traumatic arthritis is a complication of displaced intra-articular fractures. Articular cartilage has no blood supply and depends on synovial fluid for nourishment. When injured, articular cartilage has minimal healing potential. If intra-articular fractures are not anatomically reduced, the irregular surface may cause rapid arthritic change.
Arthritis can also develop indirectly from a severe angular deformity. Weight-bearing forces can be concentrated in the part of the joint causing abnormal stress concentration and joint wear. Depending on the magnitude of injury, post-traumatic arthritis can occur rapidly or slowly over a decade or more. Patients who have had a traumatic hip dislocation, for example, usually have hip arthritis in 10 to 20 years later.
Systemic Complications
Systemic complications are unusual following a fracture. Systemic complications usually result from trauma in general and not from the fracture itself. These complications include shock, sepsis, tetanus (in open injuries), gas gangrene, venous thrombosis, and fat embolism. The emergent stabilization of spine, pelvic, and long bone fractures is necessary to minimize blood loss and to allow a patient to sit upright and receive proper pulmonary physiotherapy. Accomplishing this can significantly decrease the incidence of respiratory insufficiency in multisystem trauma patients.
Joint Subluxation and Dislocation
Diagnosis and Evaluation
Subluxation of a joint is usually a transient phenomenon, when articular surfaces of a joint become partially separated. When a joint is dislocated, the articular surfaces of a joint are no longer in contact with each other. The patient is reluctant to move it. The limb may be held in a typical posture (e.g., when a hip is posteriorly dislocated, the thigh is held in flexion, adduction, and internal rotation). Neurovascular structures, in close proximity to joints, can be injured with dislocations, especially in older patients whose arteries may be thickened by atherosclerotic plaque. Not all vascular injuries are acute occlusive phenomena. An intimal tear of the artery may slowly cause thrombus formation, delaying the presentation of vascular compromise. Therefore, serial neurovascular evaluations are essential after the reduction of a dislocated joint. Asymmetry in pulses, which is detected by palpation or Doppler ultrasonography, warrants further vascular workup, especially in young patients who have had little stimulus to develop collateral circulation.
Radiographs of the involved joint are obtained in the dislocated posture. This radiograph demonstrates the pathology and allows the treating physician to infer which specific ligamentous structures are damaged. Like a fracture, a dislocation can be described as open or closed and according to the position of the distal fragment relative to the proximal fragment. If radiographic assessment will be delayed and if skin is compromised (e.g., ankle fracture or dislocation) or if neurovascular integrity is in question (e.g., knee dislocations), then reduction should be attempted immediately.
Treatment
Dislocations are usually realigned by traction along the normal axis of the extremity. Occasionally, bone or soft tissue may be interposed between joint surfaces and will require a surgical (open) reduction. Postreduction radiographs must be taken to ensure the adequacy of reduction and rule out an associated fracture.
Common Musculoskeletal Injuries
Upper Extremity
Carpal Scaphoid Fracture. The scaphoid is the bone most frequently fractured of the carpal bones. A fracture through the waist of the scaphoid usually occurs after a fall on the outstretched hand, with the wrist positioned in dorsiflexion and radial deviation. If a fracture is suspected from the mechanism of injury and tenderness in the anatomic snuff box, the patient should be treated as if there were a fracture (even if radiographic views do not indicate a fracture). A bone scan, CT scan, or follow-up radiographs at 7 to 14 days will confirm or disprove the diagnosis. The scaphoid bone is extensively covered by hyaline cartilage and has limited soft tissue attachments and blood supply. Complications of avascular necrosis, delayed union, and nonunion are increased by failure to treat a scaphoid fracture initially. Undisplaced fractures are treated in a thumb spica cast (a forearm cast extended to incorporate the thumb in the pinch position). Displaced scaphoid fractures are treated by open reduction and internal fixation.
Figure 6-15 Colles fracture of the distal radius is common to osteoporotic patients. The fracture occurs from a fall on an outstretched dorsiflexed hand. A, The anterior-posterior view demonstrates the impaction and shortening of the distal fragment (arrows). B, The lateral radiograph shows the dorsal cortex of the radius to be comminuted and impacted, which results in apex palmar angulation.
