(1)
Canberra, Aust Capital Terr, Australia
Scientific Name
Calendula officinalis L.
Synonyms
Calendula aurantiaca Kotschy ex Boiss., Calendula eriocarpa DC., Calendula hydruntina (Fiori) Lanza, Calendula officinalis var. prolifera Hort., Calendula prolifera Hort. ex Steud., Calendula × santamariae Font Quer, Calendula sinuata var. aurantiaca (Klotzsch ex Boiss.) Boiss., Caltha officinalis (L.) Moench (nom. illeg.)
Family
Asteraceae
Common/English Names
Bull’s Eye, Calendula, Common Marigold, Cowbloom, Death Flower, Drunkard Gold, English Marigold, Garden Marigold, Gold Bloom, Golden Flower of Mary, Herb of the Sun, Holligold, Hollygold, Marigold, Husband’s Dial, Kingscup, Marybud, Marygold, May Orange, Poet’s Marigold, Poor Man’s Saffron, Pot Marigold, Ruddles, Scotch Marigold, Scottish Marigold, Shining Herb, Summer’s Bride, Sun’s Bride, Water Dragon
Vernacular Names
Albanian: Kalendula Mjekësore
Arabic: Ajamir, Djoumaira
Brazil: Calêndula
Chinese: Chin Chan Hua, Jīn Zhăn Jú
Croatian: Bileć, Ljekoviti Neven, Mesiček, Ognjac, Vridovno Zelje, Zimorod
Czech: Měsíček Lékařský
Danish: Havemorgenfrue, Morgenfrue
Dutch: Goudsbloem, Tuinggoudsbloem
Eastonian: Harilik Saialill
Esperanto: Kalendulo, Kalendulo Kuraca, Orfloro Kuraca
Finnish: Kehäkukka, Tarhakehäkukka
French: Calendule, Fleur De Souci, Fleurs De Tous Les Mois, Gauche-Fer, Soubi, Souci, Souci Des Jardins, Souci Officinal, Yous Les Mois
German: Goldblume, Ringelblume, Ringelrose, Sonnenwende
Hungarian: Kerti Körömvirág, Körömvirág
Iceland: Morgunfrú
India: Genda, Surajmukhee, Zergul (Hindi), Gulsarfi (Punjabi), Sendigai, Sendigai Poo (Tamil), Banti (Telugu), Gul-E-Ashrafi (Urdu)
Italian: Calendola, Calendula, Calta, Fior D’ogni, Fiorrancio, Fiorrancio Coltivato, Fiorrancio Dei Gardine
German: Butterblume, Dotterblume, Echte Ringelblume, Garten-Ringelblume, Gartendotterblume, Goldblume, Ingelblum, Rinderblume, Ringelblume, Ringelrose, Ringula, Sonnenbraut, Sonnenwende, Studentenblume, Totenblume, Warzenkraut, Weckbröseln, Wucherblume
Japanese: Kinsenka, To Kinsenka
Korean: Kumjanhwa
Norwegian: Ringblom
Polish: Nagietek Lekarski
Portuguese: Calêndula, Belas-Noites, Boas-Noites, Maravilhas, Margarida
Russian: Kalendula, Nogot’ki, Nogot’ki Lekarstvennye
Slovašcina: Ognjič Vrtni, Vrtni Ognjič, Zdravilni Ognjič
Slovenian: Nechtík Lekársky, Vrtni Ognjič
Spanish: Botón De Oro,Caldo, Calendula, Cempasúchitl, Corona De Rey, Flamenquilla, Flaminquillo, Flor De Difunto, Flor De Merte, Maravilla, Mejorana, Mercadela, Rosa De Muertos, Virreina
Swedish: Ringblomma, Solsicka, Solsocka
Turkish: Tibbi Nergis
Vietnamese: Cúc Kim Tiền, Hoa Xu Xi, Tâm Tư Cúc, Xu Xi
Welsh: Melyn Mari
Origin/Distribution
The species is a native of the Mediterranean area, but now Calendula has naturalized in many temperate countries and is cultivated as ornamentals in warm temperate and sub-temperate areas.
Agroecology
The plant grows well in full sun and tolerate most soils—acidic, sandy, loamy and clayey soils with pH 4.5–8.3—but does best on well-drained, moist, loamy soil. In temperate areas, seeds are sown in spring for blooms that last throughout the summer and well into the fall.
Edible Plant Parts and Uses
Flowers and leaves are edible (Hedrick 1972; Facciola 1990; Roberts 2000). Fresh petals are chopped and added to salads or used as garnish in dishes. The petals can be used in omelette, curry and custard (Roberts 2000). Dried petals have a more intense flavour and are used as a seasoning in soups, cakes, drinks and baked products. An edible yellow dye is obtained from the flowers and used as colorant for butter, cheese, drinks, rice, soups, confectionery and baked products and also used as a substitute for saffron. Calendula was once known as ‘poor man’s saffron’ as its extract was fed to hens to make their egg yolks golden. An herbal tea can be prepared from the flowers and petals. The leaves can be eaten raw in salads.
Botany
The plant is usually grown as an annual, erect or procumbent and branched, stipitate-glandular, with a strong tap root. Leaves are sessile or shortly petiolate, elliptic, obovate, oblong, oblanceolate to spatulate, 3–12 (−16 cm) by 2–5 cm, with entire margins, apex acute, base sometimes clasping, sparsely arachnose on both surfaces (Plates 1 and 2). Flower heads (capitula) borne singly; involucre campanulate to hemispheric; phyllaries linear-lanceolate, pubescent and in two series; ray florets 15–50 (>100) in 1–3 or more series, functionally female, with yellow to orange, linear to oblanceolate corolla; central disc florets 20–60 (>100), hermaphrodite but functionally male, tubular with campanulate throat, corolla yellow, orange (Plates 2 and 3), reddish or purplish. Achene curved and tuberculate or transversely ridged.




Plate 1
Leaves and a flower bud

Plate 2
Yellow flowers in bloom

Plate 3
Close view of flower heads
Nutritive/Medicinal Properties
Flower Phytochemicals
Carbohydrates
The water soluble polysaccharide fraction obtained from C. officinalis inflorescences were found to contain 84.58 % pectic substances, 29.25 % ash, 9.25 % moisture, 25.77 % acidic sugars, 31.25 % reducing sugars and 4.92 % proteins and to have the following monosaccharide composition comprising glucose, galactose, arabinose, xylose, rhamnose and galacturonic acid (Chushenko et al. 1988). Three homogeneous polysaccharides were isolated from Calendula officinalis flowers (Varljen et al. 1989). All three polysaccharides contained a (1 → 3)-linked β-d–galactan backbone with branching points at C-6. The side chains are composed of short α-Araf(1 → 3)-Araf, α-l-Rhap-(1 → 3)-Araf or simple α-l-Araf units. The carbohydrate composition of Calendula officinalis consisted of free glucose and pectin substances with a low degree of esterification (Khodzhaeva and Turakhozhaev 1993). The monosaccharide composition of the stem comprised rhamnose, galactose and galacturonic acid and the inflorescences have rhamnose, galactose, glucose and galacturonic acid. Pectic substances were also found in the stems and inflorescences.
