Neurotransmission and the Central Nervous System
ORGANIZATIONAL PRINCIPLES OF THE CNS
The brain is a complex assembly of interacting neurons and nuclei that regulate their own and each other’s activities in a dynamic fashion, generally through chemical neurotransmission. It is useful to examine the major anatomical regions of the CNS and their associations with specific neurotransmitter systems and the effect of pharmacological agents thereon.
CELLULAR ORGANIZATION OF THE BRAIN
Neurons. Neurons are classified according to function (sensory, motor, or interneuron), location, the identity of the transmitter they synthesize and release or the class or classes of receptor expressed on the cell surface. Neurons exhibit the cytological characteristics of highly active secretory cells with large nuclei: large amounts of smooth and rough endoplasmic reticulum; and frequent clusters of specialized smooth endoplasmic reticulum (Golgi complex), in which secretory products of the cell are packaged into membrane-bound organelles for transport from the perikaryon to the axon or dendrites. The sites of interneuronal communication in the CNS are termed synapses. Like peripheral “junctions,” central synapses are denoted by accumulations of tiny (50-150 nm) synaptic vesicles. The proteins of these vesicles have specific roles in transmitter storage, vesicle docking, and secretion and re-storage of transmitter.
Support Cells. Neurons in the CNS are outnumbered by support cells, including macroglia, microglia, vascular elements, the cerebrospinal fluid (CSF)-forming cells of the choroid plexus found within the intracerebral ventricular system, and the meninges, which cover the surface of the brain and comprise the CSF-containing envelope. Macroglia are the most abundant support cells; some are categorized as astrocytes (cells interposed between the vasculature and the neurons, often surrounding individual compartments of synaptic complexes). Astrocytes play a variety of metabolic support roles including furnishing energy intermediates and supplementary removal of neurotransmitters following release. The oligodendroglia, a second prominent category of macroglia, are myelin-producing cells. Myelin, made up of multiple layers of compacted membranes, insulate segments of axons bioelectrically and permit nondecremental propagation of action potentials. Microglia are derived from mesoderm and are related to the macrophage/monocyte lineage. Some microglia reside within the brain, while additional cells of this class may be recruited to the brain during periods of inflammation following either microbial infection or brain injury.
BLOOD-BRAIN BARRIER. The blood-brain barrier (BBB) is an important boundary between the periphery and the CNS that forms a permeability barrier to the passive diffusion of substances from the bloodstream into the CNS. The BBB diminishes the rate of access of many chemicals from plasma to the brain and is the localization of several drug export systems in the cells that constitute the BBB (see Chapter 5). An exception exists for lipophilic molecules, which diffuse fairly freely across the BBB and accumulate in the brain.
This barrier, nonexistent in the peripheral nervous system, is much less prominent in the hypothalamus and in several small, specialized organs (the circumventricular organs) lining the third and fourth ventricles of the brain: the median eminence, area postrema, pineal gland, subfornical organ, and subcommissural organ.
Selective barriers to permeation into and out of the brain also exist for small, charged molecules such as neurotransmitters, their precursors and metabolites, and some drugs. These diffusional barriers are viewed as a combination of the partition of solute across the vasculature (which governs passage by definable properties such as molecular weight, charge, and lipophilicity) and the presence or absence of energy-dependent transport systems (see Chapter 5). Important exceptions are the specific uptake transporters for amino acids, one of which contributes to the therapeutic utility of L-dopa in the treatment of Parkinson disease.
The brain clears metabolites of transmitters into the fluid-containing lateral ventricles by excretion via the acid transport system of the choroid plexus. Substances that rarely gain access to the brain from the bloodstream often can reach the brain when injected directly into the cerebrospinal fluid. Under certain conditions, it may be possible to open the BBB, at least transiently, to permit the entry of chemotherapeutic agents. Cerebral ischemia and inflammation also modify the BBB, increasing access to substances that ordinarily would not affect the brain.
CHEMICAL COMMUNICATION IN THE CNS
A central concept of neuropsychopharmacology is that drugs that influence behavior and improve the functional status of patients with neurological or psychiatric diseases act by enhancing or blunting the effectiveness of specific transmitters and channels.
Identified targets for centrally acting drugs include ion channels that mediate change in excitability induced by neurotransmitters, neurotransmitter receptors, and transport proteins that reaccumulate released transmitter. The transport proteins include those selective for NE, dopamine, and serotonin (NET, DAT, and SERT) that accumulate released transmitter and those that package it for reuse (e.g., VMAT2). Inhibition of reuptake increases the concentration and dwell time of transmitter in the synaptic space (e.g., as do serotonin selective reuptake inhibitors and cocaine). Inhibition of vesicular storage leads to depletion of releasable neurotransmitter (e.g., inhibition of VMAT2 and NE storage by reserpine).
