(1)
Canberra, Aust Capital Terr, Australia
Scientific Name
Brassica oleracea L. (Botrytis Group)
Synonyms
Brassica oleracea L. var. botrytis (L.) Alef, Brassica oleracea L. convar. botrytis (L.) Alef. var. botrytis f. botrytis, Brassica oleracea subsp. oleracea convar. botrytis var. botrytis L., Brassica botrytis subsp. botrytis, Brassica cretica subsp. botrytis var. cauliflora (DC.) Schwarz, Brassica botrytis var. cauliflora Presl, Brassica cauliflora subsp. abortiva Lizg., Brassica botrytis subsp. botrytis apud Lizg., Brassica cretica subsp. botrytis var. cauliflora (DC.) Schwarz, Brassica botrytis var. cauliflora Presl, Brassica cauliflora subsp. abortiva Lizg.
Family
Brassicaceae
English/Common Names
Cauliflower, Heading Broccoli, Perennial Broccoli
Vernacular Names
Afrikaans: Blomkool, Kool
Arabic: Karnabeet
Burmese: Paan Gor Bi
Chinese: Cai Hua, Gai Lan Hua, Hua Lan Cai, Hua Ye Cai, Ye Cai Hua, Ye Tsoi Fa
Croatian: Cvjetača
Czech: Brukev Květák, Květák
Danish: Blomkål, Blomsterkål
Dutch: Bloemkool
Eastonian: Lillkapsas
Esperanto: Florbrasiko
Finnish: Kukkakaali
French: Chou Brocoli, Chou Fluer, Chou-Fleur Commun, Chou-Fleur D’hiver
German: Blumenkohl, Kopfbrokkoli
Greek: Brókolo, Kounoupídi, Xylokrámvi
Hungarian: Karfiol
Icelandic: Blómkál
India: Phuulgobi (Assamese), Phool Gobi, Phuul Ghobi (Bengali), Fulaver, Phool Gobi, Phulkobi (Gujarati), Ghobi, Phool Gobhi, Phuul Gobhii (Hindi), Hukusu (Kannada), Phulkobi, Pulla Kosu (Konkani), Fulkobi, Phool Ghobi (Marathi), Phul Gobi (Oriya), Baha Kubi (Santhali), Kovippu, Pukosu (Tamil), Ghobi Puvvu, Khosu Povvu (Telugu), Phuul Gobhi (Urdu)
Indonesia: Kobis Bunga, Kol Bunga
Italy: Broccoli, Cavolo Broccolo, Cavolfiore, Cavolo Fiore
Japanese: Hana Kyabetsu, Hana Yana, Hana-Yasai, Kara-Shashin, Karifurawaa
Khmer: Phkaa Spei
Korean: Ja-Ju-Yang-Bae-Chu, Mo Ran Chae
Laos: Kalmapi Dook, Phak Ka Lam Dook
Latvian: Ziedkāposti
Lithuanian: Kalafioras, Ziedinis Kopūstas
Malaysia: Kobis Bunga, Kol Bunga
Nepali: Kauli
Norwegian: Blomkål, Blomkålhode
Pakistan: Gobhi, Phool Gobhi
Papua New Guinea: Oli Flva
Philippines: Kaliplawer, Koliplor (Tagalog)
Polish: Kalafior
Portuguese: Couve-Flor, Pugliese, Raminhos De Couve-Flor
Romanian: Conopidã
Russian: Kapusta, Kapusta Tsvetnaia
Serbian: Karfiol
Slovak: Karfiol
Spanish: Coliflor
Sri Lanka: Mal Gova (Sinhala)
Thai: Dok Kha Lam
Turkish: Karnabahar, Karnibahar
Ukrainian: Cvitna Kapusta
Vietnamese: Cải Bông, Su Lơ, Súp Lơ
Welsh: Bresychen Wen
Zulu: Ukholifulawa
Origin/Distribution
The primary area of cultivation is in the eastern Mediterranean region; from there it spreads to western and Central Europe before spreading globally, even to tropical countries where it is cultivated in the cooler mountain areas above 1,000 m.
Cauliflower and broccoli are believed to have evolved in Roman times from wild or primitive cultivated forms of Brassica oleracea in the eastern Mediterranean region; from there it spreads to western and Central Europe before spreading globally, even to tropical countries where it is cultivated in the cooler mountain areas above 1,000 m. During the last 400 years, white-headed cauliflower spread from Italy to central and northern Europe, which became secondary centres of diversity for annual and biennial types. Cauliflower adapted to hot and humid tropical climates has evolved in India during the last 200 years from biennial cauliflower of mainly British and Central European origin.
The leading cauliflower- and broccoli-producing counties in terms of total production (tonnes) are China 9,030,990, India 6,745,000, Spain 527,500, Mexico 427,884, Italy 420,989, France 334,170, United States 325,180, Poland 297,649, Pakistan 227,591, Egypt 201,200, United Kingdom 180,577, Bangladesh 168,238, Turkey 162,134, Japan 153,305, Germany 144,136, Indonesia 113,492, Belgium 99,660, Morocco 92,925 and Algeria 92,500 (FAO Stat 2012).
Agroecology
Cauliflower requires a cool, moist subtemperate climate, average daily temperatures of 15–20 °C; if temperatures go too high, the plants will not produce flower heads; if too low the plants might button, resulting in small heads. It is cold tolerant but will not survive severe frosts. It thrives best in full sun in well-drained, moist, fertile soil with significant organic matter and a pH of 6–7. As with all Brassicas, seeds germinate best with a soil temperature of around 25 °C.