Distal Radius Fracture. A distal radius fracture is also caused by falling on an outstretched hand. When this injury causes a transverse fracture of the distal radius just proximal to the wrist it is referred to as a Colles fracture. It is a common fracture in elderly, osteoporotic patients. Radiographically, dorsal comminution can be noted, and the distal fragment is impacted and shortened with apex palmar angulation (Fig. 6-15). The ulnar styloid is often fractured.
Reduction is obtained by longitudinal traction applied to the hand, disimpacting the fracture. The wrist and distal fragment are manipulated into flexion and ulnar deviation to correct the dorsal and radial displacement. After reduction, a splint is applied from the elbow to the palm. A repeat radiograph should be taken following reduction at approximately 10 days to assess whether the reduction has been maintained. If it has not, a repeat manipulation or external fixation is required. The deformity tends to recur because of dorsal cortical comminution. Occasionally, a Kirschner wire, or K-wire, is inserted percutaneously to prevent loss of fracture alignment.
The median nerve is in close proximity to the volar aspect of the wrist. Its function must be documented before and after fracture manipulation. The shoulder–hand syndrome is a common complication of a Colles’ fracture in the elderly. In this syndrome, shoulder and finger stiffness results from disuse during the treatment period. Patients are encouraged to exercise the shoulder and fingers during the early phases of fracture healing.
Olecranon Fracture. An olecranon fracture is usually caused by a fall in which there is a direct blow to the point of the elbow. The fracture is displaced by contraction of the triceps muscle. Thus, there is loss of active elbow extension. The fracture also involves the elbow joint surface. Any displacement of the fracture fragments requires an open reduction to restore the articular surface and triceps integrity.
Pulled Elbow. This is a painful condition that affects young children aged 1 to 4 years. It occurs frequently when the child has been pulled forcibly by the hand. The child tends to hold the elbow slightly flexed and avoids moving it. The pain is believed to be due to impingement of the annular ligament of the radial neck. Treatment is to flex the elbow slightly and supinate the child’s hand. This repositions the annular ligament around the radial neck and relieves the symptoms.
Supracondylar Humerus Fracture. A supracondylar humerus fracture is commonly seen in children 5 to 10 years of age. It occurs from a fall on an outstretched hand with the elbow extended. The distal fragment is usually displaced posteriorly. It can cause significant neurovascular complications by entrapping the brachial artery and the median and radial nerves, either at injury or during reduction (Fig. 6-16). This fracture is a frequent cause of a forearm compartment syndrome because of ischemia (Volkmann’s ischemic contracture). It must be treated with great care and vigilance. Usual management of displaced fractures is with prompt reduction and percutaneous pin fixation in the operating room.
Figure 6-16 The dangerous supracondylar distal humerus fracture may entrap the brachial artery and the median and radial nerves. This fracture is associated with forearm compartment syndrome and warrants cautious and frequent neurovascular monitoring. (Reprinted with permission from American Academy of Orthopaedic Surgeons. Athletic Training and Sports Medicine, 2nd ed. Park Ridge, IL: American Academy of Orthopaedic Surgeons; 1991:277.)
A forearm compartment syndrome can be caused by kinking of the brachial artery at the site of the supracondylar fracture. If the flow of blood with the delivery of oxygen and removal of metabolic waste from the muscles is not restored, severe and even permanent muscle injury can occur. If muscle is ischemic at normal body temperature for more than 2 hours, there is some permanent muscle damage. If the warm ischemia time exceeds 8 hours, then the muscle is likely dead and revascularizing the limb can lead to systemic complications from hyperkalemia and release of myoglobin. The flexor compartment of the forearm is completely dependent on the blood supply from the brachial artery. If the muscles die, the fibers contract and leave a nonfunctional hand due to fingers and thumb that are flexed into the palm. There is no effective tendon transfer to restore hand and wrist function when the entire flexor compartment has stiffened and scarred.
Shoulder Dislocation. The shoulder is the most frequently dislocated joint in the body. In more than 90% of traumatic dislocations, the humeral head is anterior to the scapular glenoid fossa (Fig. 6-17A). The axillary nerve and artery can be endangered by this injury. The integrity of the axillary nerve should be documented by testing sensation over the deltoid patch and motor function of the deltoid muscle before and after reduction of the shoulder dislocation. An anterior shoulder dislocation occurs with forced external rotation of the abducted arm. This type of injury may be caused by an arm tackle in football or by blocking a basketball shot.