An understanding at the systems level is required to assemble the structural and functional properties of specific central transmitter systems, linking the neurons that make and release a given neurotransmitter to its behavioral effects. The entire concept of animal models of human psychiatric diseases rests on the assumption that scientists can appropriately infer from observations of behavior and physiology (heart rate, respiration, locomotion, etc.) that the states experienced by animals are equivalent to the emotional states experienced by human beings expressing similar physiological changes.
IDENTIFICATION OF CENTRAL TRANSMITTERS. The criteria for identification of central transmitters require the same data set used to establish the transmitters of the autonomic nervous system (see Chapter 8):
• The transmitter must be shown to be present in the presynaptic terminals of the synapse and in the neurons from which those presynaptic terminals arise.
• The transmitter must be released from the presynaptic nerve concomitantly with presynaptic nerve activity.
• When applied experimentally to target cells, the effects of the putative transmitter must be identical to the effects of stimulating the presynaptic pathway.
• Specific pharmacological agonists and antagonists should mimic and antagonize, respectively, the measured functions of the putative transmitter with appropriate affinities and order of potency.
Many brain and spinal cord terminals contain more than 1 transmitter substance. Coexisting substances (presumed to be released together) may either act jointly on the postsynaptic membrane, or may act presynaptically to affect release of transmitter from the presynaptic terminal. Clearly, if more than 1 substance transmits information, no single agonist or antagonist would be expected to faithfully mimic or fully antagonize activation of a given presynaptic element. Co-storage and co-release of ATP and NE are an example. In addition to being released as a cotransmitter with other biogenic amines, ATP and adenosine have been shown to mediate diverse effects through interactions with purinergic receptors.
MANY NEUROTRANSMITTERS AND DRUGS ACT ON IDENTIFIED NEURONAL MACROMOLECULES
A number of molecular mechanisms link receptor occupancy to biological responses (see Chapter 3). The most commonly seen post-receptor events are changes in ion flux through channels formed by a multi-subunit receptor complex and the alteration of intracellular signaling via transmembrane receptor systems.
ION CHANNELS. The electrical excitability of neurons is achieved through modification of ion channels in neuronal plasma membranes. Na+, K+, and Ca2+, as well as Cl– anions, are regulated in their flow through highly discriminative ion channels. These channels are grouped structurally and termed voltage-dependent channels (Figure 14–1), and Cl– channels (Figure 14–2). Ligand-gated ion channels, regulated by the binding of neurotransmitters, form another distinct group of ion channels; a prominent example is the nicotinic acetylcholine receptor, a Na+ channel when activated (see Figures 11–1 and 11–2).
Figure 14–1 Structural similarities of voltage-dependent Na+, Ca2+, and K+ channels. A. The subunit in both Ca2+ and Na+ channels contains 4 subunits, each with 6 transmembrane hydrophobic domains. The hydrophobic regions that connect segments 5 and 6 in each domain form the pore of the channel. Segment 4 in each domain includes the voltage-sensor. (Adapted with permission from Catterall W. Neuron, 2000;26:13–25. © Elsevier.) B. The Ca2+ channel also requires several auxiliary small proteins (α2, β, γ, and δ); α2 and δ subunits are linked by a disulfide bond. Regulatory subunits also exist for Na+ channels. C. Voltage-sensitive K+ channels (Kv) and the rapidly activating K+ channel (KA) share a similar putative hexaspanning structure resembling in overall configuration 1 repeat unit within the Na+ and Ca2+ channel structure; the inwardly rectifying K+ channel protein (Kir) retains the general configuration of just loops 5 and 6. Regulatory t subunits (cytosolic) can alter Kv channel functions. Voltage-dependent channels provide for rapid changes in ion permeability along axons and within dendrites and for excitation-secretion coupling that releases neurotransmitters from presynaptic sites. The transmembrane Na+ gradient (~140 mM outside vs. ~14 mM inside the cell) means that increases in permeability to Na+ causes depolarization. In contrast, the K+ gradient (~4 mM outside the cell vs. ~120 mM inside) is such that increased permeability to K+ results in hyperpolarization. Changes in the concentration of intracellular Ca2+ (extracellular free Ca2+: 1.25 mM; intracellular Ca2+: resting ~100 nM, rising to ~1 μM when Ca2+ entry is stimulated) affects multiple processes in the cell and are critical in the release of neurotransmitters.
