Nutrition and Infectious Diseases1
Alice M. Tang
Ellen Smit
Richard D. Semba
1Abbreviations: AIDS, acquired immunodeficiency syndrome; ARI, acute respiratory infection; DALY, disability-adjusted life year; HAART, highly active antiretroviral therapy; HIV, human immunodeficiency virus; IFN, interferon; IL, interleukin; MHC, major histocompatibility complex; NF-κ, nuclear factor-κB; TB, tuberculosis; TNF, tumor necrosis factor; UNICEF, United Nations Children’s Fund; VDR, vitamin D receptor; WHO, World Health Organization.
HISTORICAL OVERVIEW
The relationship between nutrition and infectious diseases was largely characterized in the twentieth century. In the early part of the century, the idea of certain “substances,” “accessory food factors,” or “vitamines” essential for health emerged (1, 2, 3); and knowledge of vitamins and deficiency diseases grew rapidly in the period following 1912 (4). The study of nutrition and infectious diseases was facilitated by developments in immunology, such as the description of humoral antibodies, serologic tests for the diagnosis of infectious diseases, and assays for measurements of immunologic protection (5). By the late 1930s and early 1940s, it became generally accepted that some dietary deficiencies could increase the risk of infectious diseases (6, 7, 8). With fortification of foods, improvement in diet, and a general increase in the standard of living, micronutrient deficiencies declined and became less of a public health problem in developed countries.
In 1968, a World Health Organization (WHO) expert committee reviewed the interactions between nutrition and infection (9) and concluded the following:
Infections are likely to have more serious consequences among persons with clinical or subclinical malnutrition, and infectious diseases have the capacity to turn borderline nutritional deficiencies into severe malnutrition. In this way, malnutrition and infection can be mutually aggravating and produce more serious consequences for the patient than would be expected from a summation of the independent effects of the two.
This work provided the foundation for research on nutrition and infection that has followed to the present, much of which has taken place in developing countries, where micronutrient deficiencies are more prevalent.
GENERAL PRINCIPLES
Infection with a pathogen usually triggers a series of responses; these vary depending on the infectious agent. Response to bacterial infection commences with the release of microbial products such as lipopolysaccharides and peptidoglycans from bacterial cell walls, bacterial DNA, and exotoxins; viral infections start with the release of viral double-stranded RNA and viral glycoproteins. Depending on the type of pathogen and its ability to evade the immune response, the location of the infection, host immune status, and other factors, a localized response with influx of polymorphonuclear leukocytes, macrophages, and natural killer cells may occur. Polymorphonuclear cells release inflammatory mediators from granules, including reactive oxygen intermediates such as hydroxyl radicals, hydrogen peroxide, reactive nitrogen, and superoxide anion, and antimicrobial enzymes such as lysozyme, proteases, collagenases, and phospholipases. Macrophages may phagocytize antigens and express cytokines such as interleukin (IL)-1 that attract T lymphocytes to the site of inflammation. The complement system may be activated. If the pathogen is not contained, these localized events may amplify and lead to a larger systemic condition—the acute phase response—which is characterized by fever, somnolence, anorexia, and cachexia and is accompanied by metabolic alterations such as loss of muscle and negative nitrogen balance, fat catabolism, and impaired gluconeogenesis. The acute phase response also can be triggered by other factors, including trauma, burns, surgery, tissue infarction, exposure to chemicals or radiation, and advanced cancer.
During the acute phase response, changes occur in many plasma proteins, or acute phase proteins, which have been defined as proteins whose plasma concentrations
increase (positive acute phase proteins) or decrease (negative acute phase proteins) by at least one fourth during inflammatory disorders (10, 11). The liver is the main site of synthesis of most acute phase proteins. C-reactive protein (10), α-1- acid glycoprotein (12), and serum amyloid A (13) are among the best characterized positive acute phase proteins. Other positive acute phase proteins include ferritin, haptoglobin, and ceruloplasmin, and negative acute phase proteins include albumin and transthyretin.
increase (positive acute phase proteins) or decrease (negative acute phase proteins) by at least one fourth during inflammatory disorders (10, 11). The liver is the main site of synthesis of most acute phase proteins. C-reactive protein (10), α-1- acid glycoprotein (12), and serum amyloid A (13) are among the best characterized positive acute phase proteins. Other positive acute phase proteins include ferritin, haptoglobin, and ceruloplasmin, and negative acute phase proteins include albumin and transthyretin.