Terpenoids
From Calendula officinalis flowers, five glycosides of oleanolic acid were isolated and their structures established as 3-glucuronide; 3-(galactosyl-glucuronide); 3-(galactosyl-glucuronide); 17-glucoside; 3-(galactosyl-(glucosyl)-glucuronide); and 3-(galactosyl-(glucosyl)-glucuronide); 17-glucoside (Kasprzyk and Wojciechowski 1967). A number of alcohols representing different types of pentacyclic triterpenes were identified in Calendula officinalis flowers (Kasprzyk and Pyrek 1968). In the group of monohydroxy alcohols, the following were identified: α-amyrin, β-amyrin, taraxasterol and lupeol in addition to previously isolated ψ-taraxasterol. In the group of dihydroxyalcohols, the following were identified: brein and calenduladiol (a new diol of lupeol type) in addition to the previously isolated arnidiol and faradiol. The presence of four other diols of the α-amyrin, β-amyrin and ψ-taraxasterol types was observed. Alcohols of ψ-taraxasterol type, possessing three and four hydroxyl groups, were also isolated as well as a small amount of oleanolic aldehyde. A new triterpene diol, ursodiol, was isolated from dry Calendula officinalis flowers (Sliwowski et al. 1973). Its structure was confirmed as 3,21-di-OH-ursa-12-en. Kasprzyk and Wiłkomirski (1973) isolated a triterpene triol of α-amyrin type from the flowers. A triterpene glycoside, calenduloside F, was isolated from the flowers (Vidal Ollivier et al. 1988).
In Calendula officinalis flowers, triterpene monols were found mainly in the chromoplast fraction (68 % of total) with smaller amounts in the cell debris, microsomal and supernatant fractions; the mitochondrial fraction was almost devoid of these compounds (Adler and Kasprzyk 1976). Triterpene diols were present exclusively in the chromoplast fraction, 98 % in the form of the 3-monoesters and 2 % in the form of diesters. The data suggested that the hydroxylation of the triterpene monols to the corresponding diols proceeded in the chromoplasts and the esterified form of the monols was probably the substrate for this reaction.
From Calendula officinalis flowers, five pentacyclic triterpene trihydroxyalcohols were isolated and identified as olean-12-ene-3β,16β,28-triol, lup-20(29)ene-3β,16β,28-triol, tarax-20-ene-3β,16β,22α-triol, tarax-20-ene-3β,16β,30-triol and ursa-12-ene 3β,16β,21-triol (Wiłkomirski 1985). Gracza (1987) identified the following terpenoids from the flowering heads: menthone, isomenthone, caryophyllene and an epoxide and ketone derivative, pedunculatine, α- and β-ionone, a β-ionone epoxide derivative and dihydroactinidiolide. Triterpenoids found in the flowers included faradiol monoester, monools ψ-taraxasterol, lupeol, taraxasterol and β-amyrin (Della Loggia et al. 1994). Eleven triterpene alcohols helianol, taraxasterol, ψ-taraxasterol, α-amyrin, β-amyrin, lupeol, taraxerol, cycloartenol, 24-methyl-enecycloartanol, tirucalla-7,24-dienol and dammaradienol were isolated from the tubular flowers of Calendula officinalis, Carthamus tinctorius, Cosmos bipinnatus, Chrysanthemum morifolium, Helianthus annuus and Matricaria matricarioides (Akihisa et al. 1996). All the flowers shared a common characteristic feature by containing helianol as the most predominant component (29–86 %) in the triterpene alcohol fractions.
From the 1-butanol-soluble fraction of the methanol flower extract of Calendula officinalis, four new triterpene oligoglycosides, calendasaponins A, B, C and D, were isolated, together with eight known saponins, seven known flavonol glycosides and a known sesquiterpene glucoside (Yoshikawa et al. 2001). They also isolated two new ionone glucosides (officinosides A and B) and two sesquiterpene oligoglycosides (officinosides C and D) from the flowers of Egyptian Calendula officinalis (Marukami et al. 2001). One new triterpenoid of oleanane-series, cornulacic acid acetate (1), along with oleanolic acid acetate was isolated from Calendula officinalis flowers (Naved et al. 2005). The structure of (1) was established as 3β-acetoxy-olean-12-en-27-oic acid and oleanolic acid acetate was characterized as 3β-acetoxy-olean-12-en-28-oic acid.
Ten oleanane-type triterpene glycosides, 1–10, calendulaglycoside A (1), calendulaglycoside A6′-O–n-methyl ester (2), calendulaglycoside A 6′-O–n-butyl ester (3), calendulaglycoside B (4), calendulaglycoside B 6′-O–n-butyl ester (5), calendulaglycoside C (6), calendulaglycoside C 6′-O–n-methyl ester (7), calendulaglycoside C 6′-O–n-butyl ester (8), calenduloside F 6′-O–n-butyl ester (9) and calenduloside G 6′-O–n-methyl ester (10), along with five known flavonol glycosides, 11–15 isorhamnetin-3-O-neohesperidoside, isorhamnetin-3-O-2-rhamnosyl rutinoside, isorhamnetin-3-O-rutinoside,quercetin-3-O-glucoside and quercetin-3-O-rutinoside, were isolated from the flowers of Calendula officinalis (Ukiya et al. 2006).
Lipids
Sterol esters as well triterpene monol and diol esters isolated Calendula officinalis flowers were found to contain as alcohol components all the types of sterols and triterpenic alcohols present in the plant (Wojciechowski et al. 1972). Sterols and triterpene monols were esterified with acetic, lauric, myristic and palmitic acids. Triterpene diols were esterified with lauric, myristic and palmitic acids. The main diol esters were 3-monoesters; diesters were present only in very small amount.
In C. officinalis ligulate flowers, it was shown that all free and ester-bound sterols and triterpene monols in both forms occurred in the chromoplast fraction and in the chromoplast-free fraction, whereas all diols were localized only in the chromoplast fraction (Wilkomirski and Kasprzyk 1979). The compositions of the fatty acids esterifying monols and sterols were similar to those esterifying diols in the chromoplasts. However, the fatty acids esterifying extra-chromoplast monols and sterols were different. This result indicated triterpene monol esters to be substrates for the biosynthesis of 3-monoesters of diols. Faradiol esters, namely, faradiol-3-myristic acid ester, faradiol-3-palmitic acid ester and ψ-taraxasterol, were isolated from C. officinalis flower heads (Zitterl-Eglseer et al. 1997).
The main compounds of lipophilic extracts of flower heads of Calendula officinalis comprised triterpendiol esters, mainly faradiol laurate, faradiol myristate and faradiol palmitate (Zitterl-Eglseer et al. 2001). These faradiol-3-O-monoesters were quantified in different parts of C. officinalis plants, namely, ray florets, disc florets, involucral bracts, receptacles, leaves and seeds. The contents of the esters were highest in ray florets, approximately ten times lower in disc florets than in the ray florets, and approximately ten times lower in involucral bracts than in the disc florets. In the leaves only traces of the esters could be detected, and in the receptacles no esters could be detected at all. Quantification in the seed was not possible using this method because of interfering fatty compounds.
Dichloromethane extract of dried flowers of Calendula officinalis was found to contain eight known bioactive pentacyclic terpenoids and triterpendiol monoesters, namely, faradiol-3-O-palmitate, faradiol-3-O-myristate, faradiol-3-O-laurate, arnidiol-3-O-palmitate, arnidiol-3-O-myristate, arnidiol-3-O-laurate, calenduladiol-3-O-palmitate and calenduladiol-3-O-myristate (Neukirch et al. 2004). Of the ten varieties of C. officinalis investigated, calypso orange florensis produced the highest amounts of the bioactive monoesters, followed by Fiesta Gitana Gelb and may orange florensis. The lipophilic extract from the flowers of calypso orange florensis variety also contained low levels of the newly characterized calenduladiol-3-O-laurate.
