Nutrition in Burn Injury1
Marc G. Jeschke
Celeste C. Finnerty
Rachael A. Harrison
David N. Herndon
1Abbreviations: EN, enteral nutrition; GLN, glutamine; IGF, insulinlike growth factor; IGFBP, insulinlike growth factor-binding protein; LBM, lean body mass; MREE, measured resting energy expenditure; PN, parenteral nutrition; PPAR, peroxisome proliferator-activated receptor; rhGH, recombinant human growth hormone; TBSA, total body surface area.
Severe burn injury often leads to a devastating and persistent hypermetabolic response. This response is associated with 10-fold increases in plasma catecholamines, cortisol, and inflammatory mediators that lead to whole body catabolism and, in particular, muscle protein wasting. Failure to satisfy demanding energy and protein requirements after burn injury may enhance susceptibility to infection, compromise wound healing, induce multiple organ dysfunction, and increase mortality. Optimal nutritional support is critical to ensuring adequate burn care, attenuating the hypermetabolic response, favoring wound healing, minimizing catabolism, and reducing morbidity and mortality. The recommendations and guidelines presented in this chapter are based on prospective trials, retrospective analyses, and expert opinions given by burn practitioners.
POSTBURN CATABOLIC AND HYPERMETABOLIC RESPONSE
Severe burn injury triggers a hypermetabolic response that is profound and can persist for 2 years after injury (1, 2). Characteristics of this response include the following: supraphysiologic metabolic rates, hyperdynamic circulation, aberrant catabolism of bone and muscle, susceptibility to infection, insulin resistance, and growth retardation in children (1, 2, 3, 4, 5). Furthermore, this response has two phases. The first phase, known as the ebb phase, is characterized by reduced tissue perfusion and metabolism immediately following the injury (6). The flow phase quickly ensues, in which patients experience elevated metabolic rates and hyperdynamic circulation. If not properly treated, the flow phase induces physiologic exhaustion, and the insult then leads to death (7, 8, 9, 10).
The hypermetabolic flow phase is characterized by multiple alterations. These include the following: disruption of liver function; insulin resistance; considerable elevations in glycolysis, lipolysis, and proteolysis; and losses in lean body mass (LBM) and total body mass (11, 12, 13, 14, 15, 16, 17). The consequences of sustaining significant losses in LBM resulting from chronic illness or hypermetabolism can be alarming. A 10% loss is associated with compromised immune function, a 20% loss with reduced wound healing, a 30% loss with a greater incidence of pressure sores and pneumonia, and a 40% loss with death (18).
The postburn catabolic response is primarily mediated by catecholamines, corticosteroids, and inflammatory cytokines (19). Increases (10- to 50-fold) in plasma levels of catecholamines and glucocorticoids persist for up to 1 year after burn (20, 21). Burn injury triggers immediate alterations in production of hormones, inflammatory cytokines, acute phase proteins, and constitutive proteins. These factors do not return to normal levels during the acute hospital stay (20), and some factors have abnormal concentrations several years after injury (22).
Net loss of protein leads not only to LBM loss (bringing its own aforementioned problems) but also to serious muscle wasting that reduces strength and prevents complete rehabilitation (23, 24). This protein loss is a direct result of hypermetabolism, may persist for as long as 9 months after a critical burn, and often produces whole-body and cross-leg nitrogen balances that are significantly negative (23, 25, 26). Severe burn injuries induce nitrogen losses as high as 25 g/m2 total body surface area (TBSA) per day (26, 27). Without intervention, lethal catabolism becomes imminent in less than 30 days (27). Furthermore, in young burned children, protein loss leads to pronounced growth retardation for more than 1 year after injury (3).
Data obtained by measuring stable isotopes indicate significant derangements of key energy consumption pathways (i.e., gluconeogenesis, production of urea, and protein turnover). Studies also have shown that glycolytic-gluconeogenic cycling may increase as much as 250% during the postburn hypermetabolic response, and this increase is accompanied by an even greater elevation (450%) in cycling of triglyceride fatty acids (5). Together, these events augment circulation of glucogenic precursors, gluconeogenesis, and glycogenolysis and lead to postreceptor insulin resistance and hyperglycemia (4). The delivery of glucose to peripheral tissues is elevated by as much as threefold and is accompanied by limited glucose oxidation, leading to elevated blood glucose levels. A portion of glucose is supplied to the burn wound so that inflammatory cells, endothelial cells, and fibroblasts can carry out anaerobic metabolism (28, 29). During this hyperinsulinemic state, gluconeogenesis in the liver should be suppressed but is not, most likely because of hepatic insulin resistance. However, the resultant anaerobic glucose oxidation produces lactate, which is used to produce more glucose in the liver via inappropriately regulated gluconeogenesis and to continue the hyperglycemia cycle (26).