Cytokines play an important role in the acute phase response as inducers of acute phase proteins and hormones, as modulators of inflammation, and as activators and inhibitors of central nervous system functions related to appetite and metabolism (14). Cytokines that have been implicated in the anorexia that occurs during infection include IL-1β, IL-6, and tumor necrosis factor-α (TNF-α) (14). IL-1β and TNF-α are produced primarily by macrophages and can be triggered by stimuli such as bacterial wall products, lipopolysaccharide, viruses, parasites, and microbial superantigens. IL-6 is produced by monocytes, T lymphocytes, endothelial cells, and fibroblasts and is a major stimulator of the production of acute phase proteins in the liver. These cytokines may have a direct effect on hypothalamic neurons involved in appetite and other neural mediators such as serotonin, corticotropin-releasing factor, and β-melanocyte-stimulating hormone, and decreases in dopamine and neuropeptide Y. There may be increased cytokines in the circulation as well as the brain that modulate appetite during the acute phase response (15).
MALNUTRITION AND SPECIFIC INFECTIOUS DISEASES
The nutritional consequences of infection, no matter which microorganism is causing it, tend to be predictable. Any infection, whether symptomatic or asymptomatic, is accompanied by losses of some nutrients from the body and redistribution of other nutrients. The magnitude of these changes depends on the severity and duration of the infection. Metabolic and nutritional responses that are specific to certain organisms occur when the infection becomes localized within a single organ system. For example, diarrheal infections cause sizeable losses of fluid and electrolytes, whereas paralytic forms of infection result in wasting of bone and muscle. If the infection can be cured or eliminated naturally by the host immune system, lost body nutrients can then be replenished over a period of weeks to months. If the infectious process is not eliminated and becomes chronic, however, body composition can become markedly altered, and a new equilibrium of body nutrient balances is reached at a cachectic, or extremely wasted, level.
The impact of malnutrition on the severity of infection has been investigated most extensively in children with measles, diarrheal disease, respiratory infections, and malaria and in children and adults with tuberculosis (TB) and human immunodeficiency virus (HIV) infection.
Measles
Measles is a highly contagious disease caused by a virus. An estimated 278,358 cases of measles and 164,000 measles-related deaths were reported during 2008 (16, 17). The number of deaths in 2008 reflects a decrease of 78% since 2000 after the launch of the Measles Initiative, a vaccination campaign in high-risk countries jointly led by the American Red Cross, United Nations Foundation, US Centers for Disease Control and Prevention, United Nations Children’s Fund (UNICEF), and WHO. Most measles deaths continue to occur in the Southeast Asia region, as defined by the WHO (16). Deaths from measles are largely the result of an increased susceptibility to secondary bacterial and viral infections, and the underlying mechanism includes immune suppression related to malnutrition, especially vitamin A deficiency (18). Complications may occur in 10% to 30% of cases and include pneumonia, diarrhea, malnutrition, otitis media, mouth ulcers, corneal epithelial keratitis, corneal ulceration, and blindness.
Measles virus is an enveloped RNA virus in the genus Morbillivirus of the family paramyxoviridae. One known serotype and eight clades (A to H) of measles exists. Measles is spread when a susceptible person inhales aerosolized droplets containing measles virus. Viral replication occurs initially in macrophages in the lymphoid tissue of the nasopharynx, respiratory mucosa, and lungs (19). The measles virus enters human cells through a cell surface receptor known as signaling lymphocyte activation molecule (20). Viremia allows measles virus to spread to multiple organs, including the skin, liver, and conjunctiva, and a prodrome of fever, cough, and conjunctivitis occurs approximately 14 days after infection.
The immune response to measles is thought to be consistent with T-helper type 2-like immune responses in which antibody responses predominate and are driven by IL-4, IL-6, and IL-10. Antibody responses against measles virus proteins are detectable at the onset of the rash. Immune suppression often accompanies measles infection and increases the susceptibility to secondary infections. Delayed-type hypersensitivity skin test responses and in vitro proliferation of lymphocytes to viral antigens are often minimal or absent in measles infection (19). Infants are protected against measles virus infection by passively acquired maternal antibody to measles.