Marigold also has oleoresins. The solubility of Marigold (Calendula officinalis) oleoresin in supercritical CO2 (SC-CO2) varied from 4.74 × 10−4 to 17.04 × 10−4 g oleoresin/g CO2 (Danielski et al. 2007). The use of palm oil as cosolvent for SC-CO2 extraction of Marigold flower was found to enhance the yield of lutein fatty acid esters by approximately 16 %, with the most suitable concentration being 10 % w/v palm oil (Palumpitag et al. 2011). Under this condition, approximately 87.2 % recovery of lutein fatty acid esters was obtained after 4 hours extraction at 60 °C and 40 MPa. Furthermore, saponification of the Marigold oleoresin for 3 hours with 2 ml of 40 % (w/v) KOH solution per 1 g of oleoresin resulted in the maximum conversion of lutein esters, giving approximately 157.24.4 mg of free lutein/g oleoresin.
Carotenoids
The carotenoid content was higher in orange varieties of C. officinalis: 276 mg/100 g fresh flowers for Double Esterel Orange variety and 111 mg/100 g fresh flowers for Radio Extra variety (Pintea et al. 2003). All varieties contain the same pigments (xanthophylls) but there were significant differences for the ratio between individual pigments. The main pigments identified were flavoxanthin, lutein, rubixanthin, β-carotene, γ-carotene and lycopene. The great majority of xanthophylls present had a β-ε structure: flavoxanthin, lutein and luteoxanthin. Zeaxanthin (3,3′-dihydroxy-β, β-carotene) was present in small amount, as well as their epoxides: antheraxanthin, mutatoxanthin and auroxanthin. Other carotenoids present included neoxanthin, lactucaxanthin and α-carotene. Orange varieties contained higher amounts of hydrocarbons, 44.5 % of total carotenoid in Double Esterel Orange, while yellow varieties contain mostly oxygenated derivatives, 97 % of total carotenoids in Double Esterel Jaune. In the orange varieties, a preferential biosynthesis of hydrocarbons with ψ-ψ and β-ψ structure and of monoxanthophylls with β-ψ (or α-ψ) structure was noted.
In the petals and pollens of Calendula officinalis, the main carotenoids were flavoxanthin and auroxanthin while the stem and leaves mostly contained lutein and β-carotene (Bakó et al. 2002).
Carotenoids present in C. officinalis cv Alice Orange flowers in percent of total carotenoids (Kishimoto et al. 2005) and in μg/g fresh weight (Kishimoto et al. 2007) were respectively (8′R)-luteoxanthine 11 % 186.6 μg; lutein-5,6-epoxide 1.6 % 27.1 μg; (8R)-lutein-5,8-epoxide (flavoxanthin) 28.5 %, 483.4 μg; (8R,8′R)-auroxanthin 7.1 %, 120.4 μg; (9′Z)-lutein-5,6-epoxide 5 %, 84.8 μg; lutein 2 % 33.9 μg; antheraxanthin 1 % 17.0 μg; (9Z)-lutein 0.6 %, 10.2 μg; (5′Z,9′Z)-rubixanthin 4 %, 67.8 μg; α-carotene 0.8 %, 13.6 μg; β-carotene 3.4 %, 57.7 μg; (5′Z)-rubixanthin 3 %, 50.9 μg; δ-carotene 1.4 %, 23.7 μg; (5Z,9Z,5′Z,9′Z)–lycopene 4.1 %, 69.5 μg; γ-carotene 2 %, 33.9 μg; (5′Z)-γ-carotene 4.4 %, 74.6 μg; (5Z,9Z,5′Z)-lycopene 3.5 %, 59.4 μg; (5Z,9Z)-lycopene 4.1 %, 69.5 μg and (all – E)-lycopene 8.7 %, 147.6 μg. The carotenoid composition in Alice Yellow flowers were (8′R)-luteoxanthin 15.6 %, 195 μg; lutein-5-6-epoxide 3.2 %, 40 μg; (8R)-lutein-5,8-epoxide (flavoxanthin) 42.6 %, 532.5 μg; (8R,8′R)-auroxanthin 10.7 %, 133.7 μg; (9′Z)-lutein-5,6-epoxide 8.5 %, 106.2 μg; lutein 5 %, 62.5 μg; antheraxanthin 2.5 %, 31.2 μg; (9Z)-lutein 1.5 %, 18.7 μg; and β-carotene 1.0 %, 12.5 μg. Total carotenoids in C. officinalis Alice orange was 1696.2 μg/g FW comprising 963.4 μg of yellowish carotenoids and 668.3 μg of reddish carotenoids and total carotenoids in Alice yellow was 1249.9 μg/g FW comprising 119.9 μg of yellowish carotenoids and 1.2 μg of reddish carotenoids (Kishimoto et al. 2007).
Studies showed that solvent had an influence on the stability of carotenoids in oil extracts of Calendula officinalis (Bezbradica et al. 2005). The highest degradation rates were observed in extracts prepared with linoleic acid-rich solvents (sunflower oil, soybean oil and grape seed oil), while the lowest were found in oil with saturated fatty acids (Myritol 312®) and paraffin oil. Studies showed that saline irrigation water decreased the fresh and dry weights of Calendula officinalis flower heads and pigment contents (total flavonoids and total carotenoids) but increased essential oil yield and its main components (α-cadinol, γ- and δ-cadinene) (Khalid and da Silva 2010). Fresh and dry weights of flower heads and essential oil increased towards the full bloom stage of flowering while pigment content, such as total flavonoids and total carotenoids, increased.
Flavonoids
Eight flavonoids were isolated from C. officinalis inflorescence: two aglycones (quercetin, isorhamnetin) and six glycosides (isoquercetin, isorhamnetin 3-O-β-d-glucoside, narcissin, calendoflaside, calendoflavoside and calendoflavobioside) (Komissarenko et al. 1988). Seven flavonol 3-O-glycosides were isolated from the flowers of Calendula officinalis and elucidated as isorhamnetin 3-O-glucoside, rutinoside, neohesperidoside, quercetin glucoside and 2 (G)-rhamnosylrutinoside (Vidal-Ollivier et al. 1989). Bilia et al. (2001) identified narcissin, rutin, isoquercitrin, quercetin-3-O-rutinosylrhamnoside, isorhamnetin-3-O-rutinosylrhamnoside, isorhamnetin-3-O-glucosylglucoside and isorhamnetin-3-O-glucoside in C. officinalis flowers. The triglycoside isorhamnetin-3-O-α-l-rhamnopyranosyl-(1 → 2)-O-[α-l-rhamnopyranosyl-(1 → 6)]-β-d-glucopyranoside together with the already known glycosides isorhamnetin-3-O-β-d-glucopyranoside and isorhamnetin-3-O-α-l-rhamnopyranosyl-(1 → 6)-O-β-d-glucopyranoside (narcissin) was isolated from Calendula officinalis flowers (Masterova et al. 1991). The total content of flavonoids in ligulate ray florets and tubular discflorets inclusively involucre was found to be 0.88 and 0.25 %, respectively.