Figure 6-17 A, Axillary lateral radiograph of an anterior shoulder dislocation. The humeral head lies out of the glenoid fossa (G). The coracoid process (C) is an anterior scapular structure and orients the film. B, Closed reduction of an anterior shoulder dislocation via traction countertraction. a, acromion; cl, clavicle. (Reprinted with permission from Rockwood C, Green D. Fractures in Adults, 3rd ed. Philadelphia: JB Lippincott; 1982:1092.)
Reduction can be achieved by gradual shoulder abduction while longitudinal traction is placed on the arm and countertraction is placed through the axilla with a sheet (Fig. 6-17B). Sedation and muscle relaxation facilitate the manipulation.
Posterior shoulder dislocations, although rare, are often missed because of improper interpretation of the AP radiograph, which appears to show the humeral head aligned with the glenoid. An axillary view shows the humeral head to lie posterior to the glenoid, which should reinforce the principle that two radiographs taken in perpendicular planes are needed for proper radiographic evaluation of any bony structure. Clinically, the arm is held internally rotated and cannot be externally rotated beyond the neutral position. A posterior dislocation should be considered in all patients with shoulder symptoms after an electrocution or a seizure due to epilepsy, alcohol withdrawal, electroconvulsive therapy, or electrocution.
Hip Fractures. Low-energy hip fractures are common in elderly, osteoporotic patients. They account for about 33% of admissions to large orthopedic centers. The common types are femoral neck (Fig. 6-5A) and intertrochanteric hip fractures (Fig. 6-18). In both fractures, the affected limb is externally rotated and shortened. The patient cannot bear weight and slight amounts of hip motion cause pain.
Figure 6-18 A, Intertrochanteric hip fracture. The bone is osteopenic and rarefied. B, This fracture has been reduced and internally fixed with a screw and sideplate device. This type of fixation will permit early patient mobilization.
The blood supply to the femoral head comes from vessels that run along the posterior femoral neck. These vessels can be damaged by a femoral neck fracture. If the femoral head is rendered avascular, the bone cells die. When dead bone is subjected to repetitive weight-bearing loads, it will collapse and fragment. Femoral neck fractures have a higher incidence of nonunion because the fracture is within the hip joint (intracapsular), with a thin periosteum and no muscle envelope. Femoral neck fractures are either reduced and surgically fixed or, because of attendant complications, replaced with a metal hemiarthroplasty. An intertrochanteric hip fracture occurs outside the hip joint (extracapsular). There is a good blood supply, and the fracture usually heals (Fig. 6-18A). Intertrochanteric fractures are reduced under radiographic guidance and fixed with a sliding screw and sideplate device (Fig. 6-18B). Surgical treatment allows for early patient mobilization and decreases problems related to prolonged bed confinement (e.g., pneumonia, thrombophlebitis, decubitus ulcers).
Femoral Shaft Fractures. The femoral shaft is the strongest bone in the body. In young patients, femur fractures require high-energy trauma and are incurred by motor vehicle accidents and falls from heights. Blood loss may be considerable. In a closed fracture, 1 to 3 units of blood may be lost into the thigh and the patient may present in hypovolemic shock. Other sources of hypovolemia (e.g., such as intra-abdominal and intrathoracic injuries or pelvic fractures) must be excluded. In all patients with a fractured femur, the pelvis and hip must be assessed radiologically to rule out associated fractures or dislocations. Knee stability should be evaluated.
Closed, interlocked intramedullary nailing is the preferred treatment (Fig. 6-19). Because the nail can be locked proximally and distally, the fracture can be rendered quite stable and allow early ambulation. This approach prevents the lengthy periods of bed rest required with skeletal traction (Fig. 6-11) and minimizes the risks of venous thrombosis, knee stiffness, quadriceps contracture, muscle atrophy, and disuse osteoporosis.
Figure 6-19 Comminuted femoral shaft fracture after internal fixation with an interlocked intramedullary nail.
Hip Dislocation. Hip dislocation often occurs in motor vehicle accidents when the knee strikes the dashboard. Seated posture places the hip in adduction and 90 degrees of flexion. The longitudinal load of unrestrained dashboard impact drives the hip posteriorly out of the acetabular socket and may stretch the sciatic nerve. All patients with posterior hip dislocations should be assessed for a foot drop. The hip should be reduced urgently and associated fractures repaired to restore stability of the hip joint. Delays in treatment beyond 8 to 12 hours from injury can increase the risks for avascular necrosis of the femoral head and post-traumatic arthritis of the hip.