Edible Plant Parts and Uses
Flowering head (curds) is edible. Cauliflower can be roasted, boiled, fried, steamed or eaten raw. When cooking, the outer leaves and thick stalks are removed, leaving only the florets. The florets should be broken into similar-sized pieces so they are cooked evenly. Cauliflower is often served with a cheese sauce, as in the dish cauliflower cheese, or with a meat gravy. Cauliflower stalks are fermented to produce ‘achar tandal’ in India. The leaves are also edible but are most often discarded. Low carbohydrate dieters can use cauliflower as a reasonable substitute for potatoes and is often used to produce a potato substitute known as ‘fauxtato’. Cauliflower is available as quick-frozen vegetables and processed in dried mixtures of soup vegetables.
Botany
An herbaceous annual with elongated cylindrical, non-fleshy stem base and growing to a height of 50–80 cm and higher to 150 cm when flowering. Basal and lower cauline leaves green, few to several, widely spaced, not grouped into a head, enclosing the compact terminal flowering head called the curd (Plate 1). The leaves are oblong-ovate, light greenish-grey, often simple and no lateral buds developed in the leaf axils. The curd consists of a dome of proliferated floral meristems white to cream or yellow (or purple in cultivars) on numerous fleshy peduncles (Plates 1, 2, 3 and 4). The curd is usually compact, sometimes loose, flattish or globose in shape. Bolting plants have several flower stalks. Inflorescence a raceme, 40–70 cm long with 4 merous bisexual flowers with green sepals, spathulate, yellow petals, 6 stamens—2 short and 4 long and superior ovary. Fruit a silique, 5–10 cm by 0.5 cm with 10–30 globose brown seeds.





Plate 1
Cauliflower leaves and curd

Plate 2
Harvested cauliflowers packaged for transport in an Indonesian market

Plate 3
Harvested cauliflower curds

Plate 4
A purple cauliflower curd
Nutritive/Medicinal Properties
Inflorescence/Leaf Nutrients and Phytochemicals
The proximate value per 100 g edible portion of raw cauliflower was reported as follows: water 92.07 g, energy 25 kcal (105kJ), protein 1.92g, total lipid 0.28g, ash 0.76g, carbohydrate 4.97g, total dietary fibre 2.0 g, total sugars 1.91g, glucose 0.94g, fructose 0.97 g; minerals, Ca 22 mg, Fe, 0.42 mg, Mg 15 mg, P, 44 mg, K 299 mg, Na 30 mg, Zn 0.27 mg, Cu 0.039 mg, Mn 0.155 mg, F 1 μg, Se 0.6 μg; vitamin C 48.2 mg, thiamine, 0.050 mg, riboflavin 0.060 mg, niacin 0.507 mg, pantothenic acid 0.667 mg, vitamin B-6 0.184 mg, total folate 57 μg, total choline 44.3 mg, vitamin A 13 IU, vitamin E (α-tocopherol) 0.08 mg, γ-tocopherol 0.2 mg, vitamin K (phylloquinone) 15.5 μg; total saturated fatty acids 0.064 g, 15:0 (pentadecanoic acid) 0.001 g, 16:0 (palmitic acid) 0.046 g, 17:0 (margaric acid) 0.001, 18:0 (stearic acid) 0.004 g, 20:0 (arachidic acid) 0.002 g, 22:0 (behenic acid) 0.001 g, 24:0 (lignoceric acid) 0.001 g, total monounsaturated fatty acids 0.017 g, 14:1: (myristoleic acid) 0.001 g, 16:1c (palmitoleic acid cis) 0.002 g, 18:1c (oleic acid cis) 0.014 g, 24:1c (nervonic acid) 0.001 g; and total polyunsaturated fatty acids 0.015 g, 18:2 n-6 c,c (linoleic acid) 0.006 g, 18:2 CLAs 0.002 g, 18:3 n-3 c,c,c (ALA, α-linolenic acid) 0.007 g, phytosterols 18 mg, tryptophan 0.020 g, threonine 0.076 g, isoleucine 0.071 g, leucine 0.106 g, lysine 0.217 g, methionine 0.020 g, cystine 0.020 g, phenylalanine 0.065 g, valine 0.125 g, arginine 0.086 g, histidine 0.056 g, alanine 0.116 g, aspartic acid 0.177 g, glutamic acid 0.257 g, glycine 0.071 g, proline 0.071 g, serine 0.086 g and lutein + zeaxanthin 1 μg (USDA 2012).
During period of cauliflower floral induction (2 weeks under 16 hours of light at 5 °C), there was a significant increase in sugar and starch content compared to that in vegetative plants grown at 20–26 °C (Sadik and Ozbun 1968). Sugar and starch content did not increase and flowering was prevented when light and CO2 were excluded during growth at 5 °C. A 3-day dark period at 20 °C or a high temperature treatment at 33 °C with light following growth at 5 °C reduced the carbohydrate level and prevented flowering.