The following flavonoids isorhamnetin (3′-metoxy-4′,3,5,7-tetrahydroxyflavone), isorhamnetin-3-O-glucoside, rutin, quercetin glucoside, quercetin-neohesperoside and quercetin-2G-rhamnosil-rutinoside were isolated from flowers and found to have good antioxidant activity (Albulescu et al. 2004). Two flavonoids were isolated from the flowers and identified as isorhamnetin 3-O-rutinoside (narcissin) quercetin 3-O-β-d-glucopyranoside (isoquercitrin) (Kurkin and Sharova 2007). Patulitrin (1) and patuletin (2) were found only in Marigold flowers during and after flowering (Guinot et al. 2008). A water-ethanol mixture gave a high extraction efficiency of both flavonoids.
Essential Oil
Crabas et al. (2003) reported that the essential oil of Calendula officinalis obtained from dried flowers in Italy contained methyl hexadecanoate (23.8 %), methyl linoleate (18.6 %), methyl 9,12,15-octadecatrienoate (17.2 %), methyl octadecanoate (4.8 %), methyl tetradecanoate (4.6 %), g-cadinene and cubenol (4.0 %), d-cadinene (3.2 %), a-cadinol (1.8 %) and oplopanone (1.3 %). Essential oil constituents of C. officinalis flower head (French source) and Slovakian source without fertilizer application, respectively, were β-pinene 0.45 %, 0.38 %, α-pinene 2.47 %, 2.18 %, myrcene 0.19 %, 0.29 %, phellandrene 2.76 %, 0.74 %, p-cymene 2.76 %, 2.22 %, limonene 19.16 %, 18.28 %,19.61 %, terpinene 0.98 %, 1.578 %, caryophyllene 0.96 %, 1.06 %, 1,18cineole 0.47 %, 0.66 %, linalool 20.88 %, linalyl acetate 36.5 %, 38.64 %, camphor 1.98 %, 5.63 %, borneol 0.96 %, 1.84 %, carvone 0.43 %, 2.54 %, geraniol 2.66 %, 1.9 %, geranyl acetate 0.17 %, 0.17 % and caryophyllene oxide 0.44 %, 1.05 % (Naguib et al. 2005). In another study, essential oil constituents from Calendula officinalis flowers from steam distillation were identified as α-copaene 0.9 %, α-ionone 1.5 %, α-humulene 1.2 %, geranylacetone 1.6 %, γ-muurolene 2.3 %, β-ionone 3.2 %, ledene 2.3 %, α-muurolene 5.6 %, γ-cadinene 8.9 %, δ-cadinene 22.5 %, α-cadinene 0.9 %, α-calacorene 2.3 %, caryophyllene oxide 0.5 %, copaene-4-α-ol 0.6 %, β-oplopenone 1.7 %, viridiflorol 2.2 %, ledol 1.3 %, 1,10-di-epi-cubenol 1.6 %, epi-α-muurolol 12.9 %, α-cadinol 20.4 % and cadalene 0.8 % (Gazim et al. 2008). Volatile constituents from Calendula officinalis flowers from headspace solid-phase microextraction were β-cyclocitral 2.1 %, α-cubebene 1.8 %, α-copaene 15.1 %, β-cubebene 1.8 %, α-gurjunene 2.7 %, β-caryophyllene 2.7 %, α-ionone 2.3 %, α-humulene 3.9 %, γ-muurolene 5.3 %, β-ionone 3.9 %, α-muurolene 6.2 %, γ-cadinene 25.5 %, δ-cadinene 22.1 % and α-cadinene 2.3 % (Gazim et al. 2008). Volatile constituents from Calendula officinalis flowers from headspace cold finger extraction were α-copaene 18.4 %, β-cubebene 3.7 %, α-gurjunene 4.2 %, β-caryophyllene 8.6 %, α-humulene 3.9 %, γ-muurolene 4.7 %, α-muurolene 5.8 %, γ-cadinene 24.9 %, δ-cadinene 18.6 % and α-cadinene 2.3 % (Gazim et al. 2008).
Twenty-four compounds were identified in the fresh flower oil of C. officinalis, and the yield was 0.09 % (Okoh et al. 2008). Sesquiterpenoids dominated the fresh flower oil. Major components of the fresh flower oil were α-thujene (26.9 %), T-muurolol (24.9 %) and δ-cadinene (13.1 %).
Coumarins
The ethanol extract of the flowers was found to contain coumarins—scopoletin, umbelliferone and esculetin (Derkach et al. 1987).
Terpenoids, Flavonoids and Phenolic Acids
Triterpenoid esters purified from C. officinalis flower heads included faradiol-3-O-laurate, faradiol-3-O-myristate and faradiol-3-O-palmitate (Hamburguer et al. 2003). Accompanying minor compounds of the triterpene ester fraction purified included maniladiol 3-O-laurate, maniladiol-3-O-myristate, ψ-taraxasterol and β-amyrin. Oleanolic acid, β-amyrin, β-amyrin acetate, rutin, narcissin, 3-glucoside of isorhamnetin, quercetin, isoquercitrin, vanillic acid, caffeic acid, chlorogenic acid, protocatechuic acid, p-coumaric acid and syringic acid were identified in the flower extract (Matysik et al. 2005). Ten oleanane-type triterpene glycosides were isolated from the flowers: calendulaglycoside A (1), calendulaglycoside A6′-O–n-methyl ester (2), calendulaglycoside A 6′-O–n-butyl ester (3), calendulaglycoside B (4), calendulaglycoside B 6′-O–n-butyl ester (5), calendulaglycoside C (6), calendulaglycoside C 6′-O–n-methyl ester (7), calendulaglycoside C 6′-O–n-butyl ester (8), calenduloside F 6′-O-n-butyl ester (9) and calenduloside G 6′-O–n-methyl ester (10) (Ukiya et al. 2006).
Miscellaneous Phytochemicals
Eighteen n-paraffins ranging from C18 to C35 were detected in the petals of Calendula officinalis (Komae and Hayashi 1971). The flowers also contained the bitter principle loliolide (calendin) (Willuhn and Westhaus (1987) and a tasteless yellow substance calendulin discovered by Geiger in 1819 (Shoemaker 1891).
Seed Phytochemicals
Fatty acid composition of the seed oil revealed the presence of lauric (3.90 %), myristic (3.58 %), palmitic (14.96 %), stearic (10.13 %), palmitoleic (4.55 %), oleic (16.26 %), linoleic (39.45 %) and linolenic (7.15 %) acids (Saleem et al. 1986). In the seed oil, conjugated acid was present to the extent of 4.5 % whereas the percentage of non-conjugated acid (linolenic acid) was only 2.65 %. The residual meal after the extraction of oil was also studied for its proteins (18 %) and amino acids composition.
