Neurotransmission: The Autonomic and Somatic Motor Nervous Systems
ANATOMY AND GENERAL FUNCTIONS
The autonomic nervous system (ANS; a.k.a. the visceral, vegetative, or involuntary nervous system) regulates autonomic functions that occur without conscious control. In the periphery, it consists of nerves, ganglia, and plexuses that innervate the heart, blood vessels, glands, other visceral organs, and smooth muscle in various tissues.
DIFFERENCES BETWEEN AUTONOMIC AND SOMATIC NERVES
• The efferent nerves of the ANS supply all innervated structures of the body except skeletal muscle, which is served by somatic nerves.
• The most distal synaptic junctions in the autonomic reflex arc occur in ganglia that are entirely outside the cerebrospinal axis. Somatic nerves contain no peripheral ganglia, and the synapses are located entirely within the cerebrospinal axis.
• Many autonomic nerves form extensive peripheral plexuses; such networks are absent from the somatic system.
• Postganglionic autonomic nerves generally are nonmyelinated; motor nerves to skeletal muscles are myelinated.
• When the spinal efferent nerves are interrupted, smooth muscles and glands generally retain some level of spontaneous activity, whereas the denervated skeletal muscles are paralyzed.
VISCERAL AFFERENT FIBERS. The afferent fibers from visceral structures are the first link in the reflex arcs of the autonomic system. With certain exceptions, such as local axon reflexes, most visceral reflexes are mediated through the central nervous system (CNS).
Information on the status of the visceral organs is transmitted to the CNS through 2 main sensory systems: the cranial nerve (parasympathetic) visceral sensory system and the spinal (sympathetic) visceral afferent system. The cranial visceral sensory system carries mainly mechanoreceptor and chemosensory information, whereas the afferents of the spinal visceral system principally convey sensations related to temperature and tissue injury of mechanical, chemical, or thermal origin.
Cranial visceral sensory information enters the CNS by 4 cranial nerves: the trigeminal (V), facial (VII), glossopharyngeal (IX), and vagus (X) nerves. These 4 cranial nerves transmit visceral sensory information from the internal face and head (V); tongue (taste, VII); hard palate and upper part of the oropharynx (IX); and carotid body, lower part of the oropharynx, larynx, trachea, esophagus, and thoracic and abdominal organs (X), with the exception of the pelvic viscera. The pelvic viscera are innervated by nerves from the second through fourth sacral spinal segments. The visceral afferents from these 4 cranial nerves terminate topographically in the solitary tract nucleus.
Sensory afferents from visceral organs also enter the CNS from the spinal nerves and convey information concerned with temperature as well as nociceptive visceral inputs related to mechanical, chemical, and thermal stimulation. Those concerned with muscle chemosensation may arise at all spinal levels, whereas sympathetic visceral sensory afferents generally arise at the thoracic levels where sympathetic preganglionic neurons are found. The neurotransmitters that mediate transmission from sensory fibers have not been characterized unequivocally. Substance P and calcitonin gene-related peptide (CGRP), are leading candidates for neurotransmitters that communicate nociceptive stimuli from the periphery. Somatostatin (SST), vasoactive intestinal polypeptide (VIP), and cholecystokinin (CCK), also occur in sensory neurons. ATP appears to be a neurotransmitter in certain sensory neurons. Enkephalins, present in interneurons in the dorsal spinal cord, have antinociceptive effects both pre- and postsynaptically to inhibit the release of substance P. The excitatory amino acids glutamate and aspartate also play major roles in transmission of sensory responses to the spinal cord. These transmitters and their signaling pathways are reviewed in Chapter 14.
DIVISIONS OF THE PERIPHERAL AUTONOMIC SYSTEM. The ANS consists of 2 large divisions: the sympathetic and the parasympathetic (Figure 8–1).
Figure 8–1 The autonomic nervous system. Yellow, cholinergic; red, adrenergic; dotted blue, visceral afferent; solid lines, preganglionic; broken lines, postganglionic. The rectangle at right shows the finer details of the ramifications of adrenergic fibers at any 1 segment of the spinal cord, the path of the visceral afferent nerves, the cholinergic nature of somatic motor nerves to skeletal muscle, and the presumed cholinergic nature of the vasodilator fibers in the dorsal roots of the spinal nerves. The asterisk (*) indicates that it is not known whether these vasodilator fibers are motor or sensory or where their cell bodies are situated.