Brassica plants (e.g. broccoli and cauliflower) contain substantial quantities of isothiocyanates (mostly in the form of their glucosinolate precursors) some of which (e.g. sulforaphane or 4-methylsulfinylbutyl isothiocyanate) are very potent inducers of phase two enzymes (Fahey et al. 1997). Three-day-old sprouts of cultivars of certain crucifers including broccoli and cauliflower were found to contain 10–100 times higher levels of glucoraphanin (the glucosinolate of sulforaphane) than do the corresponding mature plants. Sicilian cultivars of cauliflower possessing coloured curds displayed a high content of glucosinolates, glucoraphanin in particular, compared to white curd commercial cultivars; also some wild species had a high content of other glucosinolates (Branca et al. 2002). The glucosinolate content of 27 European cauliflower was similar to the American cauliflower cultivars but contained low amount of 2-hydroxy-3-butenyl-glucosinolate which was absent in the latter varieties (Sones et al. 1984). The major glucosinolates in European cauliflowers were identified as prop-2-enyl-glucosinolate, 3-methylsulphinyl propyl-glucosinolate, 3-indolylmethyl- glucosinolate and 1-methoxy-3-indolylmethyl-glucosinolate. Smaller amounts of but-3-enyl-glucosinolate, 2-hydroxybut-3-enyl-glucosinolate, 3-methylthiopropyl-glucosinolate, 4-methylthiobutyl-, 2-phenylethyl-glucosinolate, pent-4-enyl-glucosinolate, 2-hydroxypent-4-enyl-glucosinolate and 5-methylthiopentyl-glucosinolate were also found in some cultivars. Allyl-glucosinolate, 3-(methylthio)propyl-glucosinolate, 3-(methylsulfinyl)propyl-glucosinolate and 3-indolylmethyl-glucosinolate were present in all five American cultivars of cauliflower curd and were the major glucosinolates in their seeds as well (Carlson et al. 1987). Other glucosinolates present included 4-(methylsulfinyl)butyl-glucosinolate, 4-(methylthio)butyl-glucosinolate, 3-butenyl-glucosinolate, 4-pentyl-glucosinolate and 2-phenyl-ethyl-glucosinolate. Glucosinolates found in cauliflower purchased from local Singapore markets were in nmol/g wet weight: glucoraphanin NQ (not quantified), gluconasturtiin NQ, sinigrin 132 nmol, glucobrassicin 2,850 nmol, 4-hydroxy glucobrassicin 75.8 nmol, 4-methoxy glucobrassicin 231 nmol, 1-methoxy glucobrassicin 743 nmol, glucoiberin NQ, progoitrin (or epiprogroitrin) NQ, glucoalyssin 14.1 nmol, gluconapoleiferin NQ, gluconapin NQ, glucobrassicanapin NQ, glucoerucin NQ, 7-methylthioeptyl NQ, 8-methylthioeptyl NQ, total glucosinolates 4,190 nmol and glucobrassicans 93.2 % of total glucosinolates (Hecht et al. 2004). Glucobrassicins (glucobrassicin, 1-methoxyglucobrassicin, 4-methoxyglucobrassicin and 4-hydroxyglucobrassicin), precursors to indole-3-carbinols, were the predominant glucosinolates in seven of the nine vegetables (including cauliflower and broccoli) studied, accounting for 70.0–93.2 % of all glucosinolates.
The following phenolic compounds and glucosinolates were identified in cauliflower: four sinapic acid derivatives (1,2-disinapoyl-diglucoside; 1-sinapoyl-2-feruloyl-diglucoside; 1,2,2′-trisinapoyl- diglucoside; and 1,2′-disinapoyl-feruloyl-diglucoside), three flavonoids (quercetin-3-diglucoside-7-glucoside; kaempferol-3-diglucoside-7-glucoside; and kaempferol-3-diglucoside-7-diglucoside) and six glucosinolates namely 5-methylsulfinylpentyl glucosinolate (glucoalyssin); n-hexyl/methylpentyl glucosinolate (n-hexyl/methylpentyl-3-methylthiopropyl (glucoiberverin); 3-indolylmethyl glucosinolate (glucobrassicin); N-methoxy-3-indolylmethyl glucosinolate (neoglucobrassicin); 4-hydroxy-3-indolylmethyl glucosinolate (4-hydroxyglucobrassicin); and 4-methoxy-3-indolylmethyl glucosinolate (4-methoxyglucobrassicin)) (Gratacós-Cubarsí et al. 2010). The main hydroxycinnamic acids (sinapic, ferulic, p-coumaric and caffeic acids) were isolated from cauliflower by capillary zone electrophoresis (Lee et al. 2011).
The following phenolic compounds were found in cauliflower: quercetin derivatives (quercetin -3-O-sophoroside-7-O-glucoside and quercetin -3-O-(sinapoyl)-sophoroside-7-O-glucoside), kaempferol derivatives (kaempferol-3-O-sophorotrioside-7-O-sophoroside; kaempferol-3-O-sohorotrioside-7-O-glucoside; kaempferol-3-O-sophorotrioside-7-O-diglucoside; kaempferol-3-O-sophoroside-7-O-glucoside; kaempferol-7-O-glucoside; kaempferol -3-O-(caffeoyl)sophoroside-7-O-glucoside; kaempferol-3-O-(sinapoyl)-sophoroside-7-O-glucoside; and kaempferol-3-O-(p-coumaroyl)-sophoroside-7-O-glucoside) and hydroxycinnamic acids namely 3-caffeoylquinic acid); 1,2-disinapoylgentiobiose; 1-sinapoyl-2-feruloylgentiobiose; 1,2,2′-trisinapoylgentiobiose; and 1,2′-disinapoyl-2-feruloylgentiobiose (Cartea et al. 2011). Eight minor glucosinolates, namely, 1-methylpropyl-glucosinolate, 2-methylpropyl-glucosinolate, 2-methylbutyl-glucosinolate, 3-methylbutyl-glucosinolate, n-pentyl-glucosinolate, 3-methylpentyl-glucosinolate, 4-methylpentyl-glucosinolate and n-hexyl-glucosinolate were identified in crude sample extracts of cauliflower, broccoli and rocket salad (Lelario et al. 2012). The occurrence of these glucosinolates belonging to the saturated aliphatic side chain families C4, C5 and C6, presumably formed by chain elongation of leucine, homoleucine and dihomoleucine as primary amino acid precursors, was described. Isothiocyanate content in cauliflower juice treated by freezing, pasteurization, and high pressure was found to range from 0.79 to 25.92, 2.80 to 16.15,and 1.04 to 10.92 μmol/l, respectively (Totušek et al. 2011). Sulforaphane content in cauliflower juice treated by freezing, pasteurization, and high pressure was found to range from 0.66 to 1.61, 0.69 to 0.98 and 0.64 to 1.29 μg/ml, respectively.
