- Nitroimidazoles are prodrugs which are reduced in vivo to give radical species that are toxic to cells. Resistance arises due to decreased reduction by the cellular enzymes.
- Owing to this mechanism of action, the nitroimidazoles show activity against anaerobic bacteria and are therefore used to treat anaerobic infections.
- The nitroimidazoles are generally well tolerated, but on rare occasions can cause severe side effects such as pancreatitis.
- Alcohol should be avoided when taking nitroimidazoles.
Antibacterial nitroimidazoles used clinically are metronidazole and tinidazole; their structures are shown in Figure 2.3.1 and their therapeutic indications are listed in Table 2.3.1.
Table 2.3.1 Therapeutic indications for the nitroimidazole antibacterials.
Nitroimidazole antibacterial | Indications |
Metronidazole | Anaerobic infections, Trichomonas vaginalis infection, Helicobacter pylori eradication (part of triple therapy), rosacea, bacterial vaginosis, pelvic inflammatory disease, acute ulcerative gingivitis, acute oral infections, acute invasive amoebic dysentery, giardiasis |
Tinidazole | Anaerobic infections, bacterial vaginosis, acute ulcerative gingivitis, abdominal surgery prophylaxis, acute invasive amoebic dysentery, giardiasis |
2.3.1 Discovery
Nitroimidazole antibacterial activity was first discovered in extracts from Nocardia mesenterica (Maeda et al., 1953) and Streptomyces eurocidicus (Osato et al., 1955). The active agent, called azomycin, was identified as 2-nitroimidazole (Figure 2.3.2) (Nakamura et al., 1955), a metabolite produced from arginine (at least in the latter of these two bacteria) (Nakane et al., 1977). After many unsuccessful attempts to synthesise this simple molecule, researchers at Rhône-Poulenc turned their attention to the production and evaluation of the synthetically less challenging 5-nitroimidazole isomers, leading to the identification of metronidazole as a potent antiprotozoal agent with greater activity than azomycin and an acceptable toxicity profile (Cosar and Julou, 1959). The synthesis of 2-nitroimidazole was not achieved until 10 years later, and then only in low yield (Lancini and Lazzari, 1965). For more on the discovery of the nitroimidazoles, see Anon (1978).
2.3.2 Synthesis
The synthetic route to metronidazole patented by Rhône-Poulenc (Scheme 2.3.1) (Jacob et al., 1960) starts with 2-methyl-5-nitroimidazole, which can be accessed from 2-methylimidazole, itself prepared by reacting ethylene diamine with acetic acid to obtain the salt, then heating at high temperature until dry, resulting in the diamide 1. Deprotonation by calcium oxide initiates cyclisation to product 2, which can then be oxidised to the aromatic imidazole using Raney-nickel. This classical chemistry is successful in producing the product, but poor yields are obtained for most steps. Despite this limitation, hundreds of derivatives have been synthesised and tested (Cosar and Julou, 1959; Cosar et al., 1966) and there are many patents on the synthesis and activities of nitroimidazoles (mostly from the 1960s), the majority of which focus on the particularly low-yielding final step. Besides ethylene oxide, as depicted in Scheme 2.3.1, chloroethanol or ethylene carbonate were also commonly used. Later optimisation of the synthetic route improved the yields (Kraft et al., 1989) such that metronidazole analogues are still being prepared by the same methodology (e.g. Atia, 2009) or else use metronidazole as the starting material (e.g. Mao et al., 2009; Kumar et al., 2010).
2.3.3 Bioavailability
The 5-nitroimidazoles are generally well-absorbed, with an oral bioavailability for metronidazole and tinidazole approaching 100% (Lau et al., 1992; Freeman et al., 1997; Fung and Doan, 2005). Following oral administration, peak plasma concentrations occur after 1–2 hours, with 500 mg of metronidazole typically delivering Cmax values of 8–13 mg/L after about 15 minutes to 4 hours. Tinidazole shows similar, if slightly more favourable, pharmacokinetic parameters and the absorption of neither drug is significantly affected by food. As the MIC of these concentration-dependent antibacterial agents is generally between 0.25 and 4 mg/L for susceptible species, appropriate therapeutic concentrations are readily achieved. Both metronidazole and tinidazole distribute well throughout a variety of tissues, including the CNS, with reported volumes of distribution of 0.53–0.96 L/kg for metronidazole and approximately 0.65 L/kg for tinidazole, thus providing excellent therapeutic potential for the treatment of anaerobic infections in most organs and tissues.