The neurotransmitter of all preganglionic autonomic fibers, most postganglionic parasympathetic fibers, and a few postganglionic sympathetic fibers is acetylcholine (ACh). Some postganglionic parasympathetic nerves use nitric oxide (NO) and are referred to as nitrergic. The majority of the postganglionic sympathetic fibers are adrenergic, in which the transmitter is norepinephrine (NE, noradrenaline). The terms cholinergic and adrenergic describe neurons that liberate ACh or NE, respectively. Substance P and glutamate may also mediate many afferent impulses.
SYMPATHETIC NERVOUS SYSTEM. The cells that give rise to the preganglionic fibers of this division lie mainly in the intermediolateral columns of the spinal cord and extend from the first thoracic to the second or third lumbar segment. The axons from these cells are carried in the anterior (ventral) nerve roots and synapse, with neurons lying in sympathetic ganglia outside the cerebrospinal axis. Sympathetic ganglia are found in 3 locations: paravertebral, prevertebral, and terminal.
The 22 pairs of paravertebral sympathetic ganglia form the lateral chains on either side of the vertebral column. The ganglia are connected to each other by nerve trunks and to the spinal nerves by rami communicantes. The white rami carry the preganglionic myelinated fibers that exit the spinal cord by the anterior spinal roots. The gray rami carry postganglionic fibers back to the spinal nerves for distribution to sweat glands and pilomotor muscles and to blood vessels of skeletal muscle and skin. The prevertebral ganglia lie in the abdomen and the pelvis near the ventral surface of the bony vertebral column and consist mainly of the celiac (solar), superior mesenteric, aorticorenal, and inferior mesenteric ganglia. The terminal ganglia are few in number, lie near the organs they innervate, and include ganglia connected with the urinary bladder and rectum and the cervical ganglia in the region of the neck. In addition, small intermediate ganglia lie outside the conventional vertebral chain, especially in the thoracolumbar region. They are variable in number and location but usually are in close proximity to the communicating rami and the anterior spinal nerve roots.
Preganglionic fibers from the spinal cord may synapse with the neurons of more than 1 sympathetic ganglion. Their principal ganglia of termination need not correspond to the original level from which the preganglionic fiber exits the spinal cord. Many of the preganglionic fibers from the fifth to the last thoracic segment pass through the paravertebral ganglia to form the splanchnic nerves. Most of the splanchnic nerve fibers do not synapse until they reach the celiac ganglion; others directly innervate the adrenal medulla (see below).
Postganglionic fibers arising from sympathetic ganglia innervate visceral structures of the thorax, abdomen, head, and neck. The trunk and the limbs are supplied by the sympathetic fibers in spinal nerves. The prevertebral ganglia contain cell bodies whose axons innervate the glands and smooth muscles of the abdominal and the pelvic viscera. Many of the upper thoracic sympathetic fibers from the vertebral ganglia form terminal plexuses, such as the cardiac, esophageal, and pulmonary plexuses. The sympathetic distribution to the head and the neck (vasomotor, pupillodilator, secretory, and pilomotor) is by means of the cervical sympathetic chain and its 3 ganglia. All postganglionic fibers in this chain arise from cell bodies located in these 3 ganglia; all preganglionic fibers arise from the upper thoracic segments of the spinal cord, there being no sympathetic fibers that leave the CNS above the first thoracic level.
Pharmacologically, the chromaffin cells of the adrenal medulla resemble a collection of postganglionic sympathetic nerve cells. Typical preganglionic fibers that release ACh innervate these chromaffin cells, stimulating the release of epinephrine (EPI, adrenaline), in distinction to the NE released by postganglionic sympathetic fibers.
PARASYMPATHETIC NERVOUS SYSTEM. The parasympathetic nervous system consists of preganglionic fibers that originate in the CNS and their postganglionic connections. The regions of central origin are the midbrain, the medulla oblongata, and the sacral part of the spinal cord. The midbrain, or tectal, outflow consists of fibers arising from the Edinger-Westphal nucleus of the third cranial nerve and going to the ciliary ganglion in the orbit. The medullary outflow consists of the parasympathetic components of the VII, IX, and X cranial nerves.