Although most people recover from measles, those with malnutrition or coinfections are at increased risk of complications (21, 22, 23, 24). In the classic early investigation of a measles outbreak in the Faroe Islands by Peter Panum and August Manicus in 1846, the most severe diarrheal disease and highest mortality were described
among those patients with greatest poverty and poor diet (25). Malnourished children have more severe disease and higher mortality (26, 27). More persistent measles infection and viral shedding also have (23) been reported in malnourished children (21). A close synergism exists between measles and vitamin A deficiency. One study in Zaire demonstrated that severely vitamin A-deficient children were three times more likely to die of measles than were children with better vitamin A status before infection (28). Children with measles who are vitamin A deficient also have a much higher risk of xerophthalmia, corneal ulceration, keratomalacia, and subsequent blindness (29).
among those patients with greatest poverty and poor diet (25). Malnourished children have more severe disease and higher mortality (26, 27). More persistent measles infection and viral shedding also have (23) been reported in malnourished children (21). A close synergism exists between measles and vitamin A deficiency. One study in Zaire demonstrated that severely vitamin A-deficient children were three times more likely to die of measles than were children with better vitamin A status before infection (28). Children with measles who are vitamin A deficient also have a much higher risk of xerophthalmia, corneal ulceration, keratomalacia, and subsequent blindness (29).
Vitamin A supplementation has shown to reduce the morbidity and mortality of measles among preschool children (30, 31, 32, 33, 34). Vitamin A supplementation appears to reduce the infectious complications associated with measles immune suppression, such as pneumonia and diarrheal disease, and these effects have been associated with modulation of immune responses by vitamin A (35, 36). In a randomized controlled trial in South Africa, children with severe measles who received vitamin A supplementation recovered more quickly from complications and were hospitalized for significantly fewer days. Their risk of death or a major complication was half that of their untreated counterparts (32). A metaanalysis of studies in the United Kingdom, South Africa, and Tanzania showed that vitamin A therapy for measles reduced mortality by 67% (37). Another metaanalysis of five randomized controlled clinical trials found that at least two doses of 200,000 IU of vitamin A on consecutive days were needed for significant reduction in measles mortality. Although one dose did not have a significant effect, two doses resulted in a 62% reduction in mortality (38). High-dose vitamin A therapy is now accepted as part of standard recommended treatment of measles infection in developing countries. Improving vitamin A status and immunization coverage are also cornerstones of current measles prevention efforts.
Malaria
Malaria is caused by a parasitic infection with protozoan organisms of the genus Plasmodium. In 2008, an estimated 247 million cases of malaria and approximately 1 million deaths from malaria occurred worldwide; more than 85% of malaria cases were in Africa (39). Of the four Plasmodium species that infect humans (Plasmodium falciparum, Plasmodium vivax, Plasmodium malariae, and Plasmodium ovale), the most serious morbidity and mortality are caused by P. falciparum (40). Malarial infection begins when a female Anopheles mosquito bites a human and releases sporozoites from the salivary gland into the circulating blood of the host. Sporozoites then enter hepatocytes, and in the preerythrocytic cycle, sporozoites grow and develop in the liver into thousands of merozoites. The hepatocytes then release merozoites, which invade erythrocytes. In the asexual erythrocytic cycle, merozoites develop into schizonts, and erythrocytes rupture, releasing more merozoites that can invade erythrocytes. In the sexual stage, some merozoites develop into gametocytes that are taken up in a blood meal by a mosquito, and within the mosquito, these gametocytes develop in microgametes and macrogametes.
In addition to the type of parasite, the extent of transmission and illness depends on the species of the Anopheles vector, human immunity, and climate. Specifically, vector species that have a long life span and species that prefer to bite humans over animals increase the chances of human transmission. Adults can develop some, but not complete, immunity to malaria after years of exposure, and transmission is highest in higher temperature and humid conditions (39). Transmission can be significantly reduced by insecticide-treated mosquito nets and indoor spraying with insecticides.