The plasma half-life of metronidazole is between 6 and 8 hours, while that of tinidazole is rather longer (12–14 hours), resulting in a requirement for 2–3 times daily dosing for metronidazole and 1–2 times daily for tinidazole. Both metronidazole and tinidazole are primarily metabolised in the liver by CYP 3A4; the metabolites are mostly excreted in the urine, while a small proportion (about 10–15%) of unchanged drug is excreted in the bile and in the urine, and a further 10% is excreted in the faeces.
2.3.3.1 Bacterial Uptake of Metronidazole
This small molecule can enter many bacteria by passive diffusion; however, the uptake of metronidazole into bacterial cells has also been linked to the presence of ferredoxin-linked electron transport or hydrogenase systems, which correlates with the antibacterial activity. As we will discuss in the next subsection, the mode of action of metronidazole involves its bioreduction by a ferredoxin-based enzyme or hydrogenase, which facilitates its transport into susceptible organisms.
2.3.4 Mode of Action and Selectivity
As far as possible, the following account of the mode of action and selectivity of the 5-nitroimidazoles relates to all products in the class, but metronidazole is the most commonly prescribed nitroimidazole and has been the most extensively studied for its mode of action, so it is often the focus of this summary.
During studies on the scope of activity and the mode of action, it was quickly evident that the nitro group is responsible for the bactericidal action of 5-nitroimidazoles, while the other substituents on the imidazole ring confer slightly different pharmacokinetic properties on each nitroimidazole, providing a choice of agents for different infections (Goldman, 1982).
Despite the clinical use of metronidazole over many years, the exact details of its mode of action remain unclear, but it is known to involve four steps: (1) the nitroimidazole must pass into the cell, before (2) the nitro group is bioreduced to a reactive radical species, which (3) can react with a cellular species, such as DNA, and lastly (4) inactivated products are released. A similar mode of action is believed to exist for the other 5-nitroimidazoles (Tocher, 1997).
Studies of susceptible bacteria and protozoa led to a link between the bactericidal activity and the presence of ferredoxin-linked electron transport or hydrogenase systems. Although not a perfect correlation, the presence and activity of ferredoxin (Edwards et al., 1973) or a ferredoxin-linked hydrogenase (Church et al., 1996) can generally be matched to susceptibility to the 5-nitroimidazoles. Furthermore, the cellular uptake of metronidazole is linked to the rate of reduction – as it is reduced intracellularly, a favourable concentration gradient results in more metronidazole being taken into the cell to be reduced, contributing to its efficiency. This was first noted in the protozoan species Trichomonas vaginalis (Ings et al., 1974), but appears to be general across metronidazole-susceptible species, including bacteria (Edwards, 1980).
Once in the cell, the reduction of a nitro compound can progress through a number of stages to the ultimate production of the corresponding amino compound; the first one-electron reduction results in the reactive nitroimidazole radical anion, the reactive radical species referred to in Step (2) above, which is a key intermediate and has a number of possible fates (Scheme 2.3.2). It can:
- React with a cellular species, such as DNA or protein, and cause irreparable damage, leading to bactericidal activity: pathway (a).
- Accept a further electron to form the nitrosoimidazole product: pathway (b).
- React with another nitroimidazole radical anion in a disproportionation reaction to form a neutral nitrosoimidazole product and a molecule of nitroimidazole: pathway (c).
- Decompose to form an imidazole radical and nitrite anion: pathway (d).
- React with molecular oxygen, forming a superoxide radical anion and the starting nitroimidazole: pathway (e) (Goldman, 1982; Edwards, 1993; Tocher 1997).
There is some evidence that the nitroimidazole radical anion becomes protonated and in this form is more cytotoxic (Tocher, 1997).