Serum levels of insulin and glucose are considerably elevated during the acute hospital stay (glucose because of insulin resistance) (20). The increase in available glucose provides energy to mitigate protein breakdown after severe burn injury (20, 30, 31, 32). However, studies performed in critically ill patients have shown that these high glucose levels are linked to poor outcomes if they are left untreated (30, 33, 34).
Catabolic hormones play a major role in metabolic dysfunction after burn by countering the anabolic actions of insulin and, in doing so, inducing proteolysis, lipolysis, and hyperglycemia (19). To meet the considerable energy needs during hypermetabolism, the body rapidly (albeit inefficiently) uses proteins and lipids after large burns (35, 36, 37). The major source of fuel then becomes skeletal muscle, with all the expected complications associated with LBM loss.
Metabolism and catabolism also can be increased by other perturbations occurring in the severely burned patient. For example, patients who develop sepsis exhibit rates of protein catabolism and metabolism that can be 40% higher than rates in patients with similar-sized burns without sepsis (1, 38). Patients in this catabolic state are more likely to develop sepsis because of compromised immune function, thus creating a vicious cycle. These situations are complicated by multidrug-resistant organisms, which can cause infections associated with sepsis and death (39, 40). In response to burn wounds and burn wound infections, inflammatory cells anaerobically metabolize glucose to pyruvate and lactate (41). These substrates are returned to the liver for gluconeogenesis, provide energy for leukocytes and fibroblasts in the burn wound, and contribute to hyperglycemia, as previously mentioned (28, 42).
ATTENUATION OF THE HYPERMETABOLIC RESPONSE
Nonpharmacologic Modalities
Nutritional Support of Severely Burned Patients
Determinants of successful initial burn treatment are early aggressive resuscitation (including nutrition), control of infection, and early closure of the burn wound. In general, the primary goal of nutritional support is to provide an adequate energy supply and the nutrients necessary to support life and function. Dietary nutrients must be ingested, digested, absorbed, and processed before the garnered substrates are used, stored, or expended for energy. Aggressive, early enteral feeding provides maximal benefits to the patient by alleviating catabolism and improving outcomes (43, 44). In contrast, the use of oral alimentation alone in patients with burns covering 40% of the TBSA resulted in a 25% decrease in preadmission body weight within 21 days after injury (13). However, overcompensation in the form of excess calories or protein is ineffective and is associated with increased complications such as hyperglycemia, carbon dioxide retention, and azotemia (45). Thus, the primary goal of nutritional support is to meet the unusual metabolic needs of severely burned patients adequately, to promote wound healing, increase resistance to infection, and attenuate the persistent loss of muscle protein that is characteristic of burn injury while avoiding exacerbation of existing complications.
Current clinical practice is to administer nutrition enterally whenever possible, given the strong evidence provided by preclinical and clinical studies, as well as by clinical comparison of oral feedings with gastric or small bowel tube feedings (25, 44). Enteral nutrition (EN), in particular, was shown to decrease bacteremia (related to translocation of bacteria from the intestines), reduce sepsis, maintain motility of the gut, and preserve first-pass nutrient supply to the liver in an animal model of severe burn injury (43). In patients in whom EN is not applicable (e.g., those with prolonged ileus or intolerance to EN), parenteral nutrition (PN) should be used to maintain appropriate macronutrient and micronutrient intake (see the chapters on EN and PN). PN remains of critical importance in patients with burns in whom appropriate dietary support cannot be achieved or in those whose total caloric requirements cannot be fully supplied by EN alone.