The amounts (%) of lipids in C. officinalis seeds were: neutral lipids, 15.7 %; glycolipids (GLs), 0.9 %; and phospholipids (PhLs), 0.6 % (Ul’chenko et al. 1998). The lipid yield of extracts from the flowers (EF) was 17.1 % and from the leaves (EL) 9.3 %. The following classes of lipids were found (% by weight) hydrocarbons, 0.9 %; esters of sterols and triterpenols with fatty acids, 0.5 %; triacylglycerols (TAGs), 20.0 % comprising TAGs-I, 59.0; TAGs, 2–11.8 %; TAGs, 3–4.3 %; free fatty acids, trace; hydroxy-TAGs, 0.8 %; free sterols and free triterpenols, 0.4 %; diacylglycerols and monoacylglycerols, 1.2 %; and unidentified components, 1.1 %. The phospholipid complex of Marigold seeds consisted of eight classes, which may be arranged in order of content by weight as follows: PCs (phosphatidylcholines) > PIs (phosphatidylinositols) > N-acyl-PEs (N-acyl phosphatidylethanolamines) > N-acyl-lyso-PEs (N-acyl-lyso-phosphatidylethanolamines) > lyso-PIs (lyso-phosphatidylinositols) > PSs (phosphatidylserines) > lyso-PCs (lyso-phosphatidylcholines) > PEs (phosphatidylethanolamines). The glycolipids of the seeds were represented by four components forming the following sequence by mass content: SGs (sterylglycosides) > ESGs (ester sterylglycosides) > MGDGs (monogalactosyldiacylglycerols) > DGDGs (digalactosyldiacylglycerols).
The lipid content of seed of 11 genotypes varied between 13.6 and 21.7 g oil/100 g seeds (Dulf et al. 2013). PUFA contents varied between 60.4 and 66.4 %, while saturates comprising mainly palmitic and long chain saturated fatty acids were found in higher amounts in sterol esters (49.3–55.7 % of total fatty acids). Calendic acid [18:3 (8 t, 10 t, 12c) (n−6)] with contents of 51.47–57.63 % of total fatty acids was the predominant polyunsaturated fatty acid (PUFA) followed by linoleic acid [18:2 (n−6)] (28.50–31.86 %), oleic acid [18:1 (n−9)] (4.44–6.25 %) and palmitic acid (16:0) (3.86–4.55 %). Small and very small (or trace) amounts (<2 %) of stearic (18:0), β-calendic [18:3 (8 t, 10 t, 12 t) (n−6)], elaidic [18:1 (9 t) (n−9)], arachidic (20:0), behenic (22:0), gondoic [20:1 (n−9)], α-linolenic [18:3 (n−3)], linoelaidic [18:2 (9 t,12 t) (n−6)], cis-7-hexadecenoic [16:1 (n−9)], palmitoleic [16:1 (n−7)], lauric (12:0), myristic (14:0), pentadecanoic (15:0) and margaric (17:0) acids were also present. Cromack and Smith (1998) reported the seed oil content of around 20 %, of which up to 60 % was calendic acid, a useful industrial feedstock. Chisholm and Hopkins (1967) reported that Calendula could accumulate more than 40 % of calendic acid. Ozgul-Yucel found that Turkish calendula seed oil was characterized by high concentration of linoleic acid (43.5 %) and low content of CLNAs (calendic acid (18.3 %) + β-calendic (11.2 %). Angelini et al. (1997) reported 16–46 % levels of calendic acid in the Italian Pot Marigold seed oils.
Crombie and Holloway (1985) found that linoleic and oleic acids were precursors in the biosynthesis of calendic acid in Marigold (C. officinalis) seeds but not linolenic acid. (9S)-Hydroxyoctadeca-(10E,12Z)-dienoic acid (α-dimorphecolic acid) was isolated and converted into (R/S)-hydroxy- and -hydroperoxy-[9-3H]octadeca-(10E,l2Z)-dienoic acids but neither labelled specimen was converted into calendic acid by Marigold seed homogenate. Also α-dimorphecolic acid, a minor component of Marigold seed oil, was found to be a terminus rather than an intermediate for calendic acid.
Plant and Leaf Phytochemicals
In the flowers, stem and leaves of C. officinalis, 15 amino acids were detected in the free state: alanine, arginine, aspartic acid, asparagine, valine, histidine, glutamic acid, leucine, lysine, proline, serine, tyrosine, threonine, methionine and phenylalanine (Absavoa et al. 1994). Among them, six predominated: arginine, proline, glutamic acid, phenylalanine, lysine and leucine. The leaves contained about 5 % of amino acids, the stems 3.5 % and the flowers 4.5 %. Szakiel et al. (2005) found that Marigold (C. officinalis) synthesized significant amounts of oleanane saponins, found not only in flowers but also in all organs of plant. These glycosides comprised two series of structurally related compounds, that is, derivatives of 3-O-monoglucoside of oleanolic acid (hence named ‘glucosides’) and derivatives of 3-O-monoglucuronide (‘glucuronides’), depending on the first sugar moiety linked to the C-3 hydroxyl group of oleanolic acid, which is either glucose or glucuronic acid. The occurrence of both series of oleanolic acid glycosides occurring in C. officinalis was earlier reported by Kasprzyk and Wojciechowski (1967) and Wojciechowski et al. (1971). ‘Glucuronides’, known as the series I, were designated with letters (F, D, D2, C, B, A) and ‘glucosides’, forming the series II—with Roman numerals from I to VIII. ‘Glucuronides’ were found in relatively large amounts (up to 2 % of the dry mass) in flowers and in considerably lower quantity in green organs of the plant. Ruszkowski et al. (2003) found ‘glucuronides’ in roots of young Marigold plants, while ‘glucosides’ accumulated mainly in roots of grown and senescing plants and also found in green organs of the plant. However, only glycosides I, II, III, VI and VII were found in Marigold shoots. Oleanolic acid and its 3-O-glucuronide derivatives and 3-O-glucoside derivatives were found in vacuoles prepared from protoplasts and cell walls obtained from leaf cells of Calendula officinalis (Szakiel and Kasprzyk 1989). In both cell compartments 37 % of total cellular oleanolic acid accumulated, 0.6 % occurring as free oleanolic acid (only in vacuoles). Glucuronides accounted for 31.1 % (20.7 % in vacuoles and 10.4 % in cell walls) and glucosides for 5.3 % (2.6 % in vacuoles and 2.7 % in cell walls). Szakiel et al. (1995) found that [3-3H]oleanolic acid glycosides formed in the cytosol of C. officinalis leaf cells were transported to the extracellular space in the form of pentaglucoside VI (44 %), whereas glucuronides derived from [3-3H]oleanolic acid 3-O-monoglucuronide (29 %) as well as a part of glucosides (24 %) were transported into the cell walls.
Studies confirmed that plastoquinone occurred only in the chloroplasts, ubiquinone only in the mitochondria and α-tocopherol in both these subfractions of C. officinalis leaves (Janiszowska et al. 1976). In Calendula officinalis leaves the cyclization of squalene to β-amyrin and its further oxidation to oleanolic acid as well as the biosynthesis of all derivatives of oleanolic acid 3-glucoside and some derivatives of oleanolic acid 3-glucuronoside were found to occur in the microsomal fraction (Janiszowska and Kasprzyk 1977). The final metabolites of oleanolic acid 3-glucoside series, that is, pentaglycosides, were translocated from this fraction, one to the cell wall and plasmalemma fraction and the other to the cytosol. The derivatives of oleanolic acid 3-glucoronoside were synthesized partially in other fractions and accumulated in the different membranous structures of the cell.
Polyphenolic compounds (g/kg dry matter) in the aerial Marigold plant parts were determined as follows: chlorogenic acid 0.55 g, 3,5-DCQA (dicaffeoylquinic acid) 0.78, total caffeoyl derivatives 1.33 g, total dihydroxycinnamic acid derivatives 7.54 g, total flavonoids 5.12 g, total dihydroxycinnamic acid derivatives + flavonoids 12.66 g and total polyphenolic compounds 24.97 g (Fraisse et al. 2011).