Scheme 2.3.2 Single electron reduction of nitroimidazoles and possible fates of the key intermediate, nitroimidazole radical anion
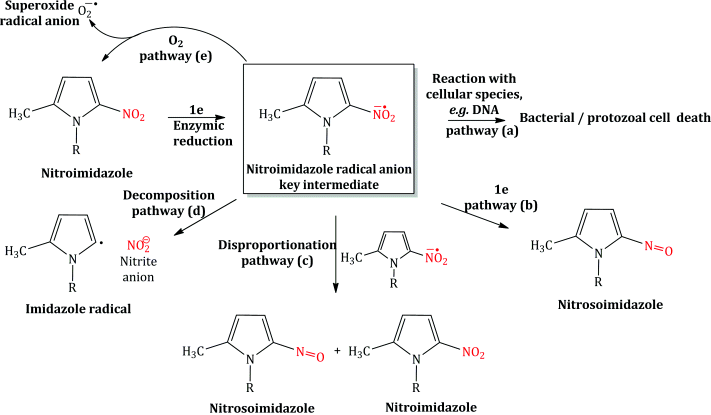
The nitroimidazoles, then, are prodrugs that are bioactivated in vivo to the reactive nitroimidazole radical anion. In the presence of oxygen, such as that found in obligate aerobes, the cytotoxic nitroimidazole radical anion is rapidly inactivated, eliminating the bactericidal effect; only when reduced under anaerobic conditions, such as by obligate anaerobic bacteria, where the potential to trap and neutralise the reactive species is limited, does irreparable cellular damage result, mostly observed as DNA degradation (Knight et al., 1978; Tocher and Edwards, 1994), leading to cell death.
Further two-electron reduction of the nitrosoimidazole yields the hydroxylamine, and a final two-electron reduction results in the amine (Scheme 2.3.3), but studies with these products suggest that they are non-cytotoxic and have no apparent role in the bactericidal effect (Tocher and Edwards, 1994).
Scheme 2.3.3 Further reduction of nitrosoimidazole to the aminoimidazole through the hydroxylaminoimidazole intermediate

2.3.4.1 Selective Toxicity
Tests on the reduction of metronidazole in cultured mammalian cells showed significant cytotoxicity, yet there are few side effects to metronidazole treatment and it is commonly used during pregnancy. It seems likely that metronidazole is not reduced by mammalian enzymes and that its selectivity arises due to its reduction solely by intracellular bacterial and protozoal enzymes (probably due to favourable redox potentials in these species) (Edwards, 1993; Ehlhardt et al., 1988), followed by rapid reaction of the reactive products with the bacterial or protozoal DNA, before the products are able to escape the cell into the mammalian host.
2.3.5 Mechanisms of Resistance
As the mode of action involves reduction of the nitroimidazole to a bioactive form, it is probably not surprising to find that resistance arises due to a decrease in expression and/or activity of the reductive bacterial (or protozoal) enzyme, known to be ferredoxin in clostridia species (Edwards, 1980, 1993). Despite this obvious ‘Achilles’ heel’ point of fragility, resistance to the 5-nitroimidazoles is rare, even though they have been used extensively worldwide over a 40-year period. Ferredoxin is part of the essential glucose metabolism pathway and the few bacteria which have developed resistance to the nitroimidazoles appear to have compensated for reduced ferredoxin/oxidoreductase activity through the upregulation of alternative enzymes for glucose metabolism (such as lactate dehydrogenase in a resistant strain of Bacteroides fragilis) (Edwards, 1980, 1993), and these resistant strains do not reduce and activate the nitroimidazole.
As we will see in the next subsection, the recent rise in resistance to metronidazole of Helicobacter pylori, again through the inactivation of the oxidoreductase enzyme(s) reponsible for the reduction and activation of the nitroimidazole, is a great concern (van der Wouden et al., 2000; Jenks and Edwards, 2002).
The resistance of anaerobic protozoa has been studied more fully and there are several reviews of the topic (e.g. Land and Johnson, 1997, 1999; Upcroft and Upcroft, 2001; Dunne et al., 2003), but this is beyond the scope of this book, which seeks to concentrate upon bacteria.
2.3.6 Clinical Applications
2.3.6.1 Spectrum of Activity
Metronidazole and tinidazole have activity against a wide range of pathogenic microorganisms, encompassing various protozoa (such as Giardia lamblia and T. vaginalis) and Gram negative (such as B. fragilis and Fusobacterium necrophorum) and Gram positive anaerobic bacteria (such as Clostridium difficile and Peptostreptococcus anaerobius). Another relatively recent development is that these agents also have activity against H. pylori, a Gram negative, microaerophillic bacterium found in the stomachs of humans, which is thought to cause peptic ulcers.