No differences in free sugar (glucose, sucrose and fructose) or glucosinolate profiles in cauliflower were found between air and controlled atmosphere (3 % O2 and 5 % CO2 at 0 °C) (Hodges et al. 2006) storage treatments. However, the glucosinolates gluconapin (3-butenyl glucosinolate) and glucobrassicin (3-indolylmethyl glucosinolate) increased for each treatment during storage, albeit later in controlled atmosphere storage. Glucobrassicin was the major glucosinolate component, and the dramatic increase in the concentrations of this compound was reflected in increased total glucosinolate levels of air-stored cauliflower on day 28 of storage. Levels of the other glucosinolates (sinigrin (2-propenyl glucosinolate), progoitrin (2-hydroxy-3-butenyl glucosinolate), epiprogoitrin, 4-OH-glucobrassicin and 4-MeOH-glucobrassicin, as well as an unidentified glucosinolate) did not change during storage; glucoiberin content decreased after day 28. Increases in levels of gluconapin and glucobrassicin may be related to metabolic changes associated with natural and/or stress-induced senescence.
Brassica vegetables including cauliflower contain phytochemicals including glucoraphanin, the precursor of sulforaphane (SFN) and glucobrassicin, the precursor of indole-3-carbinole (I3C) and ascorbigen (ABG) (Wagner et al. 2010). Glucobrassicin was found to be enzymatically hydrolyzed to indole-3-carbinol, which in turn reacted with l-ascorbic acid to yield ascorbigen (Wagner and Rimbach 2009). The degradation of glucobrassicin was induced by plant tissue disruption. The formation of ascorbigen was dependent on pH and temperature. The degradation of ascorbigen in acidic medium released l-ascorbic acid and a formation of methylideneindolenine; in more alkaline medium, the degradation of ascorbigen yielded 1-deoxy-1-(3-indolyl)-α-l-sorbopyranose and 1-deoxy-1-(3-indolyl)-α-l-tagatopyranose.
A total of 28 compounds were identified in cauliflower agroindustrial by-products; of these, 22 were produced naturally by the plant (Llorach et al. 2003b). The main compounds found were kaempferol 3-diglucoside-7-glucoside and its combinations with different hydroxycinnamic acids. The new products isolated were identified as kaempferol 3-diglucoside-7-diglucoside, kaempferol 3-triglucoside-7-diglucoside, kaempferol 3-feruloyldiglucoside-7-diglucoside, kaempferol 3-sinapoyltriglucoside-7-glucoside, kaempferol 3-disinapoyltriglucoside-7-glucoside, kaempferol 3-sinapoyltriglucoside-7-diglucoside and kaempferol 3-disinapoyltriglucoside-7-diglucoside.
Three indole compounds indole-3-acetonitrile, indole-3-carbinol (I3C) and 3,3′-diindolylmethane (DIM) were identified from three cruciferous vegetables, Brussels sprouts, cabbage and cauliflower (Loub et al. 1975; Wattenberg 1975). Four known (isalexin, S-(-)-spirobrassinin, 1-methoxybrassitin, brassicanal C) and three new (caulilexins A–C) phytoalexins were isolated from cauliflower florets under abiotic (UV light) elicitation, and the first synthesis of brassicanal C was reported (Pedras et al. 2006).
Six organoselenium compounds including selenium analogues of known myrosinase-derived Brassica volatiles 4-(methylseleno)butanenitrile, 5-(methylseleno)pentanenitrile, 3-(methylseleno)propylisothiocyanate, 4-(methylseleno)butylisothiocyanate and 5-(methylseleno)pentylisothiocyanate were identified in the pentane/ether extracts of broccoli and cauliflower florets and roots of forage rape, all obtained from plants treated with sodium selenate (Matich et al. 2012). LC–MS analysis of ethanol extracts identified three selenoglucosinolates: 3-(methylseleno)propylglucosinolate (glucoselenoiberverin), 4-(methylseleno)butylglucosinolate (glucoselenoerucin) and 5-(methylseleno)pentylglucosinolate (glucoselenoberteroin).
Serine trans-hydroxymethylase, purified 46-fold from cauliflower, was found to be specific to serine (Mazelis and Liu 1967). Polyguanylate polymerase and polyadenylate polymerase were detected in the DNA-dependent RNA polymerase I fraction from cauliflower; poly(G) and poly(A) formed in-vitro by the enzyme fraction had chain length of 25–28 and 84–89 nucleotides (Mizuochi and Fukasawa 1976). Two distinct DNA polymerases, A and B, with molecular weights of 78,000 and 50,000, respectively, were isolated from a rapidly growing apical tissue of cauliflower inflorescence (Fukasawa et al. 1980). Polymerase-A bore strong resemblance to polymerase-alpha from mammalian cells in all properties examined, while polymerase-B was quite similar to polymerase-beta of mammalian cells. An ATP-independent DNA topoisomerase with molecular weight of 54,000 was isolated from chloroplasts of cauliflower leaves (Fukata et al. 1991). Cauliflower chloroplast topoisomerase could relax positive supercoils as well as negative supercoils. From these properties, cauliflower chloroplast topoisomerase could be classified as a eukaryotic type I DNA topoisomerase.
A protein with structure-specific endonuclease activity and a molecular mass of 40 kDa was purified from cauliflower inflorescence (Kimura et al. 1997). A DNA polymerase purified from cauliflower inflorescence-designated cauliflower polymerase 1 was found to be a monopolypeptide with a molecular mass of 100 kDa (Seto et al. 1998). The enzyme was clearly different from cauliflower mitochondrial polymerase. Tonoplast intrinsic proteins of molecular mass 23–29 kDa were detected in the meristematic tissues of cauliflower (Barrieu et al. 1998). Appreciable differences in the degree of methyl-esterification (ME) of pectic polysaccharides were detected in cauliflower stem (Femenia et al. 1998). About 65 % of galacturonic acid (GalpA) residues were methyl esterified in floret tissues. Relative ME showed a basipetal decrease, from 94 % in the upper stem to 51 % in the lower stem vascular tissues. The decrease was not related to a basipetal increase in glucuronic acid (GlcpA) residues. An acyl-CoA oxidase and a second protein of the plant nucleotide pyrophosphatase family purified from cauliflower inhibited both glutamine synthetase (GS) and nitrate reductase (Moorhead et al. 2003). S-methyl methanethiosulfonate, an antimutagenic compound, was isolated from cauliflower (Nakamura et al. 1993).