In addition to the nutritional route of administration, a major determinant of outcome for severely burned patients is timing, because any delays in resuscitation lead to poorer outcomes (46). In the short term, the significant gut mucosal damage present after burn injury leads to increased bacterial translocation secondary to abnormal
gut barrier function and a decreased ability to absorb nutrients (47, 48). Accordingly, optimal nutritional support for the severely burned patient is best accomplished by early (within 12 hours after injury) initiation of EN (49). Multiple studies in animal models supported this recommendation and demonstrated that early institution of EN could significantly modulate the hypermetabolic responses to severe burns, as described earlier (43, 44). Specifically, these studies showed that by 2 weeks after burn, animals given early continuous EN (by tube, within 2 hours after burn) had significantly lower metabolic rates than animals fed 3 days after burn; this finding points to the benefits of delivering feedings into the gut lumen as soon as possible (43). Early EN significantly reduced catecholamine levels and supported gut mucosal integrity in these models (50).
gut barrier function and a decreased ability to absorb nutrients (47, 48). Accordingly, optimal nutritional support for the severely burned patient is best accomplished by early (within 12 hours after injury) initiation of EN (49). Multiple studies in animal models supported this recommendation and demonstrated that early institution of EN could significantly modulate the hypermetabolic responses to severe burns, as described earlier (43, 44). Specifically, these studies showed that by 2 weeks after burn, animals given early continuous EN (by tube, within 2 hours after burn) had significantly lower metabolic rates than animals fed 3 days after burn; this finding points to the benefits of delivering feedings into the gut lumen as soon as possible (43). Early EN significantly reduced catecholamine levels and supported gut mucosal integrity in these models (50).
In human studies, early continuous EN delivered appropriate calculated caloric requirements (based on the resting energy expenditure) by postburn day 3, attenuated the hypermetabolic response, and significantly decreased circulating levels of catecholamines, cortisol, and glucagon (50, 51). Intestinal hypoperfusion (ileus) secondary to delays in resuscitation can be reversed by reperfusion or adequate resuscitation. Postburn ileus spares the small bowel and primarily affects the stomach and colon (52). Patients with severe burn injury can be safely enterally fed in the duodenum or jejunum within 6 hours after burn, whether or not they have total gastroduodenal function (53). Thus, nasojejunal or nasoduodenal feeding must be administered early to facilitate the full resuscitation of the severely burned patient.
Nutritional Requirements
Currently, resting energy requirements of burned patients are commonly estimated using equations that incorporate body mass, age, and gender. Traditionally, the performance of these equations has been weighed against measured resting energy expenditure (MREE) (obtained through indirect calorimetry), even though the accuracy and precision of MREE is not well established in burns. Furthermore, investigations have shown that MREE requires a correcting factor of +20% to 40% to maintain adequate body weight (54, 55). Validation and agreement analysis of formulas such as the Harris-Benedict, Schofield HW, Curreri, and World Health Organization formulas have shown that although these formulas are based on patient-specific factors such as age, gender, weight, and burn size, they may significantly overestimate caloric requirements in patients with burns, thus increasing the risk of overfeeding and its subsequent deleterious effects (54, 56). After considering the significant expense of acquiring and maintaining the equipment for bedside indirect calorimetry, more researchers and facilities have sought to identify new and more accurate equations to ensure that the equipment is useful and beneficial. The adapted Toronto equation may be one such equation because the calculated results very closely matched the MREEs of 10 adult patients (56). The hope is that, as further testing of these formulas and indirect calorimetry itself continues, the ideal method to quantify the required nutritional support in burn adults and children will be refined (2, 57, 58). Although imperfect, measurement of resting energy expenditures using the current method of indirect calorimetry can still provide a value close to actual caloric requirements (2, 54, 57). Goran et al (57) found that feeding patients with 1.2 times MREE preserves body weight, with a 10% loss in LBM and gains in fat deposition (54, 58). This finding further underscores the need for improvement in meeting nutritional needs because preservation of LBM should be the primary goal of nutritional support in patients with severe burns.
The major energy source for patients with burns should be carbohydrates, which serve as fuel during wound healing, thereby sparing protein from oxidation for energy and allowing the protein to be more appropriately used (e.g., protein synthesis by the liver, for wound healing). Critically ill burned patients are estimated to have caloric requirements that far exceed the body’s ability to assimilate glucose, which has been reported to be 5 mg/ kg/minute or approximately 7 g/kg/day (2240 kcal for an 80-kg man) (59, 60, 61). However, providing a limited amount of dietary fat diminishes the need for carbohydrates and ameliorates glucose tolerance.