Fatty acids C12–C22 were found to be components of acylated steryl glucosides in Calendula officinalis (Zdzislaw et al. 1975). As a source of acyl groups for the synthesis of steryl acylglucosides, various phospholipids obtained from the same plant were utilized in the following sequence: phosphatidylinositol greater than phosphatidylethanolamine greater than phosphatidylcholine. It does not utilize triacylglycerols and monogalactosyldiacylglycerols.
In 3-day and 14-day-old seedlings and leaves of Calendula officinalis, the following sterols were identified: cholestanol, campestanol, stigmastanol, cholest-7-en-3-β-ol, 24-methylcholest-7-en-3β-ol, stigmast-7-en-3β-ol, cholesterol, campesterol, sitosterol, 24-methylcholesta-5,22-dien-3β-ol, 24-methylenecholesterol, stigmasterol and clerosterol (Adler and Kasprzyk 1975). Sitosterol was predominant in young and stigmasterol in old tissues. Young tissues contained relatively more campesterol, but in old tissues a C28Δ5,22 diene was present suggesting transformation of campesterol to its Δ5,22 analog, similar to that of sitosterol to stigmasterol. All the identified sterols were present as free compounds and also in the steryl esters, glucosides, acylated glucosides and water-soluble complexes.
Petroleum ether extract of Calendula officinalis leaf showed the presence of fatty acids, and chloroform extracts showed the presence of triterpenes and sterols (Chakraborthy 2010). Flavonoids, carbohydrates, amino acids, and saponins were present in methanol extract, and saponins, phenolic substances, and tannins were present in the water extract of Calendula officinalis.
Thirty and twenty-one compounds were identified in the fresh leaf and dry leaf oils of C. officinalis and the yield was 0.06 and 0.03 %, respectively (Okoh et al. 2008). Sesquiterpenoids dominated the fresh leaves (59.5 %) while the monoterpenes dominated the oil in the dry leaves (70.3 %). The fresh leaf oil was dominated by T-muurolol (40.9 %), α-thujene (19.2 %) and δ-cadinene (11.4 %), while the dry leaves oil was found to be rich in 1,8-cineole (29.4 %), γ-terpinene (11.6 %), δ-cadinene (9.0 %), β-pinene (6.9 %) and α-thujene (6.3 %).
The yield in Calendula officinalis leaf essential oil showed a maximum at the full flowering stage (0.97 %) and a minimum during the pre-flowering stage (0.13 %) (Okoh et al. 2007). The compositions also showed different patterns at different phases of the vegetative cycle. Sesquiterpenes (α-cadinene, α-cadinol, T-muurolol and epi-bicyclosesquiphellandrene) and monoterpenes (limonene, 1,8-cineole and trans-β-ocimene) showed the highest correlations with the age of the plant. Aiming the use of essential oil as a food ingredient, the most interesting stage is the post-flowering period, the essential oil at this time being rich in α-cadinene, α-cadinol, t-muurolol, limonene and 1,8-cineole, with p-cymene present at lower levels. The total yields of the essential oils at the different stages of the vegetative cycle increased with the age of the plant and ranged between 0.13 % (3rd week) and 0.97 % (12th week, flowering); α-cadinene is an important flavouring agent in baked foods, candy and chewing gum and also used as a fragrance in cosmetics and detergents. T-muurolol and α-cadinol are important antimicrobial agents. The essential oil at 12 week was dominated by geraniol (44.5 %), α-cadinol (24.20 %), δ-cadinene (23.8 %), T-muurolol (22.50 %), 1,8-cineole (22.10 %), cadina-1,4-diene (12.20 %), germacrene D (11.50 %), α-cadinene (10.7 %) and calarene (5.70 %). Other minor components included α-pinene (2.90 %), limonene (2.6 %), α-cubebene (1.7 %), α-humulene (1.7 %), β-pinene (1.4 %), nerolidol (1.3 %), sabinene (0.9 %), endobourbonene (1.0 %), β-caryophyllene (0.9 %), α-ylangene (0.8 %), palustron (0.7 %), epi-bicyclosesquiphellandrene (0.5 %), β-selinene (0.3 %), alloaromadendrene (0.2 %), α-bourbonene (0.2 %), muurolene (0.10 %), terpene-4-ol (0.10 %), p-cymene (0.10 %), carvacrol (0.10 %), α-thujene (0.10 %) and α-gurjunene (0.10 %). These compounds occurred in trace amounts: δ-3-carene, nonanal, 3-cyclohexene-1-ol, α-phellandrene, bornyl acetate, sabinyl acetate, α-copaene, β-cubebene, aromadendrene and oplopenone.
Root Phytochemicals
A new series of glycosides of oleanolic acid was found in the roots of old Calendula officinalis plants (Wojciechowski et al. 1971). These compounds were derivatives of 3-glucoside of oleanolic acid and were different, from those previously isolated from the flowers, derivatives of 3-glucuronoside of oleanolic acid. The sugar components of eight representatives of the new series are glucose and galactose in following ratios: in glucoside I, 1:0; in II, 1:1; in III, 1:2; in IV, 2:1; in V, 3:1; in VI, 3:2; in VII, 4:1; and in VIII, 4:1. The sugars in I–VII were attached only in position 3 of oleanolic acid, but in VIII one glucose molecule was joined to 28-carboxyl of oleanolic acid. Glucoside I was identified as 3-monoglucoside and II as 3-(4′-galactosyl)-glucoside of oleanolic acid. Besides these compounds, 6′-methyl ester of 3-glucuronoside of oleanolic acid was also found in the roots.
A monoside 3-O-β-d-glucopyranoside of oleanic acid and a bioside (calenduloside A) with the structure 3-galactosylglucosyloleanolic acid (Vecherko et al. 1969, 1971b) and calenduloside B with the structure O-β-d-galactopyranosyl-(1 → 4)-O-β-d-glucopyranosyl-(1 → 3)-oleanoloyl-(28 → 1)-α-d-glucopyranoside (Vecherko et al. 1971a); oleanolic acid 3-{[galactopyranosido-(1 → 3)] [glucopyranosido–(1 → 2)]-β-d-glucopyranoside} (calenduloside C) and 28-acyl-β-d-glucopyranoside of calenduloside C (calendulososide D) (Vecherko et al. 1975); glucopyranosyl oleanolate 3-O-β-d-glucuronopyranoside (calenduloside F) (Vecherko et al. 1973), oleanolic acid 3-O-β-d-galactopyranosyl-(1 → 3)-β-d-glucuronoside (calenduloside G) and 28-acyl-β-d-glucopyranoside of calenduloside G (calenduloside H) (Vecherko et al. 1974) were isolated from the roots of C. officinalis. Calenduloside B, trioside of oleanolic acid, was isolated from the roots (Iatsyno et al. 1978).
Chemical studies have revealed the presence of various classes of compounds, the main being triterpenoids, flavonoids, coumarins, quinones, volatile oil, carotenoids and amino acids in C. officinalis plant parts (Muley et al. 2009; Khalid and da Silva 2012). The extract of this plant as well as pure compounds isolated from it had been demonstrated to possess multifold pharmacological activities: antioxidant, antiinflammatory, antiedematous, immunostimulant, anticancer, lymphocyte and wound healing, hepatoprotective, antibacterial and antifungal, anti-HIV, spasmolytic and spasmogenic, cytotoxic, genotoxic and antigenotoxic, inhibition of heart rate and antiviral, among others.