Cauliflower was found to accumulate dehydrin-like proteins with molecular weight of 10–100 kDa in the leaf and inflorescence mitochondria during some abiotic (cold) stress (Rurek 2010). A peroxidase, a primer antioxidant enzyme, with molecular weight 44 kDa was purified with 19.3-fold and 0.2 % efficiency from cauliflower buds (Köksal and Gülçin 2008).
Upon treatment with norflurazon, both cauliflower wild type and Or gene calli synthesized significant amounts of phytoene (Li et al. 2006). Phytoene was accumulated at comparable levels, and no major differences in carotenogenic gene expression were observed between the wild-type and Or calli, suggesting Or-induced β-carotene accumulation did not result from an increased capacity of carotenoid biosynthesis.
Studies showed significant differences among broccoli and cauliflower cultivar groups for the glucosinolate proportions as well as the contents of health-promoting and flavour-influencing alkyl, alkenyl and indole glucosinolates (Schonhof et al. 2004). These differences impacted on differences in sensory properties colour, taste properties such as bitter and sweet, flavour such as green/grassy, spicy, broccoli-like, cabbage-like, cauliflower-like, kohlrabi-like, leek-like and mouth-feel pungent. Consumers were seen to prefer cultivars with a bright colour, a lower level of bitter tasting glucosinolates (alkenyl and indole glucosinolates) and a higher sucrose content. Studies showed that neoglucobrassicin and sinigrin were responsible for the bitterness of cooked cauliflower (Engel et al. 2002). Allyl isothiocyanate (AITC), dimethyl trisulphide (DMTS), dimethyl sulphide (DMS) and methanethiol (MT) were the key odorants of cooked cauliflower ‘sulphur’ odours. They concluded that AITC, DMTS, DMS, MT, sinigrin and neoglucobrassicin were the potential physicochemical determinants of cooked cauliflower acceptance.
Cauliflower and red cabbage showed differences in their anthocyanin profiles: cyanidin-3,5-diglucoside was absent in cauliflower, while it was well represented in red cabbage, together with the characteristic anthocyanin of Brassica genus, cyanidin-3-sophoroside-5-glucoside (Scalzo et al. 2008). The p-coumaryl and feruloyl esterified forms of cyanidin-3-sophoroside-5-glucoside were predominant in cauliflower, while the sinapyl one was mostly present in red cabbage. All thermal treatments, except microwave heating, drastically reduced total cauliflower anthocyanin content.
From disrupted leaf tissues of cauliflower, the predominant vapour component was cis-hex-3-enyl acetate (Wallbank and Wheatley 1976). Apart from isothiocyanates, dimethyl disulphide was detected from cauliflower, particularly in vapour from disrupted curd tissue. From fresh Romanesco cauliflower Navona variety leaves extract, a total of 61 compounds were identified, representing 96.8 % of the oil (Valette et al. 2006). The major constituent was found to be hex-3(Z)-enol (61.1 %). From fresh disrupted inflorescence tissues of Romanesco, 35 compounds were detected, representing 97.7 % of the extract. Dimethyl disulphide, dimethyl trisulphide and hex-3(Z)-enol were identified as major constituents of the hydrodistillation products, representing, respectively, 30.2, 24.2 and 21.7 % of the volatiles. From ripening and frozen inflorescence tissues, dimethyl disulphide and trisulphide were again detected as predominant components. In the latter, hex-3(Z)-enol had almost disappeared (0.8 %) whereas dimethyl trisulphide represented 49.7 % of the oil. The steam distillation compounds present in the leaves and inflorescence tissues of cauliflower included limonene, α-pinene; β-pinene; α-terpineol; terpinene-4-ol; γ-terpinene; 4-methylene-1-(1-methylethyl)-bicyclo[3.1.0]hexane; nonanal; hexanal; camphor; decanal; eugenol; benzeneacetaldehyde; heptanal; 1-hexanol; (E)-2-hexenal; (Z)-3-hexen-1-ol; (E,E)-2,4-decadienal; trans-β-ionone; furfural; pyridine; 2-buten-1-one, 1-(2,6,6-trimethyl-1,3-cyclohexadien-1-yl)-, (E)-; methional; pinocarvone; dimethyl disulphide; dimethyl trisulphide; 2-pentyl-furan; acetic acid, hexyl ester; hexadecanoic acid, methyl ester; benzyl benzoate; (E,Z)-2,6-nonadienal; (Z)-3-hexen-1-ol, acetate; indole; tetradecanal; 2-methoxy-4-vinylphenol; (E)-2-Hexen-1-ol-; (E,E)-2,4-Heptadienal; 2,6-dimethyl pyrazine; 1-(2-furanyl)-ethanone; pentadecanal; 2,6,6-trimethyl-1-cyclohexene-1-carboxaldehyde; tridecanal; 1-docecene; (Z)-3-hexen-1-ol, benzoate; 2-pentadecanone; butanoic acid, 3-hexenyl ester, (Z)-; 1-tridecene; dimethyl sulphoxide; dimethyl tetrasulphide; 2-acetylthiazole; 3-ethyl-phenol; (Z)-2-penten-1-ol; 2,6,6-trimethyl-1,3-cyclohexadiene-1-carboxaldehyde; 4-methyl-1-(1-methylethyl)-bicyclo[3.1.0]hex-3-en-2-one; 4-vinylphenol; 2-hexadecanone; (E,E)-3,5-octadien-2-one; 2,2,6-trimethyl-cyclohexanone; 1,2-dihydro-1,1,6-trimethyl naphthalene; 4-(2,2,6-trimethyl-7-oxabicyclo[4.1.0]hept-1-yl)-3-buten-2-one; methyl (methylthio)methyl disulphide; 1-(methylthio)pentane; β-damascone; trans-2-(2-pentenyl)furan; benzenepropanenitrile; 2,6,6-trimethyl-1-cyclohexene-1-acetaldehyde; benzene, (2-isothiocyanatoethyl)-; 4-methylthiobutyl isothiocyanate; 5-(methylthio)pentanenitrile; propane, 1-isothiocyanato-3-(methylthio)-; isobutyl isothiocyanate; 1-(methylthio)-3-pentanone; 4-methylthio butanenitrile; 2,6-dimethyl-3-methoxymethyl-p-benzoquinone; and 1,3,5-trimethyl-2-(1,3-butadienyl)benzene.