Tibia and Fibular Shaft Fracture. Shaft fractures of the tibia and fibula occur nine times more often than femoral shaft fractures. Almost 33% of the tibial surface is subcutaneous. For this reason, tibia fractures are often open and contaminated. The limited blood supply to the tibia causes fractures of this bone to have delayed union and nonunion. Tibia fractures are at risk for compartment syndrome (discussed later), which requires attentive observation and early diagnosis. The key clinical sign in a conscious patient is pain that is out of proportion to the injury. Complications associated with tibia fracture management are the most frequent cause of trauma-related orthopedic malpractice suits.
Closed reduction and above-the-knee cast immobilization are the standard treatment for uncomplicated closed fractures of the tibia and fibula. Open reduction and internal fixation are considered only when an acceptable reduction cannot be achieved by closed means or the reduction cannot be maintained by a plaster cast. External fixation (Fig. 6-14) is frequently used in managing open fractures of the tibia: stability and alignment of the fracture fragments are maintained while allowing access to treat the soft tissue wound. Current trends are toward adequate surgical debridement at the time of injury followed by internal fixation. The implant of choice is a reamed intramedullary nail.
Ankle Injuries. Ankle injuries are common in young, athletic individuals and may involve both ligamentous and bony structures. The ankle is a mortise and tenon joint. The three-sided mortise is composed of the tibial malleolus, the tibial plafond (ceiling), and the fibular malleolus. The talus represents the tenon. The mechanism of injury can be inferred from the plane of the fracture line (Fig. 6-20). A transverse fracture line occurs from a tensile or “pulling off” force. Thus, when the medial malleolus fracture is transverse, it suggests an abduction (eversion or pronation) force of the foot on the leg (Fig. 6-20B and 6-20C). If the lateral malleolus fracture is transverse, the force applied to the foot is adduction (inversion or supination; Fig. 6-20A). A spiral fracture configuration implies a rotatory force. A coronal plane spiral fracture is a common lateral malleolar fracture pattern and is seen when the foot is externally rotated on the leg and body (Fig. 6-20C). Bimalleolar ankle fractures are common. When a posterior tibial fragment is seen on the lateral radiograph, it is called a trimalleolar fracture and results from vertical loading of the plantar flexed ankle (Fig. 6-20D).
Figure 6-20 Ankle fractures. The basic mechanism of injury can be identified by the characteristic fracture patterns. A transverse fracture line implies that tensile, avulsive force was applied to the bone and is usually the first fracture to occur in the injury pattern. A, Adduction (inversion), in which the lateral malleolus is pulled off transversely and the medial malleolus is pushed off obliquely by the talus. B, Pure abduction (eversion) in which the medial malleolus is pulled off transversely, the fibula pushed off obliquely by the talus. The lateral malleolus is fractured in the sagittal plane. In some cases, the fibula is fractured above the joint line, indicating a tear in the interosseous membrane. C, Abduction (eversion) and external rotation (common), in which the medial malleolus is pulled off transversely while the lateral malleolus is obliquely fractured by the talus as it externally rotates and abuts the fibula. The fibular fracture is in the coronal plane. D, Vertical load, in which the posterior malleolus, seen best on a lateral x-ray film, can be fractured by a vertical compression load as the talus impacts the posterior tibia. The addition of this fracture fragment to any of the above constitutes a trimalleolar fracture. (Reprinted with permission from Rockwood C, Green D. Fractures in Adults, 3rd ed. Philadelphia: JB Lippincott; 1982.)
Because ankle fractures are intra-articular, anatomic restoration of the joint congruity is an essential treatment principle. One millimeter of ankle displacement can reduce joint surface contact by 40%. Anatomic open reduction with internal fixation is the ideal treatment for displaced ankle fractures.
Spinal and Pelvic Fractures
Spinal and pelvic fractures in young people result from high-velocity trauma and are associated with intrathoracic, intra-abdominal, and extremity injuries. In the elderly, spine fractures may occur after minimal trauma in bone that is weakened by osteoporosis or tumor.