These drugs were originally introduced to treat protozoal infections, but were then subsequently found to have activity against certain pathogenic anaerobic bacteria. The combined protozoal and anaerobic activity of these agents allows them to be used in the treatment of a broad range of conditions.
As infections due to protozoa are outwith the scope of this book, we will mention these here only in brief.
2.3.6.2 Trichomoniasis
The introduction of metronidazole to the market in 1957 significantly improved the management of trichomoniasis infections. Single-dose therapy with metronidazole (2 g) is effective at treating trichomoniasis (Woodcock, 1972) (also sometimes known as ‘trich’), a sexually transmitted infection (STI) affecting men and women caused by the flagellated, singled-celled, protozoan T. vaginalis. Trichomoniasis infection is a significant public health concern with around 170 million people estimated to be infected worldwide (World Health Organization, 2001). Indeed, it has been reported that the incidence of trichomoniasis infection is higher than that of other common non-viral STIs, such as chlamydia and gonorrhoea (Weinstock et al., 2004). In women, the clinical manifestations of T. vaginalis infection can include green/yellow vaginal discharge, vulvar erthema, and dyspareunia (painful sexual intercourse), while in men they can include urethral discharge and occasionally urethritis. Symptoms of infection are more common in women than in men, but as many as 50% of women will not show any symptoms.
Single-dose therapy offers the advantage of improving patient compliance, but may be associated with higher side effects, such as nausea, when compared to lower-dose weekly regimens. Patients who cannot tolerate the adverse effects associated with the single-dose treatment may be offered a longer treatment regimen; for example, 200 mg every 8 hours for 7 days, or 400–500 mg every 12 hours for 5–7 days. Alternatively, if metronidazole is ineffective or not tolerated, tinidazole can be given as a one-off single 2 g dose to treat the infection. Metronidazole can also be given intravaginally, but this is not recommended, as parasitological cure rates are low compared to oral treatment (around 90% cure rate for oral treatment compared to around 50% cure rate for intravaginal treatment) (Forna and Gülmezoglu, 2003).
Unfortunately, clinical isolates resistant to metronidazole have been reported and their number appears to be increasing (Cudmore et al., 2004). Refractory cases are often treated using an increased dose of metronidazole, or by switching to tinidazole, but because of the similar mode of action of these agents, cross-resistance is a real clinical concern.
A Cochrane review confirms that the nitroimidazoles, metronidazole and tinidazole, are effective at treating trichomoniasis, but the authors were unable to recommend the use of one drug over another due to lack of good-quality evidence comparing the two agents (Forna and Gülmezoglu, 2003).
2.3.6.3 Giardiasis
Symptoms of giardiasis infection include diarrhoea, abdominal cramps, nausea, and steatorrhea (‘floaty poos’), and one risk of chronic infection, particularly in children, is malabsorption, which could ultimately lead to malnutrition and poor physical development.
Several years after the introduction of metronidazole for the treatment of T. vaginalis infections, in 1962, Darbon and coworkers reported that metronidazole could also be used to manage Giardia lamblia infections (Darbon et al., 1962). Since then, other agents, such as the anthelmintics, albendazole and mebendazole, have also been shown to be effective, and these have a different mode of action (and spectrum of activity) to the nitroimidazoles.
A review of the drugs used to treat giardiasis showed that metronidazole was the most frequently tested drug (i.e. it had the largest number of published clinical trials in the literature). In addition, the data in this review showed that, in randomised controlled trials, metronidazole had a mean cure rate of 81.5%, while tinidazole, albendazole, and mebendazole had mean cure rates of 91.1, 73.4, and 65.6%, respectively (Busatti et al., 2009). It appears that metronidazole is more effective at treating giardiasis when given at high doses over a prolonged period of time, while tinidazole remains effective when given as a one-off single dose, and these findings are reflected in the recommended dosage regimens for metronidazole and tinidazole: metronidazole should be given as 2 g daily for 3 days (or alternatively, 400 mg three times daily for 5 days, or 500 mg twice daily for 7–10 days), while tinidazole can be given as a 2 g single dose. Currently, metronidazole is the drug of choice in the treatment of giardiasis, and tinidazole should be used as an alternative.