Antioxidant Activity
Superoxide radicals and hydroxyl radicals were observed in decreasing concentrations in the presence of increasing concentrations of C. officinalis butanolic fraction with IC50 values of 1 and 0.5 mg/ml, respectively, suggesting a possible free radical scavenging effect (Cordova et al. 2002). Lipid peroxidation in liver microsomes induced by Fe2+/ascorbate was 100 % inhibited by 0.5 mg/ml of the fraction (IC50 = 0.15 mg/ml). Its total reactive antioxidant potential (TRAP) (in μM Trolox equivalents) was 368.14 and its total antioxidant reactivity (TAR) was calculated to be 249.19 μM. The results suggested the butanolic fraction of C. officinalis to have significant free radical scavenging and antioxidant activity.
The methanol and water extracts of wild Marigold, Calendula arvensis (GWM) and cultivated Marigold, Calendula officinalis (CM), in a concentration range of 0.10–0.90 mg/ml, scavenged all types of investigated radicals in dependence on their applied concentrations (Ćetković et al. 2004). Generally, CM extracts possessed higher scavenging and antioxidant activity than GMW extracts, while methanol extracts exhibited lower activities than water extracts. Water extracts of CM had the best antioxidant properties; 0.75 mg/ml extracts completely eliminated hydroxyl radical, which was generated in the Fenton system. The same concentration of this extract scavenged 92 % DPPH and 95 % peroxyl radical during lipid peroxidation. Antioxidant properties were in correlation with the contents of total phenolic compounds (14.49–57.47 mg/g) and flavonoids (5.26–18.62 mg/g) in extracts. The electron spin resonance (ESR) spectroscopy data demonstrated that methanol and water extracts of CM possessed similar free radicals scavenging and antioxidative activity as synthetic antioxidants BHA. Total antioxidant capacity (%) (DPPH scavenging activity) of C. officinalis aerial plant parts was 1.52 % and contribution from the main caffeoyl derivatives was as follows: chlorogenic acid 3.15 %, 3,5-DCQA (dicaffeoylquinic acid) 6.90 % and total caffeoyl derivatives 10.05 % (Fraisse et al. 2011).
Calendula officinalis flower top extract was found to scavenge superoxide radicals generated by photoreduction of riboflavin and hydroxyl radicals generated by Fenton reaction and inhibited in-vitro lipid peroxidation (Preethi et al. 2006). Concentrations needed for 50 % inhibition (IC50) were 500, 480 and 2,000 mg/ml, respectively. The extract scavenged ABTS radicals and DPPH radicals with IC50 of 6.5 and 100 mg/ml, respectively. The extract also scavenged nitric oxide (IC50 = 575 mg/ml). Oral administration of Calendula extract inhibited superoxide generation in macrophages in vivo by 12.6 and 38.7 % at doses of 100 and 250 mg/kg body weight. Oral administration of the extract to mice for 1 month significantly increased catalase activity and produced significant increase in glutathione levels in the blood and liver. Glutathione reductase was found to be increased, whereas glutathione peroxidase was found to be decreased after extract administration. The results indicated Calendula officinalis possessed significant antioxidant activity in-vitro and in-vivo.
The propylene glycol extract of Calendula officinalis exerted its anti-ROS (reactive oxygen species) and anti-RNS (reactive nitrogen species) activity in a concentration-dependent manner during polymorphonuclear leukocytes burst, with significant effects being observed at even very low concentrations: 0.20 μg/ml without l-arginine, 0.10 μg/ml when l-arginine was added to the test with phorbol 12-myristate 13-acetate and 0.05 μg/ml when it was added to the test with N-formyl-methionyl-leucyl-phenylalanine (Braga et al. 2009). Electron paramagnetic resonance (EPR) spectroscopy confirmed these findings, 0.20 μg/ml being the lowest concentration of C. officinalis extract that significantly reduced 2,2-diphenyl-1-picrylhydrazyl (DPPH). Calendula officinalis propylene glycol extracts were found to have protective effect against oxidative DNA damage and lipid peroxidation induced by high polyunsaturated fatty acid (PUFA) intake in young growing pigs (Frankic et al. 2009). It elicited a numerical trend towards the reduction of plasma malondialdehyde and urinary isoprostane (iPF2α-VI) excretion. Its effect was comparable with that of vitamin E. The extract from flower tops showed less antioxidant potential than the extract from petals. They concluded that the amount of C. officinalis extracts proposed for internal use by traditional medicine protected the organism against DNA damage induced by high PUFA intake.
Methanol enabled more efficient extraction of flavonoids from C. officinalis flowers than the other solvents isopranol and ethanol tested (Butnariu and Coradini 2012). From cv Petran and cv Plamen flowers, methanol extracted, respectively, 2.04 and 2.142 mg/100 g FW of carotene pigments (lycopene, lutein), 10.358 and 5.222 mg/100 g FW of total photosynthetic pigments (chlorophyll a, b), total flavonoid content 96.17 and 90.37 mg quercetin equivalent (QE)/100 ml and total phenolic content of 134 and 153.23 mg GAE/100 ml. The highest antioxidant activity correlated to the polyphenol content was obtained for extracts prepared using methanol. For the methanol extract of cv Petran and cv Plamen, the DPPH radical scavenging activity was2.64 and 2.97 mmol Trolox/g and the ferric reducing antioxidant power (FRAP) was 0.29 and 1.55 mmol Fe2+/g.
Studies by Ozkol et al. (2012) showed that administration of Calendula officinalis extract protected rats against subacute cigarette smoke-induced cell injury. Marigold extract decreased the elevated levels of malondialdehyde, reduced glutathione and protein carbonyl caused by cigarette smoke. Further, Marigold extract increased the diminished levels of glutathione peroxidase (GPx), superoxide dismutase activities and β-carotene and vitamins A and C caused by cigarette smoke.
Bernatoniene et al. (2011) confirmed dry Calendula officinalis extract to be an effective scavenger of H2O2 radicals in in-vitro studies with the mitochondria of rat cardiac muscles. Calendula extract incorporated into hydrophilic cream containing complex emulsifier provided significant antioxidant effect due to the content of carotenoids, polyphenols and flavonoids and good emulsion quality. Cream with the best properties (0.9 % of Calendula extract) contained 0.73 mg/100 g of total carotenoids expressed as β-carotene.
Anticancer/Antiproliferative Activities
Laser Activated Calendula Extract (LACE) showed a potent in-vitro inhibition (70–100 %) of tumour cell proliferation when tested on a wide variety of human (leukaemias, melanomas, fibrosarcomas and cancers of the breast, prostate, cervix, lung, pancreas and colorectal) and murine tumour cell lines (Jiménez-Medina et al. 2006). Mechanisms of inhibition were identified as cell cycle arrest in G0/G1 phase and caspase-3-induced apoptosis. In contrast, the same extract showed an opposite effect when tested on human peripheral blood lymphocyte (PBL) and NKL (natural killer) cell line, in which in-vitro induction of proliferation and activation of these cells was observed. The intraperitoneal injection or oral administration of LACE in nude mice inhibited in-vivo tumour growth of Ando-2 melanoma cells and prolonged the survival day of the mice. Two triterpene glycosides from the flowers, calenduloside F 6′-O–n-butyl ester (9) and calenduloside G 6′-O–n-methyl ester, exhibited potent cytotoxic effects against colon cancer, leukaemia and melanoma cells (Ukiya et al. 2006).