Malaria symptoms include fever, chills, and flu-like illness; and, if left untreated, malaria can progress to severe illness and death. Treatment generally includes antimalarial drugs, although regimens depend on the country and region where the infection occurs. Natural history studies suggest that the severity of malaria may be related to nutritional status. Some studies in the older literature have reported that malnutrition, and specifically protein-energy malnutrition, is protective against malaria. In particular, refeeding after famine appeared to increase the risk of malaria, especially among persons who are carriers of the disease (41, 42). Nevertheless, comprehensive reviews on protein-energy malnutrition showed that persons with better nutritional status have less severe malaria and a lower risk of death (40, 43, 44, 45).
Specific nutrients have been shown to affect malaria morbidity. Vitamin A, in particular, influences the incidence as well as the morbidity related to malaria (46, 47, 48). A randomized, placebo-controlled clinical trial was conducted in Papua New Guinea to examine the effects of vitamin A supplementation (60 mg retinol equivalents every 3 months) on malarial morbidity in preschool children (49). Children between 6 and 60 months of age were randomly allocated to receive vitamin A or placebo every 3 months. A weekly morbidity surveillance and clinic-based surveillance were established for monitoring acute malaria, and children were followed up for 1 year. Vitamin A significantly reduced the incidence of malaria attacks by about 20% to 50% for all except those with extremely high levels of parasitemia. Similarly, vitamin A supplementation reduced clinic-based malaria attacks, which consisted of self-solicited visits to the clinic by mothers who thought that their children should be seen because of fever. Vitamin A supplementation had little impact in children less than 12 months of age and the greatest effect among children 13 to 36 months old.
Studies of the benefits of zinc on malaria incidence and morbidity have shown conflicting results. Several studies
showed that zinc supplementation reduces the morbidity of malaria (50, 51, 52, 53, 54). In the Gambia, 110 children were randomized to receive 70 mg zinc or placebo twice weekly for 1.24 years. Zinc supplementation was associated with a 30% reduction in the incidence of clinical malaria episodes (50). In Papua New Guinea, 274 preschool children, 6 to 60 months old, received 10 mg zinc or placebo/day for 46 weeks. Zinc supplementation resulted in a 38% reduction in malaria morbidity (51). Other randomized controlled trials of zinc supplementation in children showed a 50% reduction in malaria attacks (P. vivax) and a reduction in malaria mortality (52, 53). In contrast, a clinical trial from Burkina Faso, in which children were supplemented with 12.5 mg zinc or placebo/day for 6 months, did not show an effect of zinc supplementation on clinical malaria episodes (55). Zinc supplementation also did not appear to reduce the morbidity of malaria (reduction of fever or parasitemia) when it was given as adjunct therapy with chloroquine to children, 6 months to 5 years old, who presented with an attack of acute uncomplicated falciparum malaria (56). Methodologic differences in assessment of malaria may explain the conflicting results, and more research is needed to determine the benefits of zinc supplementation on malaria.
showed that zinc supplementation reduces the morbidity of malaria (50, 51, 52, 53, 54). In the Gambia, 110 children were randomized to receive 70 mg zinc or placebo twice weekly for 1.24 years. Zinc supplementation was associated with a 30% reduction in the incidence of clinical malaria episodes (50). In Papua New Guinea, 274 preschool children, 6 to 60 months old, received 10 mg zinc or placebo/day for 46 weeks. Zinc supplementation resulted in a 38% reduction in malaria morbidity (51). Other randomized controlled trials of zinc supplementation in children showed a 50% reduction in malaria attacks (P. vivax) and a reduction in malaria mortality (52, 53). In contrast, a clinical trial from Burkina Faso, in which children were supplemented with 12.5 mg zinc or placebo/day for 6 months, did not show an effect of zinc supplementation on clinical malaria episodes (55). Zinc supplementation also did not appear to reduce the morbidity of malaria (reduction of fever or parasitemia) when it was given as adjunct therapy with chloroquine to children, 6 months to 5 years old, who presented with an attack of acute uncomplicated falciparum malaria (56). Methodologic differences in assessment of malaria may explain the conflicting results, and more research is needed to determine the benefits of zinc supplementation on malaria.