The fibers in the VII (facial) cranial nerve form the chorda tympani, which innervates the ganglia lying on the submaxillary and sublingual glands. They also form the greater superficial petrosal nerve, which innervates the sphenopalatine ganglion. The autonomic components of the IX (glossopharyngeal) cranial nerve innervate the otic ganglia. Postganglionic parasympathetic fibers from these ganglia supply the sphincter of the iris (pupillary constrictor muscle), the ciliary muscle, the salivary and lacrimal glands, and the mucous glands of the nose, mouth, and pharynx. These fibers also include vasodilator nerves to these same organs. The X (vagus) cranial nerve arises in the medulla and contains preganglionic fibers, most of which do not synapse until they reach the many small ganglia lying directly on or in the viscera of the thorax and abdomen. In the intestinal wall, the vagal fibers terminate around ganglion cells in the myenteric and submucosal plexuses. Thus, in the parasympathetic branch of the autonomic nervous system, preganglionic fibers are very long, whereas postganglionic fibers are very short. The vagus nerve also carries a far greater number of afferent fibers (but apparently no pain fibers) from the viscera into the medulla. The parasympathetic sacral outflow consists of axons that arise from cells in the second, third, and fourth segments of the sacral cord and proceed as preganglionic fibers to form the pelvic nerves (nervi erigentes). They synapse in terminal ganglia lying near or within the bladder, rectum, and sexual organs. The vagal and sacral outflows provide motor and secretory fibers to thoracic, abdominal, and pelvic organs (see Figure 8–1).
ENTERIC NERVOUS SYSTEM. The processes of mixing, propulsion, and absorption of nutrients in the GI tract are controlled through the enteric nervous system (ENS). The ENS consists of both afferent sensory neurons and a number of motor nerves and interneurons that are organized principally into 2 nerve plexuses: the myenteric (Auerbach) plexus and the submucosal (Meissner) plexus.
The myenteric plexus, located between the longitudinal and circular muscle layers, plays an important role in the contraction and relaxation of GI smooth muscle. The submucosal plexus is involved with secretory and absorptive functions of the GI epithelium, local blood flow, and neuroimmune activities. The ENS incorporates components of the sympathetic and parasympathetic nervous systems and has sensory nerve connections through the spinal and nodose ganglia (see Figure 46–1). Parasympathetic preganglionic inputs are provided to the GI tract via the vagus and pelvic nerves. ACh released from preganglionic neurons activates nicotinic ACh receptors (nAChRs) on postganglionic neurons within the enteric ganglia. Excitatory preganglionic input activates both excitatory and inhibitory motor neurons that control processes such as muscle contraction and secretion/absorption. Postganglionic sympathetic nerves also synapse with intrinsic neurons and generally induce relaxation. Sympathetic input is excitatory (contractile) at some sphincters. Information from afferent and preganglionic neural inputs to the enteric ganglia is integrated and distributed by a network of interneurons. ACh is the primary neurotransmitter providing excitatory inputs between interneurons, but other substances such as ATP (via postjunctional P2X receptors), substance P (by NK3 receptors), and serotonin (via 5HT3 receptors) are also important in mediating integrative processing via interneurons.
The muscle layers of the GI tract are dually innervated by excitatory and inhibitory motor neurons with cell bodies primarily in the myenteric ganglia. ACh is a primary excitatory motor neurotransmitter released from postganglionic neurons. ACh activates M2 and M3 receptors in postjunctional cells to elicit motor responses. Pharmacological blockade of muscarinic cholinergic (mAChRs) receptors does not block all excitatory neurotransmission, however, because neurokinins (neurokinin A and Substance P) are also coreleased by excitatory motor neurons and contribute to postjunctional excitation. Inhibitory motor neurons in the GI tract regulate motility events such as accommodation, sphincter relaxation, and descending receptive relaxation. Inhibitory responses are elicited by a purine derivative (either ATP or β-nicotinamide adenine dinucleotide (β-NAD) acting at postjunctional P2Y1 receptors) and NO. Inhibitory neuropeptides, such as VIP and pituitary adenylyl cyclase-activating peptide (PACAP), may also be released from inhibitory motor neurons under conditions of strong stimulation.