Environmental stress affecting harvest time was found to impact on the antioxidant properties and lipidic profile as quality indexes of cauliflower in a 3-year (2001–2003) study (Scalzo et al. 2007). Late harvested cauliflowers in 2002 were found to have a very different composition profile (increase of dry and fatty matter, polyphenols and antioxidant activity, decrease in ascorbic acid content) and a difference in fatty acid composition related to a relative increase in unsaturated fatty acids with respect to saturated ones.
Major volatile components identified in cauliflower included nonanal, octanol, 3-methylthiopropyl cyanide, 3-methylthiobutyl cyanide, 2-phenylethyl cyanide and 2-phenylethyl isothiocyanate (Buttery et al. 1976). Volatiles emitted during blanching of cauliflowers included thiols, sulphides, polysulphides, aldehydes, isothiocyanates, nitriles, furans, esters, terpenes and carbonyl compounds (Van Langenhove et al. 1991). Aldehydes were the most abundant cauliflower volatiles. Blanching of purple, two white and two green varieties of cauliflower, prior to freezing, reduced total aliphatic and indole glucosinolates (GLS) by 31 and 37 %, respectively (Volden et al. 2009). l-ascorbic acid (L-AA), total phenols (TP), anthocyanins, FRAP (ferric reducing ability) and ORAC (oxygen radical absorbance capacity) were on average reduced by 19, 15, 38, 16 and 28 %, respectively. Long-term freezer storage did not markedly affect total aliphatic and indole GLS in cauliflower. Freezer storage did result in an average L-AA decrease of 24 % for all but the purple cultivar; smaller reductions in the TP levels were also found. Reductions in FRAP and ORAC values occurred towards the end of the storage period; the mean reductions were 15 and 37 %, respectively. Long-term freezer storage did not affect the anthocyanin content and only minor effects were found for the colour parameters.
Seed Phytochemicals
Forty-three compounds, representing more than 99.7 % of Romanesco cauliflower seed oil for the Natalino variety, 41 (99.6 %) for the Campidoglio variety and 32 (99.5 %) for the Navona variety, were identified (Valette et al. 2006). The major compounds were cyanides such as 4-(methylthio)butyl cyanide (61.3, 66.3 and 79.6 %, respectively), 3-(methylthio)propyl cyanide (21.7, 21.6 and 10.7 %) and isothiocyanates such as 4-(methylthio)butyl isothiocyanate (5.3, 4.0 and 6.7 %) for the three oils. The majority of identified volatile compounds were obtained by hydrolysis of glucosinolates. The compounds identified included nonanal; hexanal; benzeneacetaldehyde; phenylethyl alcohol; 6 methyl-5-hepten-2-one; (E,E)-2,4-decadienal; furfural; (E)-2-nonenal; methional; 2-pentyl-furan; indole; 1-hexadecanol; 2-methoxy-4-vinylphenol; 2-furanmethanol; dihydro-5-pentyl-2(3H)-furanone; benzophenone; 1-octadecanol; 4-methoxy-6-(2-propenyl)-1,3-benzodioxole; benzyl nitrile; hexanenitrile; 6-methyl-5-hepten-2-ol; (E,E)-3,5-octadien-2-one; allyl isothiocyanate; benzene, (isothiocyanatomethyl)-; heptanonitrile; 4-isothiocyanato-1-butene; benzenepropanenitrile; isoamyl cyanide; benzene, (2-isothiocyanatoethyl)-; 5-methyl-hexanenitrile; 1-isothiocyanato-3-(methylthio)-propane; isobutyl isothiocyanate; 4-methylpentyl isothiocyanate; 5-methylthiopentyl isothiocyanate; pent-4-enenitrile; 1-isothiocyanato-3-methyl-butane and 4-(methylthio)-butanenitrile.
As seen from the above, cauliflower is low in fat and high in folate, dietary fibre and vitamin C and a good source of vitamin A, calcium, iron, manganese, riboflavin, niacin, thiamin, pantothenic acid and vitamin B6. It has beneficial antioxidant phenolics and flavonoids and glucosinolates and isothiocyanates with anticancer and other positive biological properties.
Antioxidant Activity
Cauliflower by-product extracts showed significant free radical scavenging activity (DPPH and ABTS radicals), ferric reducing ability (FRAP assay) and capacity to inhibit lipid peroxidation (ferric thiocyanate assay) (Llorach et al. 2003a). Furthermore, the antioxidant activity was linearly correlated with the phenolics content. The water and ethanol extraction of cauliflower yielded a phenolic content of 33.8 mg/g freeze-dried extract and 62.1 mg/g freeze-dried extract, respectively. This percentage increased considerably when the extracts were purified yielding a phenolic content of 186 mg/g freeze-dried extract (water extract) and 311.1 mg/g freeze-dried extract (ethanol extract). The results indicated that the cauliflower by-products are a cheap source of antioxidant phenolics.