Spinal Fractures. Spinal stability is the critical concept in the treatment of spinal fractures. The spine is unstable if unprotected movement causes fracture displacement that can compromise the integrity of neural structures. In all cases of suspected spinal injury, a complete and detailed baseline neurologic assessment should be performed and documented as soon as the patient’s condition permits. In unconscious patients or in those with any injuries above the level of the clavicle (facial), the cervical spine is presumed to be injured until proven otherwise (see Chapter 10 in Lawrence’s Essentials of General Surgery, 4th edition). Patients with minor wedge compression fractures (Fig. 6-5B) of the lower thoracic or lumbar spine often develop an ileus from retroperitoneal bleeding and should not be fed enterally until the ileus has resolved. If paraplegia results from a catastrophic spinal column injury, the signs of other injuries are masked by the lack of sensation. A systematic and thorough examination of all vital structures must therefore be carried out in patients with paraplegic. The patient with suspected spine trauma is properly splinted at the site of injury in a cervical collar with the head secured by taped sandbags. The thorax, abdomen, and extremities are strapped to a spine board. Consultation with a neurosurgeon or an orthopedic surgeon is indicated if the physician has any doubt about the stability of the spine injury.
Pelvic Fractures. The pelvis transfers body weight through the sacroiliac joints and acetabula in stance and through the ischial tuberosities in seated postures. The pelvis also protects the lower abdominal and genitourinary tracts. The pelvis houses the extensive vascular arborizations of the iliac vessels and the lumbosacral plexus of nerves. Pelvic fractures usually occur after high-velocity blunt trauma and can be associated with massive blood loss and multiorgan system injuries (Fig. 6-21). Therefore, in a hemodynamically unstable patient, emergency pelvic stabilization with external fixation is considered essential to the trauma resuscitation. The two goals of acute pelvic fracture surgery are to stop bleeding and permit sitting stability to facilitate pulmonary physiotherapy.
Figure 6-21 A, Anterior-posterior (AP) pelvis radiograph depicting diastasis of the symphysis pubis. The retrograde cystogram demonstrates bladder compression from a large pelvic hematoma. This type of pelvic injury can cause massive amounts of internal hemorrhage. B, AP pelvis radiograph after internal fixation with a plate and screws. Fracture reduction decreases pelvic volume, which both stabilizes and facilitates tamponade of bleeding fracture surfaces.
Almost one in five pelvic fractures has a concomitant bladder or urethral injury in males. When blood is seen at the external urethral meatus or when the patient cannot pass urine, a retrograde urethrogram is obtained to evaluate the integrity of the urethra before an indwelling catheter is placed. With hematuria, an intravenous pyelogram is performed to show renal function. If blood is detected in the rectum or vagina, the pelvic fracture may be open. Open pelvic fractures are treated with a diverting colostomy after debridement and external fixation to prevent ongoing fecal soilage of the fracture.
Traumatic Amputations and Replantation
With the advent of microsurgical techniques, completely severed digits and limbs can be surgically reattached. Limb replantation is most successful if the part is amputated cleanly with a minimum of crushed tissue. Children enjoy better nerve regeneration than adults and are ideal candidates for replantation. A general rule applies to replantation: Because muscle tissue is sensitive to ischemic injury, the greater the amount of muscle attached to the amputated part, the poorer is the prognosis for its function after replantation.
The best amputation levels for replantation in adults are the thumb, multiple digits, and the wrist or metacarpal level of the hand. In children, amputations at any level have a good chance for successful replantation. Contraindications to replantation include amputations with large crush or avulsive components; body parts that have been amputated at multiple levels; individual digit amputations (other than the thumb), especially proximal to the middle phalanx; and amputation in older patients who have concurrent disease or mental instability.
An amputated part may remain viable for approximately 6 hours of warm (36°C) ischemia. Cooling decreases tissue metabolism and increases the duration of viability. Amputated tissues can tolerate up to 16 hours of cold (10°C) ischemia. Thus, preparation of a severed part for transportation should include cleansing of superficial contamination, wrapping in moist gauze, and placement in an airtight plastic bag that is then immersed in ice water. Dry ice is never used because it causes frostbite and further tissue damage.