COMPARISON OF SYMPATHETIC, PARASYMPATHETIC, AND MOTOR NERVES (FIGURE 8–2)
• The sympathetic system is distributed to effectors throughout the body, whereas parasympathetic distribution is much more limited.
• A preganglionic sympathetic fiber may traverse a considerable distance of the sympathetic chain and pass through several ganglia before it finally synapses with a postganglionic neuron; also, its terminals make contact with a large number of postganglionic neurons. The parasympathetic system has terminal ganglia very near or within the organs innervated and is generally more circumscribed in its influences.
• The cell bodies of somatic motor neurons reside in the ventral horn of the spinal cord; the axon divides into many branches, each of which innervates a single muscle fiber, so more than 100 muscle fibers may be supplied by 1 motor neuron to form a motor unit. At each neuromuscular junction, the axonal terminal loses its myelin sheath and forms a terminal arborization that lies in apposition to a specialized surface of the muscle membrane, termed the motor end plate (see Figure 11–3).
Figure 8–2 Wiring diagram for somatic motor nerves and the efferent nerves of the autonomic nervous system. The principal neurotransmitters, acetylcholine (ACh) and norepinephrine (NE), are shown in red. The receptors for these transmitters, nicotinic (N) and muscarinic (M) cholinergic receptors, t and adrenergic receptors, are shown in green.
• Somatic nerves innervate skeletal muscle directly at a specialized synaptic junction, the motor end plate, where ACh activates Nm receptors.
• Autonomic nerves innervate smooth muscles, cardiac tissue and glands. Both parasympathetic and sympathetic systems have ganglia, where ACh is released by the preganglionic fibers; ACh acts on Nn receptors on the postganglionic nerves. ACh is also the neurotransmitter at cells of the adrenal medulla, where it acts on Nn receptors to cause release of EPI and NE into the circulation.
• ACh is the dominant neurotransmitter released by postganglionic parasympathetic nerves and acts on muscarinic receptors. The ganglia in the parasympathetic system are near or within the organ being innervated with generally a one-to-one relationship between pre- and post-ganglionic fibers.
• NE is the principal neurotransmitter of postganglionic sympathetic nerves, acting on α- or β-adrenergic receptors. Autonomic nerves form a diffuse pattern with multiple synaptic sites. In the sympathetic system the ganglia are generally far from the effector cells (e.g., within the sympathetic chain ganglia). Preganglionic sympathetic fibers may make contact with a large number of postganglionic fibers.
RESPONSES OF EFFECTOR ORGANS TO AUTONOMIC NERVE IMPULSES. In most instances, the sympathetic and parasympathetic neurotransmitters can be viewed as physiological or functional antagonists (Table 8–1).
Table 8–1
Responses of Effector Organs to Autonomic Nerve Impulses
Most viscera are innervated by both divisions of the autonomic nervous system, and their activities on specific structures may be either discrete and independent or integrated and interdependent. For example, the effects of sympathetic and parasympathetic stimulation of the heart and the iris show a pattern of functional antagonism in controlling heart rate and pupillary aperture, respectively, whereas their actions on male sexual organs are complementary and are integrated to promote sexual function.
GENERAL FUNCTIONS OF THE AUTONOMIC NERVOUS SYSTEM. The ANS is the primary regulator of the constancy of the internal environment of the organism.
The sympathetic system and its associated adrenal medulla are not essential to life in a controlled environment, but the lack of sympathoadrenal functions becomes evident under circumstances of stress. In the absence of the sympathetic system: body temperature cannot be regulated when environmental temperature varies; the concentration of glucose in blood does not rise in response to urgent need; compensatory vascular responses to hemorrhage, oxygen deprivation, excitement, and exercise are lacking; and resistance to fatigue is lessened. Sympathetic components of instinctive reactions to the external environment are lost; and other serious deficiencies in the protective forces of the body are discernible. The sympathetic system normally is continuously active, the degree of activity varying from moment to moment and from organ to organ, adjusting to a constantly changing environment. The sympathoadrenal system can discharge as a unit. Heart rate is accelerated; blood pressure rises; blood flow is shifted from the skin and splanchnic region to the skeletal muscles; blood glucose rises; the bronchioles and pupils dilate; and the organism is better prepared for “fight or flight.” Many of these effects result primarily from or are reinforced by the actions of epinephrine secreted by the adrenal medulla.