2.3.6.4 Amoebiasis
Amoebiasis is a disease caused by the protozoan parasite Entamoeba histolytica and is responsible for a high number of mortalities annually worldwide (in excess of 50 000 deaths per year). Indeed, in terms of mortality caused by protozoan parasites, amoebiasis is second only to malaria, which gives an indication of the severity of this infection. Perhaps the most famous (and significant) outbreak of amoebiasis was during 1933, in Chicago, when a sewer pipe contaminated the water supply to a local hotel, causing more than 700 cases of amoebic dysentery to be reported (Bundesen et al., 1934).
Metronidazole is considered the drug of choice for invasive intestinal amoebiasis and extraintestinal amoebiasis (including liver abcesses). Tinidazole is a useful alternative to metronidazole; it is more effective at reducing clinical failure when compared to metronidazole and is also associated with fewer side effects (Gonzales et al., 2009).
In patients with invasive disease, metronidazole should first be prescribed (usually for 5 or 10 days) to eradicate trophozoites in the tissues (such as the liver). This should be followed by the administration of a luminal amoebicide (usually for 10 days) to eliminate any surviving organisms in the colon.
2.3.6.5 Helicobacter Pylori
Metronidazole is also used as part of combination therapy to eradicate H. pylori – a Gram negative, microaerophillic bacterium, which is a leading cause of gastritis, peptic ulcer disease, and gastric cancer worldwide (around 95% of duodenal ulcers and 80% of gastric ulcers are thought to be caused by H. pylori). Indeed, because of the increased risk of developing gastric cancer associated with H. pylori infection, the WHO’s International Agency for research on cancer has classified it as a type I carcinogen (i.e. carcinogenic to humans).
H. pylori infection is now universally accepted as being a leading cause of gastroduodenal disease. This, however, was not always the case – prior to 1982 it was thought that stomach and duodenal ulcers were caused by smoking, stress, and lifestyle factors. It was not until 1982 that two Australian researchers, Robin Warren and Barry Marshall, made the connection between H. pylori infection and peptic ulcer disease (Marshall and Warren, 1984). Despite this evidence, several attempts to culture the bacterium were unsuccessful and it wasn’t until the culture plate was unintentionally left in the incubator for 5 days over the Easter holidays (we’ve all seen kitchens where something similar happens) that a culture of H. pylori was successfully obtained (Marshall et al., 1984). Prior to this, the cultures were discarded after 48 hours if no growth had occurred. Once H. pylori had been isolated, the medical community still remained sceptical that a bacterium could cause gastroduodenal disease. Marshall then showed that ingestion of H. pylori could cause acute symptoms of gastritis, by drinking a suspension containing a 4-day-old culture of H. pylori, a dramatic (and brave) confirmation that H. pylori was, indeed, a pathogen.
By the mid-nineties, the medical communities had accepted Warren and Marshall’s pioneering research, and in 2005 they were awarded the Nobel Prize in Physiology or Medicine for their discovery of ‘the bacterium Helicobacter pylori and its role in gastritis and peptic ulcer disease’.
It soon became apparent that antibacterial drugs could be used to eradicate H. pylori, which proved to be an efficient and effective way of treating gastritis and peptic ulcer disease3 (Marshall et al., 1988). The use of triple therapy (two antibacterial agents plus an acid suppressant, such as ranitidine) proved to be particularly effective at eradicating H. pylori (Borody et al., 1989). One study showed that ranitidine, metronidazole, and amoxicillin eradicated H. pylori in 89% of patients compared to 2% of patients taking ranitidine alone. Furthermore, the same study also showed that ulcers reoccurred in 2% of patients in whom H. pylori had been eradicated, compared to 85% in whom H. pylori had persisted (Hentschel et al., 1993).
Nowadays, metronidazole forms a key component in many regimens used to eradicate H. pylori (Table 2.3.2). Tinidazole can also be used for eradication therapy, as an alternative to metronidazole, although this is not commonplace. Unfortunately, it will come as no surprise to learn that, as with most bacterial infections, the development of resistance has had a significant impact on successful treatment. Resistance to metronidazole is common and can vary widely between geographical locations (Wang et al., 2000; Kim et al., 2001), with resistance rates as high as 80% reported in central Africa (Glupczynski et al., 1990).
Table 2.3.2 Recommended regimens used to eradicate H. pylori in adults. Regimens are generally taken for seven consecutive days (adapted from BNF).