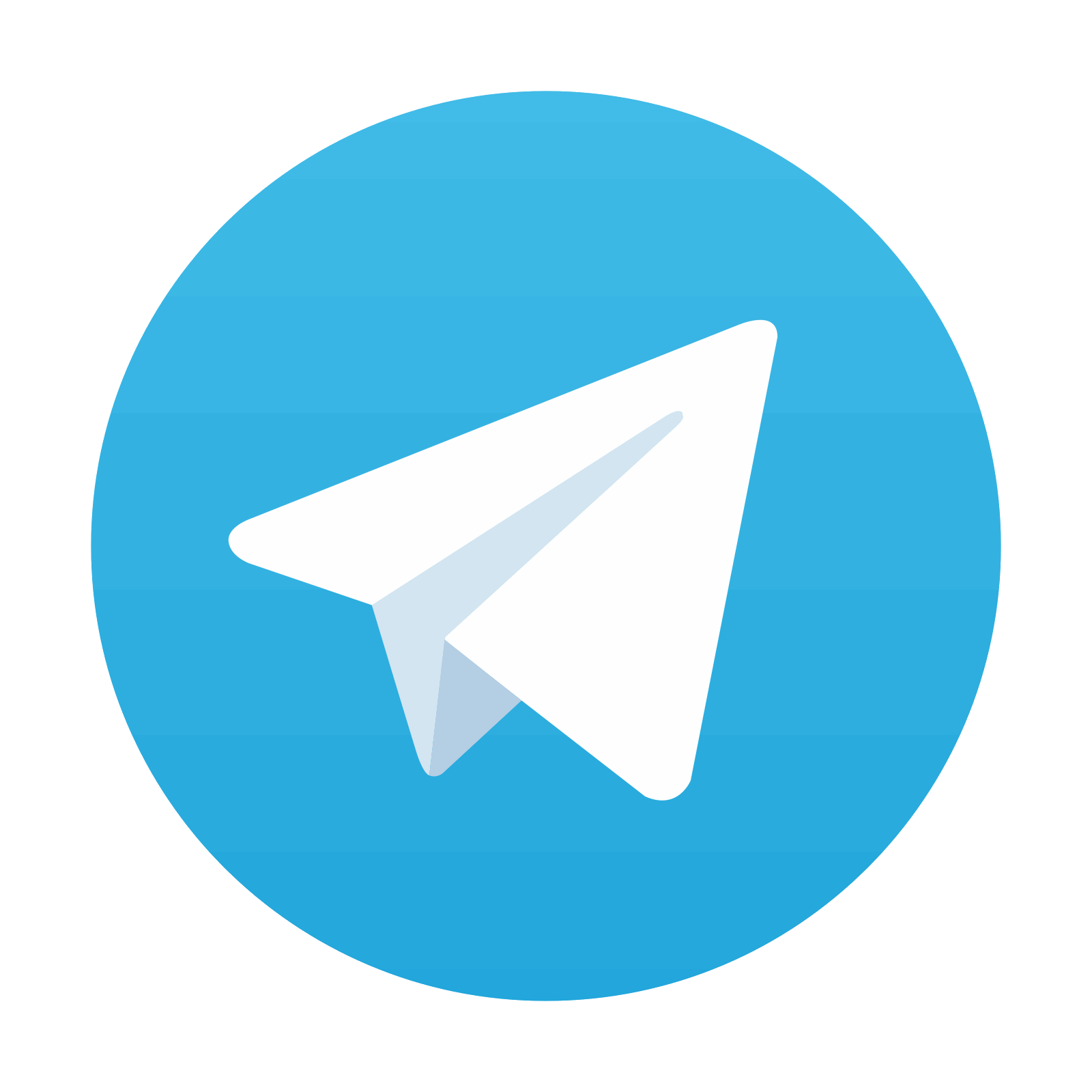
Stay updated, free articles. Join our Telegram channel

Full access? Get Clinical Tree
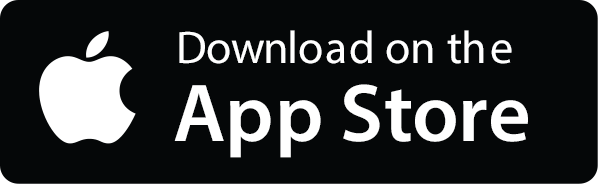
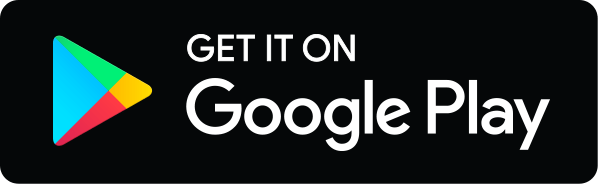