The parasympathetic system is organized mainly for discrete and localized discharge. Although it is concerned primarily with conservation of energy and maintenance of organ function during periods of minimal activity, its elimination is not compatible with life. The parasympathetic system slows the heart rate, lowers the blood pressure, stimulates GI movements and secretions, aids absorption of nutrients, protects the retina from excessive light, and empties the urinary bladder and rectum.
NEUROTRANSMISSION
Nerve impulses elicit responses in smooth, cardiac, and skeletal muscles, exocrine glands, and postsynaptic neurons by liberating specific chemical neurotransmitters. Neurohumoral transmission relates to the transmission of impulses from postganglionic autonomic fibers to effector cells. Evidence supporting this concept includes:
• Demonstration of the presence of a physiologically active transmitter and its biosynthetic enzymes at appropriate sites
• Recovery of the transmitter from the perfusate of an innervated structure during periods of nerve stimulation but not (or in greatly reduced amounts) in the absence of stimulation
• Demonstration that the putative transmitter is capable of producing responses identical to responses to nerve stimulation
• Demonstration that the responses to nerve stimulation and to the administered transmitter candidate are modified in the same manner by various drugs, usually competitive antagonists
While these criteria are applicable for most neurotransmitters, including NE and ACh, there are now exceptions to these general rules. For example, NO has been found to be a neurotransmitter; however, NO is not stored in neurons and released by exocytosis. Rather, it is synthesized when needed and readily diffuses across membranes. Synaptic transmission in many instances may be mediated by the release of more than 1 neurotransmitter.
STEPS INVOLVED IN NEUROTRANSMISSION
The sequence of events involved in neurotransmission is of particular importance because pharmacologically active agents modulate the individual steps.
AXONAL CONDUCTION. At rest, the interior of the typical mammalian axon is ~70 mV negative to the exterior. In response to depolarization to a threshold level, an action potential is initiated at a local region of the membrane. The action potential consists of 2 phases. Following depolarization that induces an open conformation of the channel, the initial phase is caused by a rapid increase in the permeability and inward movement of Na+ through voltage-sensitive Na+ channels, and a rapid depolarization from the resting potential continues to a positive overshoot. The second phase results from the rapid inactivation of the Na+ channel and the delayed opening of a K+ channel, which permits outward movement of K+ to terminate the depolarization.
The transmembrane ionic currents produce local circuit currents such that adjacent resting channels in the axon are activated, and excitation of an adjacent portion of the axonal membrane occurs, leading to propagation of the action potential without decrement along the axon. The region that has undergone depolarization remains momentarily in a refractory state.
The puffer fish poison, tetrodotoxin, and a close congener found in some shellfish, saxitoxin, selectively block axonal conduction by blocking the voltage-sensitive Na+ channel and preventing the increase in Na+ permeability associated with the rising phase of the action potential. In contrast, batrachotoxin, an extremely potent steroidal alkaloid secreted by a South American frog, produces paralysis through a selective increase in permeability of the Na+ channel, which induces a persistent depolarization. Scorpion toxins are peptides that also cause persistent depolarization by inhibiting the inactivation process. Na+ and Ca2+ channels are discussed in more detail in Chapters 11, 14, and 20.
JUNCTIONAL TRANSMISSION.