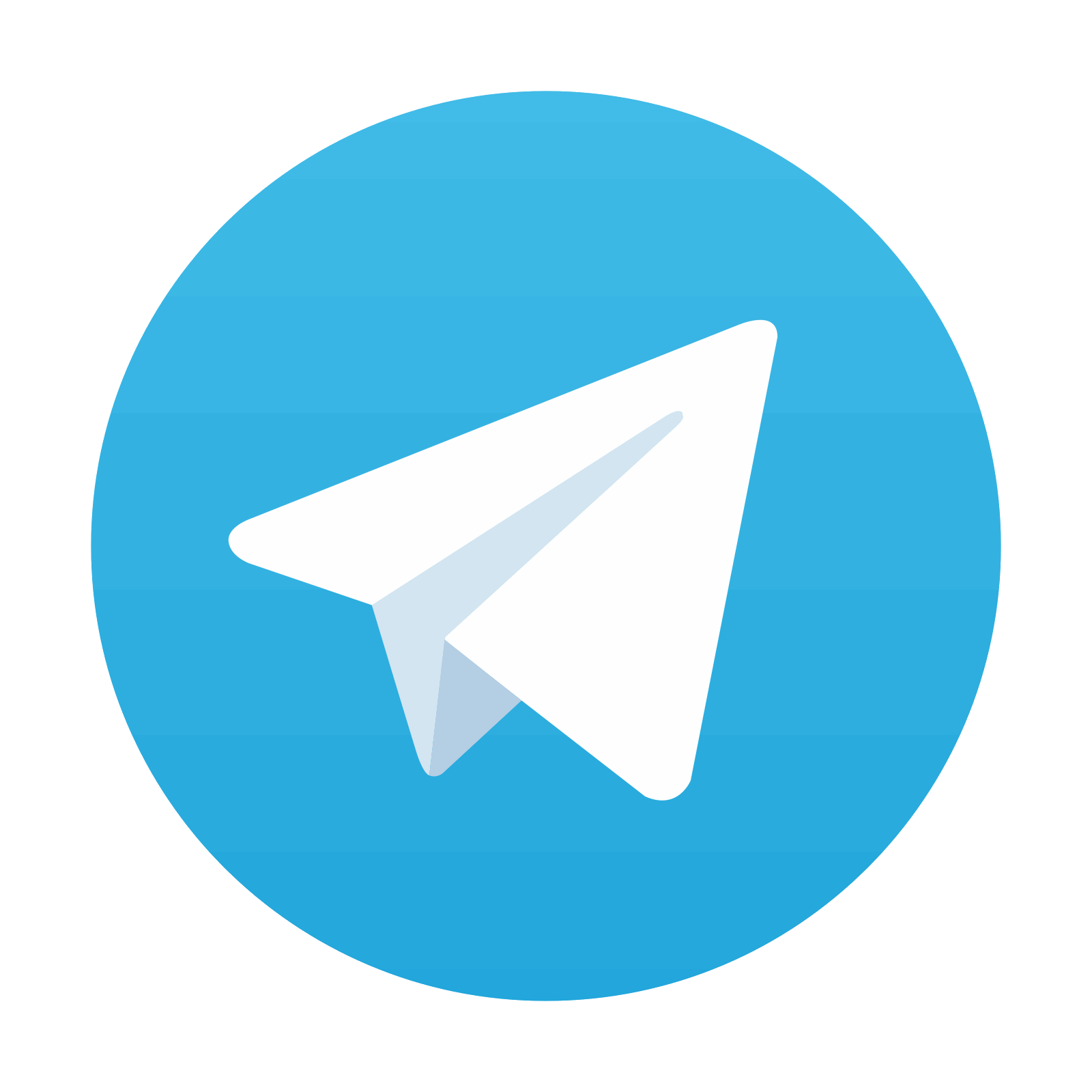
Stay updated, free articles. Join our Telegram channel

Full access? Get Clinical Tree
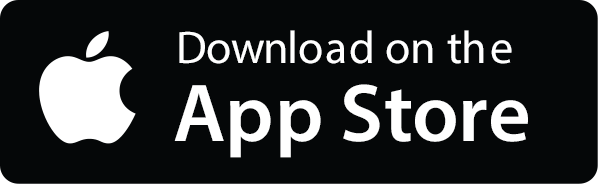
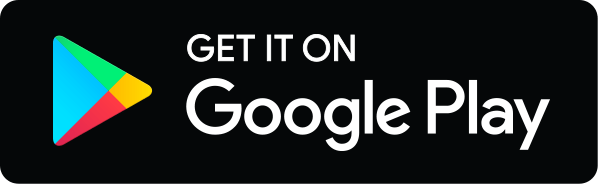