Regimen | Dose |
Metronidazole | 400 mg twice daily |
Clarithromycin | 250 mg twice daily |
Lansoprazole | 30 mg twice daily |
Clarithromycin | 500 mg twice daily |
Amoxicillin | 1 g twice daily |
Lansoprazole | 30 mg twice daily |
Metronidazole | 400 mg three times daily |
Amoxicillin | 500 mg three times daily |
Omeprazole | 20 mg twice daily |
Amoxicillin | 1 g twice daily |
Clarithromycin | 500 mg twice daily |
Omeprazole | 20 mg twice daily |
2.3.6.6 Anaerobic Infections
Metronidazole has activity against a range of pathogenic anaerobic bacteria and is consequently considered the agent of choice to treat anaerobic infections (Löfmark et al., 2010). Indeed, metronidazole has been successfully used to treat a variety of serious anaerobic infections, with examples including septicaemia caused by F. necrophorum, osteomyelitis caused by B. fragilis, and necrotising pneumonia caused by Fusobacterium nucleatum (Tally et al., 1975; Boutoille et al., 2003). It is also used to treat other infections from which pathogenic anaerobic bacteria have been isolated, such as brain abscess, bacteraemia, pelvic abscess, puerperal sepsis, and post-operative wound infections.
Metronidazole also has a role to play in the treatment of bacterial vaginosis, a condition commonly caused by overgrowth of Gardnerella vaginalis. Some women with bacterial vaginosis can remain asymptomatic, while others can show symptoms – commonly an abnormal vaginal discharge with an unpleasant ‘fish-like’ odour. In view of this, it was previously considered a minor infection with few complications, but there is now evidence to suggest that, in pregnancy, bacterial vaginosis can lead to a higher risk of preterm birth (Hillier et al., 1995). Metronidazole is considered the agent of choice for the treatment of bacterial vaginosis; it may be given as a tablet, or if the patient prefers, an intravaginal gel – both formulations appear to be equally effective, with similar cure rates observed (Ferris et al., 1995; Hanson et al., 2000).
Another indication of metronidazole is for the treatment of C. difficile infection (CDI) (also known as ‘C. diff’) – a Gram positive anaerobe that has, over the last few years, received a great deal of media attention. Around 5% of healthy adults carry C. difficile in their colon without showing any signs of infection. However, if a patient is taking a broad-spectrum antibiotic4 for, say, a UTI, this can disrupt the normal intestinal flora, which may facilitate the colonisation of C. difficile and ultimately lead to CDI. C. difficile produces two principal toxins – A and B – which cause diarrhoea, inflammation, and ultimately, C. difficile-associated diarrhoea (CDAD), which can be fatal (Starr, 2005). CDAD is diagnosed by the presence of diarrhoea and the positive detection of C. difficile toxins in the faeces. As diagnosis tends to focus on the presence of C. difficile toxins, laboratories tend not to culture it, although culturing techniques are available (Figure 2.3.3). This, however, has its disadvantages, as toxins are absent in a small number of confirmed cases, meaning that only testing for the presence of C. difficile toxin may not necessarily diagnose all cases of CDAD (Delmée et al., 2005).
Figure 2.3.3 C. difficile colonies grown on blood agar (top) and cycloserine mannitol agar (bottom); both after 48 hours (Image courtesy of Public Health Image Library, Image ID3647 and ID3649, Online, [http://phil.cdc.gov/phil/home.asp, last accessed 29th March 2012].)