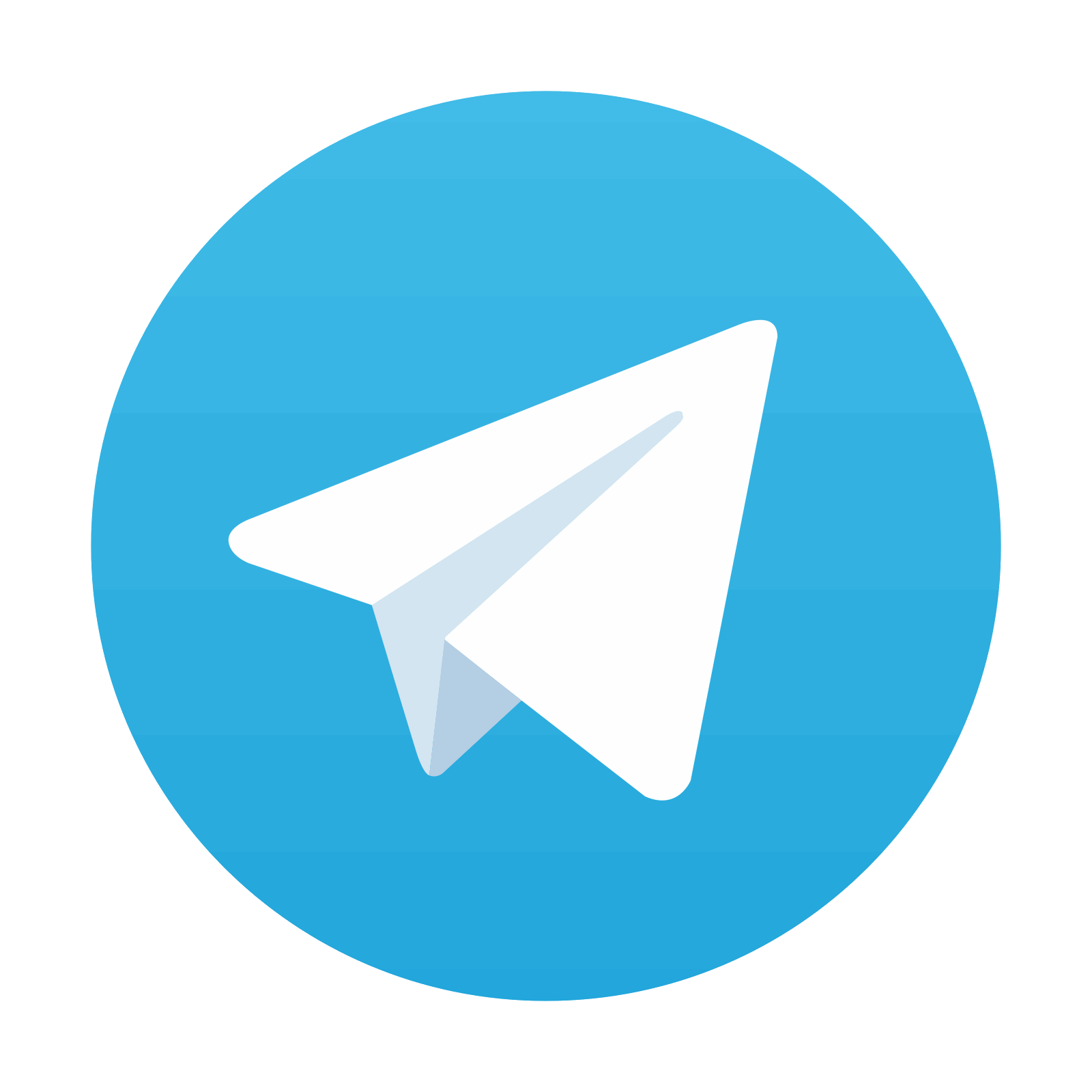
Stay updated, free articles. Join our Telegram channel

Full access? Get Clinical Tree
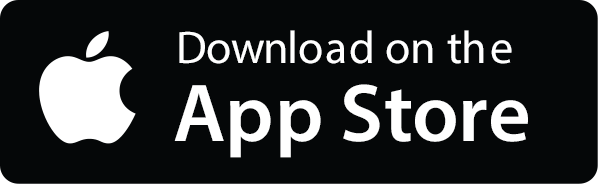
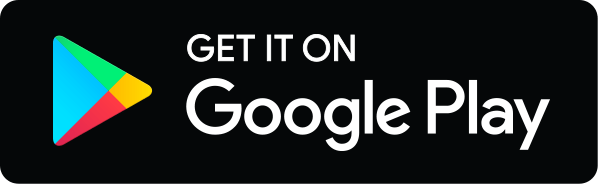