Cauliflower glucosinolates and their derivatives were found to have significant antioxidant capacity in the oxygen radical absorbance capacity (ORAC) and superoxide radical scavenging activity (SRSA) assays especially glucobrassicin, followed by glucoiberin and gluconapin (Cabello-Hurtado et al. 2012). In contrast the antioxidant activities of the glucosinolates in the 2,2-diphenyl-1-picrylhydrazyl (DPPH) and 2,2′-azino-bis-(3-ethyl-benzothiazoline-6-sulphonic acid) (ABTS) assays were weak. There was no synergy or antagonism between glucosinolates. Breakdown products of glucosinolates were far more active than the native glucosinolates. The highest increases in antioxidant activity were obtained for enzymatic hydrolysis-derived products (EHDP) from progoitrin, sinigrin and glucoraphanin, progoitrin EHDP being the second most active derivative behind glucobrassicin EHDP. Overall, the contribution of glucosinolates to antioxidant activity of cauliflower appeared low.
Anticancer Activity
In-Vitro Studies
Three indole compounds indole-3-acetonitrile, indole-3-carbinol and 3,3′-diindolylmethane were identified from three cruciferous vegetables, Brussels sprouts, cabbage and cauliflower (Loub et al. 1975; Wattenberg 1975). These indoles were found to be naturally occurring inducers of aryl hydrocarbon hydroxylase (Loub et al. 1975) and microsomal mixed function oxidase system and to exert a protective effect against chemical carcinogens (Wattenberg 1975). Besides these indoles, other naturally occurring inducers include flavones, safrole, isosafrole, β-ionone and oxidized sterols (Wattenberg et al. 1976). Thus, the composition of the diet could play a role in inhibiting the neoplastic response to carcinogenic agents.
Indole-3-carbinol (I3C), a secondary plant metabolite produced in vegetables of the Brassica genus, including cabbage, cauliflower and Brussels sprouts, could be both an anti-initiator and a promoter of carcinogenesis (Bjeldanes et al. 1991). Consumption of I3C by humans and rodents could lead to marked increases in activities of cytochrome P-450-dependent monooxygenases and in a variety of phase II drug-metabolizing enzymes. They reported previously that the enzyme-inducing activity of I3C was mediated through a mechanism requiring exposure of the compound to the low-pH environment of the stomach. They found that indolo[3,2-b]carbazole (ICZ), a major acid condensation product generated from I3C in the order of 0.01 % in-vitro and, after oral intubation, in-vivo to be an aromatic hydrocarbon responsiveness-receptor agonist and to induce cytochrome P4501A1 activity in murine hepatoma Hepa 1c1c7 cells. Isothiocyanates and indoles (e.g. indole-3-carbinol) from Brassica vegetables (e.g. broccoli, cauliflower) induce phase I and phase II enzymes responsible for the oxidation, reduction and metabolism of endogenous and exogenous carcinogens (Fowke et al. 2006). Oral administration of indole-3-carbinol (I3C) had been shown to manipulate oestrogen metabolism in humans in a possibly beneficial manner (Brignall 2001). I3C increased the 2/16-hydroxyestrone ratio, a ratio found to be predictive of breast cancer risk in some prospective studies. Animal and in-vitro studies had identified a number of other possibly beneficial effects of I3C and its metabolites, including inhibition of oestrogen binding and modulation of oncogene expression. A chemopreventive effect of I3C had been demonstrated in a number of animal models. Some chemical carcinogenesis models had found a tumour-promoting effect of I3C, however. Ascorbigen, a derivative of indole-3-carbinol may partly mediate the known anticarcinogenic effect of diets rich in Brassicaceae (Wagner and Rimbach 2009). Further, ascorbigen could induce phase I and II enzymes centrally involved in the detoxification of xenobiotics.
Both indole-3-carbinol (I3C) and diindolylmethane (DIM) from Brassica vegetables were found to be partial aryl hydrocarbon (Ah) receptor antagonists in the T47D human breast cancer cell line (Chen et al. 1996). In aryl hydrocarbon (Ah)-responsive T47D human breast cancer cells, I3C and DIM did not induce significantly CYP1A1-dependent ethoxyresorufin O-deethylase (EROD) activity or CYP1A1 mRNA levels at concentrations as high as 125 or 31 μM, respectively. In T47D cells co-treated with 5 nM [3H]TCDD (2,3,7,8-tetrachlorodibenzo-p-dioxin) alone or in combination with 250 μM I3C or 31 μM DIM, there was a 37 and 73 % decrease, respectively, in formation of the nuclear Ah receptor. The more effective inhibition of induced EROD activity by I3C and DIM was due to in-vitro inhibition of enzyme activity. Firestone and Bjeldanes (2003) found that indole-3-carbinol and 3-3′-diindolylmethane antiproliferative signalling pathways controlled cell-cycle gene transcription in human MCF-7 breast cancer cells by regulating nuclear promoter-Sp1 transcription factor interactions.