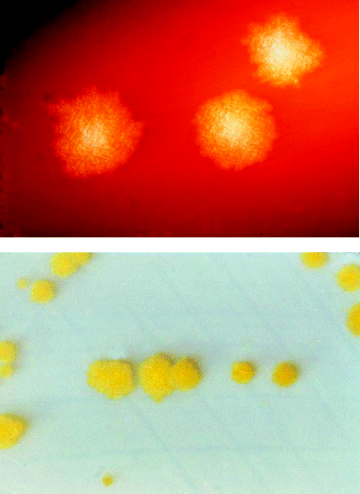
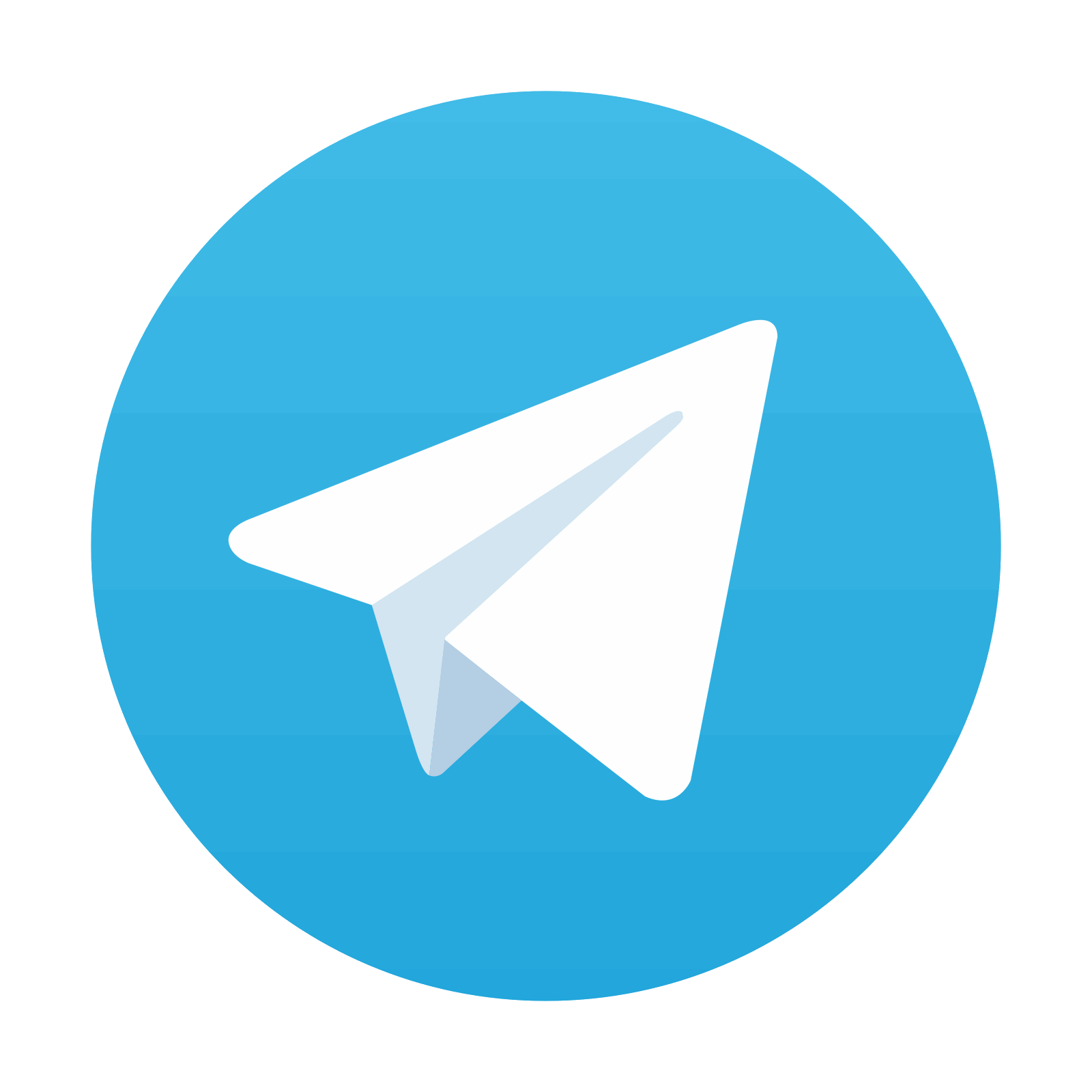
Stay updated, free articles. Join our Telegram channel

Full access? Get Clinical Tree
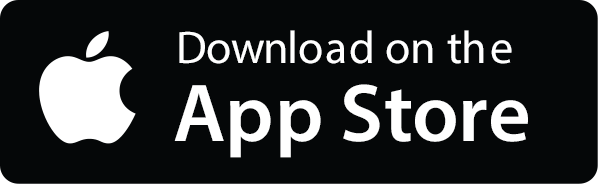
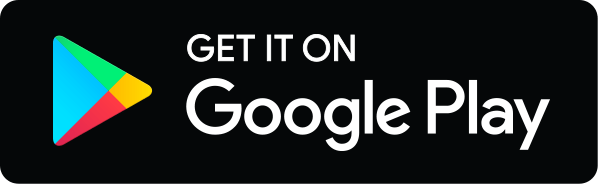