Simultaneous administration of C. officinalis flower extract to tumour-bearing male C57BL/6 mice reduced the lung B16F-10 melanoma tumour nodules by 74 % with 43.3 % increase in life span (Preethi et al. 2010). Elevated levels of hydroxyproline, uronic acid, hexosamine, serum sialic acid and γ-glutamyl transpeptidase in the metastatic controls were significantly lowered in the C. officinalis-treated animals. The extract also inhibited expression of MMP-2, MMP-9, prolyl hydroxylase and lysyl oxidase and activated TIMP-1 and TIMP-2 and downregulated proinflammatory cytokines. The results indicated antimetastatic effects of Calendula officinalis flowers through the inhibition of key enzymes were involved in processes of metastasis.
Studies demonstrated that chamomile and Marigold (Calendula officinalis) tea exerted selective dose-dependent cytotoxic action against target cancer cells; cytotoxicity of Marigold tea was higher than chamomile (Matić et al. 2013). However, the cytotoxic effect of chamomile tea was very weak to healthy peripheral blood mononuclear cells (PBMC), while the effect of Marigold tea on PBMC was more pronounced. Marigold tea exerted highly selective antitumor effect especially to melanoma Fem-x cells in comparison to the action to normal healthy PBMC. Chemical analyses showed that dominant phenolic compounds in examined infusions and decoctions were flavonoid glycosides and hydroxycinnamic acid derivatives. Ethanol extracts of C. officinalis and other Asteraceae species were found to have antileukemic properties and to induce J-45.01 human acute T leukaemia cell death via apoptosis. The correlation between antileukemic activity and total polyphenol content was determined (Wegiera et al. 2012).
Three major flavonoid fractions separated from C. officinalis flower methanol extract did not exhibit inhibitory effect on the parent and tamoxifen-resistant T47D human breast cancer (Ostad et al. 2004). Quercetin increased cell proliferation of the resistant T47D cells in the presence of tamoxifen but no effect was detected by using quercetin itself. Also it was found that isorhamnetin did not have any proliferative or antiproliferative activity on the both cell lines.
Wound Healing Activity
Studies on surgically induced skin wound in Wistar albino rats showed that application of % unguentum containing fractions C1 and C5, isolated from Calendula officinalis flowers of in combination with allantoin, significantly stimulated physiological skin regeneration and epithelialization (Klouchek-Popova et al. 1982).
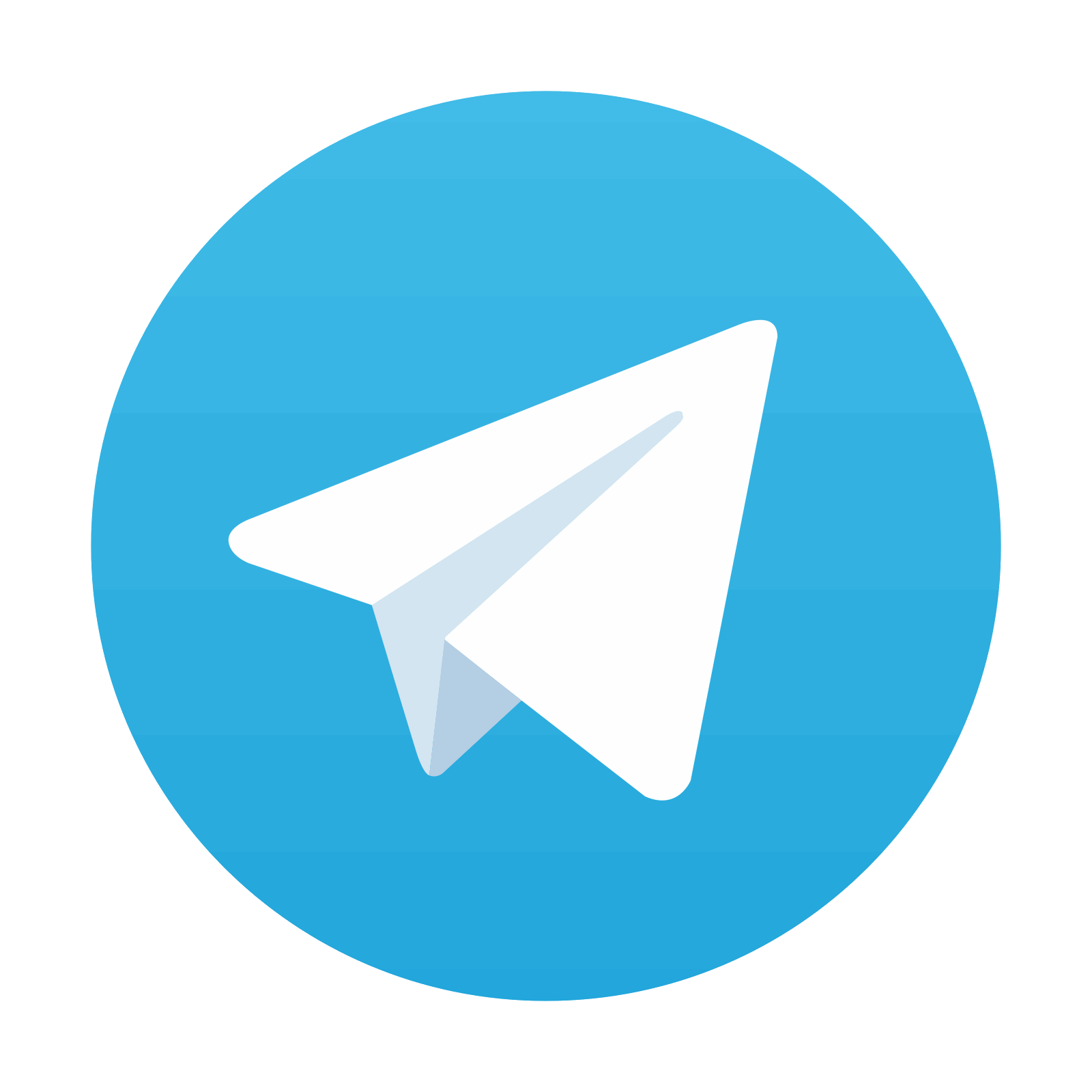
Stay updated, free articles. Join our Telegram channel

Full access? Get Clinical Tree
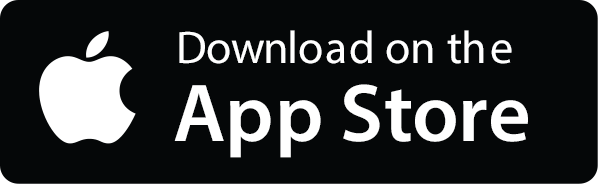
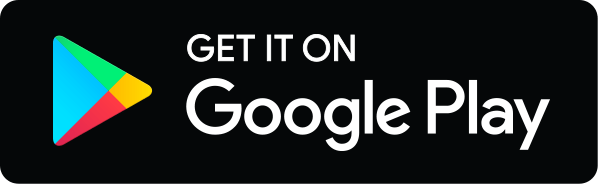