Following ingestion of Brassica vegetables, indole-3-carbinol (I3C) is converted to a series of oligomeric products that presumably are responsible for the in-vivo effects of I3C; tumour-promoting effects of high doses of I3C may be due to activation of aryl hydrocarbon receptor (AhR)-mediated pathways (Riby et al. 2000). Hong et al. (2002) found that 3,3′-diindolylmethane (DIM), a major in-vivo derivative of the putative anticancer agent indole-3-carbinol, could induce apoptosis in breast cancer cells independent of oestrogen receptor status by a process mediated by the modulated expression of the Bax/Bcl-2 family of apoptotic regulatory factors. Degner et al. (2009) found that co-treatment of MCF-7 breast cancer cells with 3,3′-diindolylmethane (DIM) (10 μmol/l) abrogated the xenobiotic 2,3,7,8 tetrachlorodibenzo(p)dioxin (TCDD)-induced recruitment of the aryl hydrocarbon receptor and acetylated histone H4 to the acetylated histone H4 promoter and the induction of COX-2 mRNA and protein levels. The data suggested that naturally occurring modulators of the aryl hydrocarbon receptor such as DIM may be effective agents for dietary strategies against epigenetic activation of COX-2 expression by aryl hydrocarbon receptor agonists. In another study, 3,3′-diindolylmethane (DIM), a putative anticarcinogenic agent formed in the acidic environment of the stomach following consumption of indole-3-carbinol (I3C), inhibited the proliferation of three human breast cancer cell lines, MCF-7, MDA-MB-231 and SKBr-3 and concomitantly inhibited the expression of transcription factor Sp1 and fatty acid synthase (FAS) but not in nontumorigenic human breast epithelial cell line MCF-10A (Saati and Archer 2011).
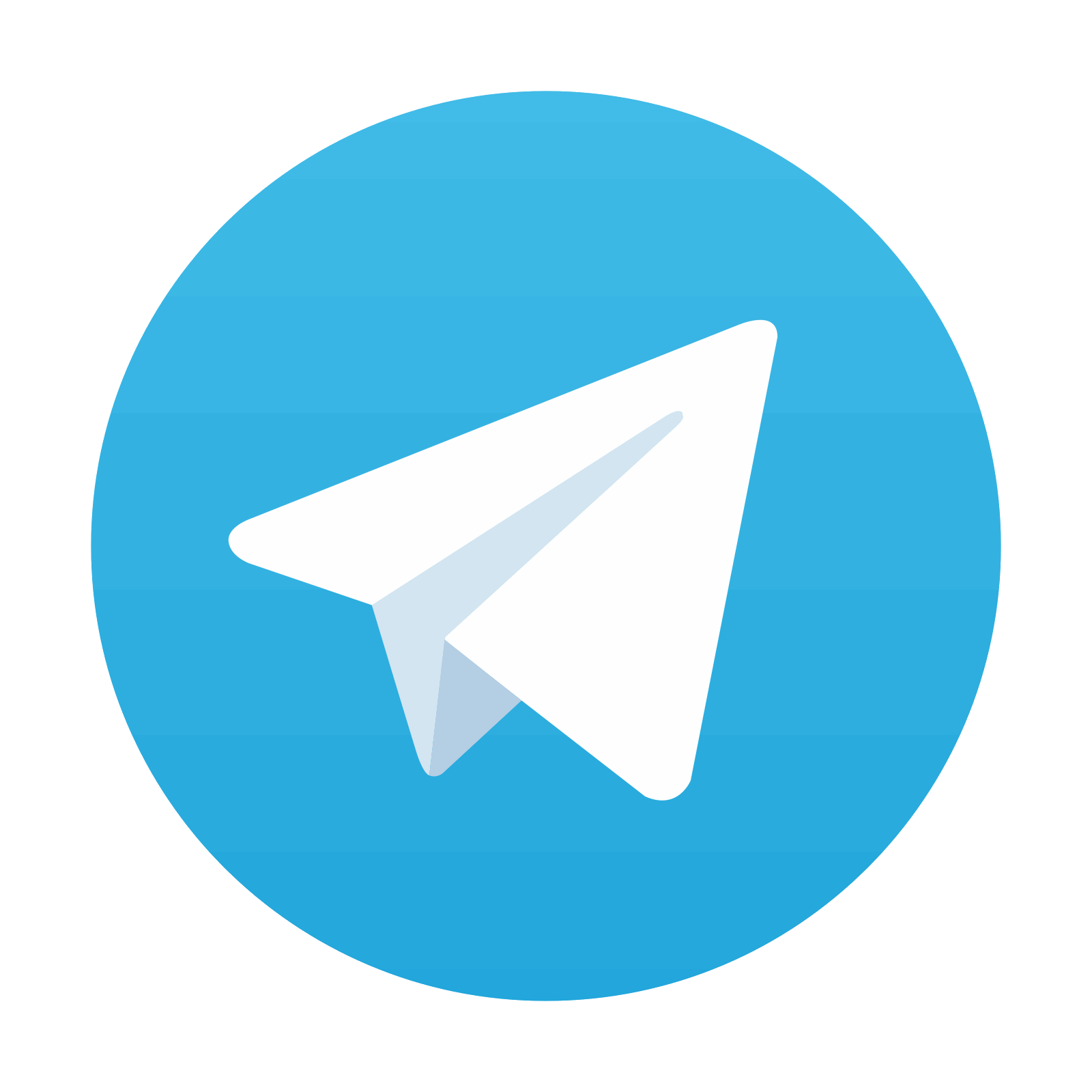
Stay updated, free articles. Join our Telegram channel

Full access? Get Clinical Tree
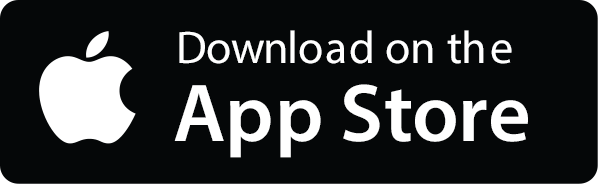
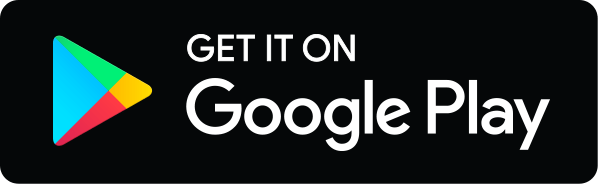