Iron deficiency anemia is widespread among malaria endemic regions, and iron supplementation is often a key component of relief efforts. A metaanalysis of iron supplementation in malaria endemic regions, however, showed a tendency toward higher parasite counts in individuals who received iron supplements (57). In contrast, a Cochrane Review in 2009 reported no increased risk of malaria with iron supplementation in areas with regular malaria surveillance and where malaria treatment services were provided (58). Therefore, the investigators concluded that iron supplementation should not be discontinued in areas where malaria is endemic.
Diarrheal Disease
Diarrheal disease, the second leading cause of death in children younger than 5 years of age, causes an estimated 1.5 million deaths per year (59). The main causes of diarrheal diseases among children in developing countries are rotavirus, Escherichia coli, Shigella, Vibrio cholerae, Salmonella, and Entamoeba histolytica. The epidemiology, clinical features, immunology, and pathogenesis of diarrhea differ according to the characteristics of the pathogen in terms of production of toxins, tissue invasion, fluid and electrolyte loss, and location of infection.
Diarrhea is generally defined as the condition in which three or more liquid stools are passed in a 24-hour period (60). In developing countries, community-based studies suggest that children less than 5 years of age have a median incidence of three diarrheal episodes per year (59, 60). In general, host defenses in the gut include gastric acidity, the presence of normal microflora, gut motility, mucus production, integrity of microvilli, local secretion of antibody, and cell-mediated immunity; micronutrient malnutrition may impair some of these host defenses. Malnourished infants and children are at higher risk for diarrhea, which, in turn, can exacerbate malnutrition and can result in more severe disease and higher mortality from diarrhea (59, 61, 62).
Diarrhea can lead to dehydration, electrolyte imbalance, and malabsorption of both macronutrients and micronutrients. Vitamins A, D, B12, copper, folate, iron, magnesium, zinc, and selenium deficiencies have all been described during diarrheal disease (60). Frequent episodes of diarrheal disease can also cause significant damage to the lining of the gut and disrupt normal gut flora colonization, changes that further exacerbate poor nutrition status and immune function.
Exclusive breast-feeding for the first 4 to 6 months of life decreases both the severity and the incidence of diarrhea among infants (63). In particular, breast-feeding reduces sanitation concerns with other forms of infant feeding and eliminates the need for clean sources of drinking water.
Supplementation of specific micronutrients may play an important therapeutic role in managing diarrheal disease. In a community-based study in India, children who received micronutrient supplementation with zinc had a 23% faster recovery following a diarrheal episode and a 39% reduction of likelihood that the episode lasted longer than 7 days, compared with children who received micronutrient supplementation without zinc (64). Zinc also was associated with a reduction in the severity of diarrheal disease. In an analysis of four clinical trials on children with persistent diarrhea, zinc was associated with a 29% faster recovery and 49% lower rate of treatment failure or death (65). In 2004, the WHO and UNICEF jointly recommended that zinc be used as a therapy for all children with diarrheal disease (66).
Vitamin A deficiency is associated with an increased incidence of diarrheal disease, and diarrheal disease is associated with an increased incidence of vitamin A deficiency in children (67, 68, 69, 70, 71, 72). Although earlier studies on vitamin A supplementation or fortification showed a reduction in morbidity and mortality of diarrheal diseases among preschool children, more recent trials had conflicting results (60, 73). The benefit of vitamin A appears to be limited to children who are vitamin A deficient or malnourished or to children with shigellosis, and the current WHO recommendation does not include vitamin A as part of treatment of diarrhea.
Some evidence is emerging on the benefits of probiotics, lectins, short-chain fatty acids, and fiber, although further studies are needed to confirm their role in the treatment of diarrhea (60).
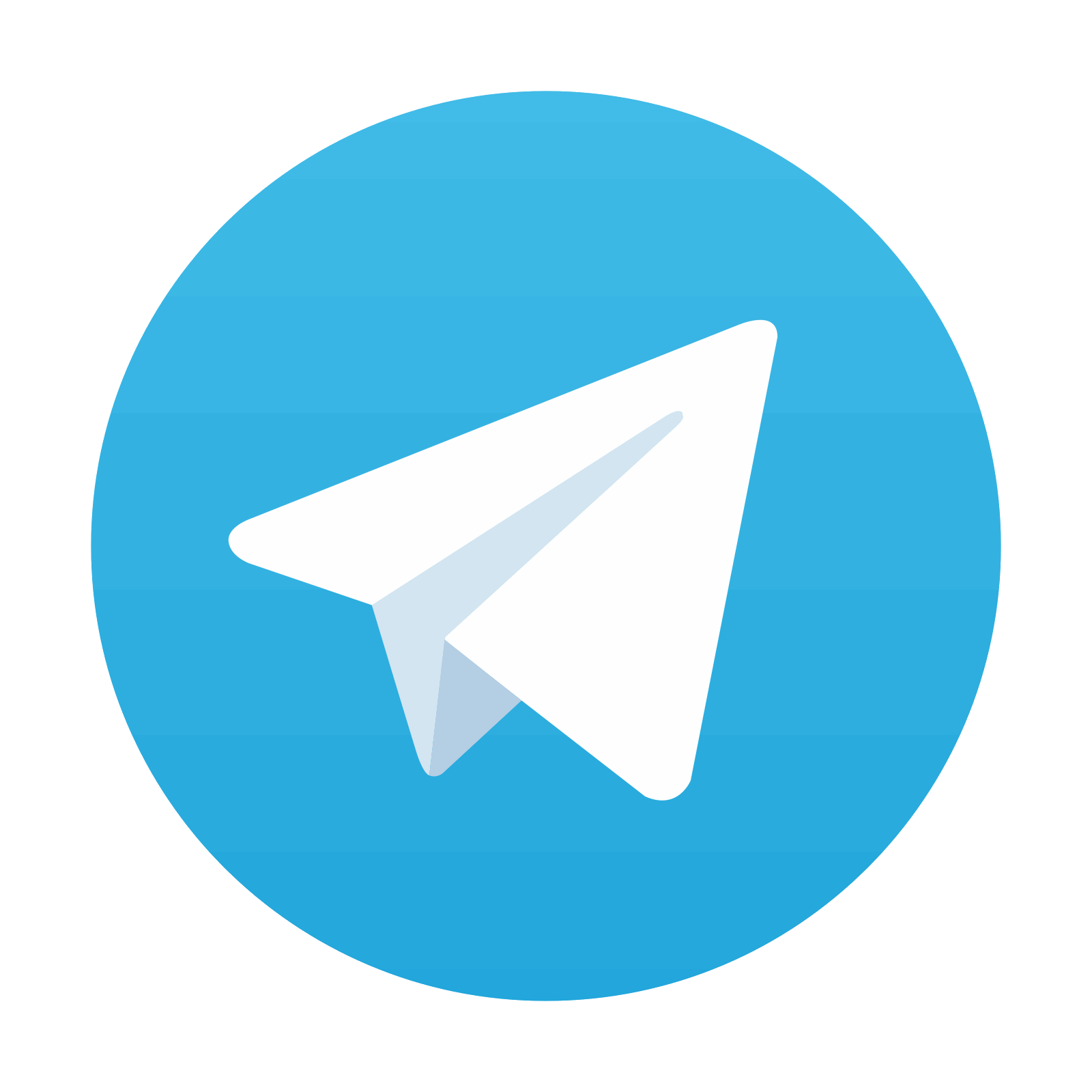
Stay updated, free articles. Join our Telegram channel

Full access? Get Clinical Tree
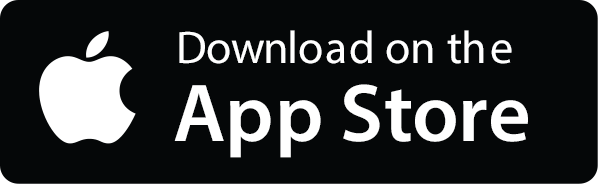
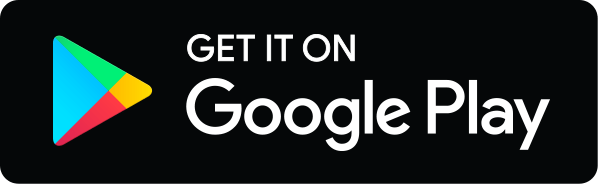