Figure 14–2 Three families of Cl– channel. Due to the Cl– gradient across the plasma membrane (~116 mM outside vs. 20 mM inside the cell), activation of Cl– channels causes an inhibitory postsynaptic potential (IPSP) that dampens neuronal excitability; inactivation of these channels can lead to hyperexcitability. There are 3 distinct types of Cl– channel:
• Ligand-gated channels are linked to inhibitory transmitters including GABA and glycine.
• ClC channels, of which 9 subtypes have been cloned, affect Cl-flux, membrane potential, and the pH of intracellular vesicles.
• Cystic fibrosis transmembrane conductance regulated (CFTR) channels bind ATP and are regulated by phosphorylation of serine residues.
(M, transmembrane domains; NBF, nucleotide-binding fold; R, regulatory [phosphorylation] domain.) (Reproduced with permission from Jentsch J. Chloride channels: A molecular perspective. Curr Opin Neurobiol, 1996;6:303–310. Copyright 3 Elsevier.)
Two other families of channels regulate ion fluxes: cyclic nucleotide–gated (CNG) channels, and transient receptor potential (TRP) channels. CNG channels consist of 2 groups:
• The CNG channels, which play important roles in sensory transduction for olfactory and photoreceptors
• The hyperpolarization-activated, cyclic nucleotide–gated (HCN) channels
HCN channels are cation channels that open with hyperpolarization and close with depolarization; upon direct binding of cyclic AMP or cyclic GMP, the activation curves for the channels are shifted to more hyperpolarized potentials. These channels play essential roles in cardiac pacemaker cells and presumably in rhythmically discharging neurons.
TRP channels are a family of hexaspanning receptors containing a cation-permeable pore. TRP channels respond to multiple stimuli and function in sensory physiology, including thermosensation, osmosensation, and taste. Members of the IRPV subfamily (vanilloid receptors) interact with ligands such as the endogenous cannabinoid anandamide and capsaicin, a “hot” or “irritant” component of chili peppers.
TRANSMEMBRANE RECEPTOR SYSTEMS. A variety of membrane receptors interact with neurotransmitters and neurohormones. These systems have been described in detail in Chapter 3.
CELL SIGNALING AND SYNAPTIC TRANSMISSION. Most cell-to-cell communication in the CNS involves chemical transmission that requires a series specializations for transmitter synthesis, storage, release, recognition, and termination of action (Figure 14–3; see also Figures 8–2, 8–3, and 8–5). In addition to primary neurotransmitters (usually vesicular), there are neurohormones neuromodulators, and neurotrophic factors that influence CNS function:
Figure 14–3 Transmitter release, action, and inactivation. Depolarization opens voltage-dependent Ca2+ channels in the presynaptic nerve terminal. (1) The influx of Ca2+ during an action potential (AP) triggers (2) the exocytosis of small synaptic vesicles that store neurotransmitter (NT) involved in fast neurotransmission. Released neurotransmitter interacts with receptors in the postsynaptic membranes that either couple directly with ion channels (3) or act through second messengers, such as (4) GPCRs. Neurotransmitter receptors in the presynaptic nerve terminal membrane (5) can inhibit or enhance subsequent exocytosis. Released neurotransmitter is inactivated by reuptake into the nerve terminal by (6) a transport protein coupled to the Na+ gradient (e.g., for DA, NE, and GABA), or by (7) degradation (ACh, peptides), or by (8) uptake and metabolism by glial cells (Glu). The synaptic vesicle membrane is recycled by (9) clathrin-mediated endocytosis. Neuropeptides and proteins are stored in (10) larger, dense core granules within the nerve terminal. These dense core granules are released from (11) sites distinct from active zones after repetitive stimulation.
NEUROHORMONES. The anterior and posterior pituitary secrete a variety of hormones and releasing factors. Hypothalamic neurons affecting the anterior pituitary release their hormones into the hypothalamic–adenohypophyseal portal blood system, which delivers them to the anterior pituitary, where they regulate the release of trophic hormones (i.e., ACTH, FSH, GH, LH, prolactin) into the blood. Other hypothalamic neurons project onto the posterior pituitary, where they release their peptide contents, oxytocin and arginine vasopressin (antidiuretic hormone) into the systemic circulation (see Chapters 25 and 38 and Figure 38–1).
NEUROMODULATORS.
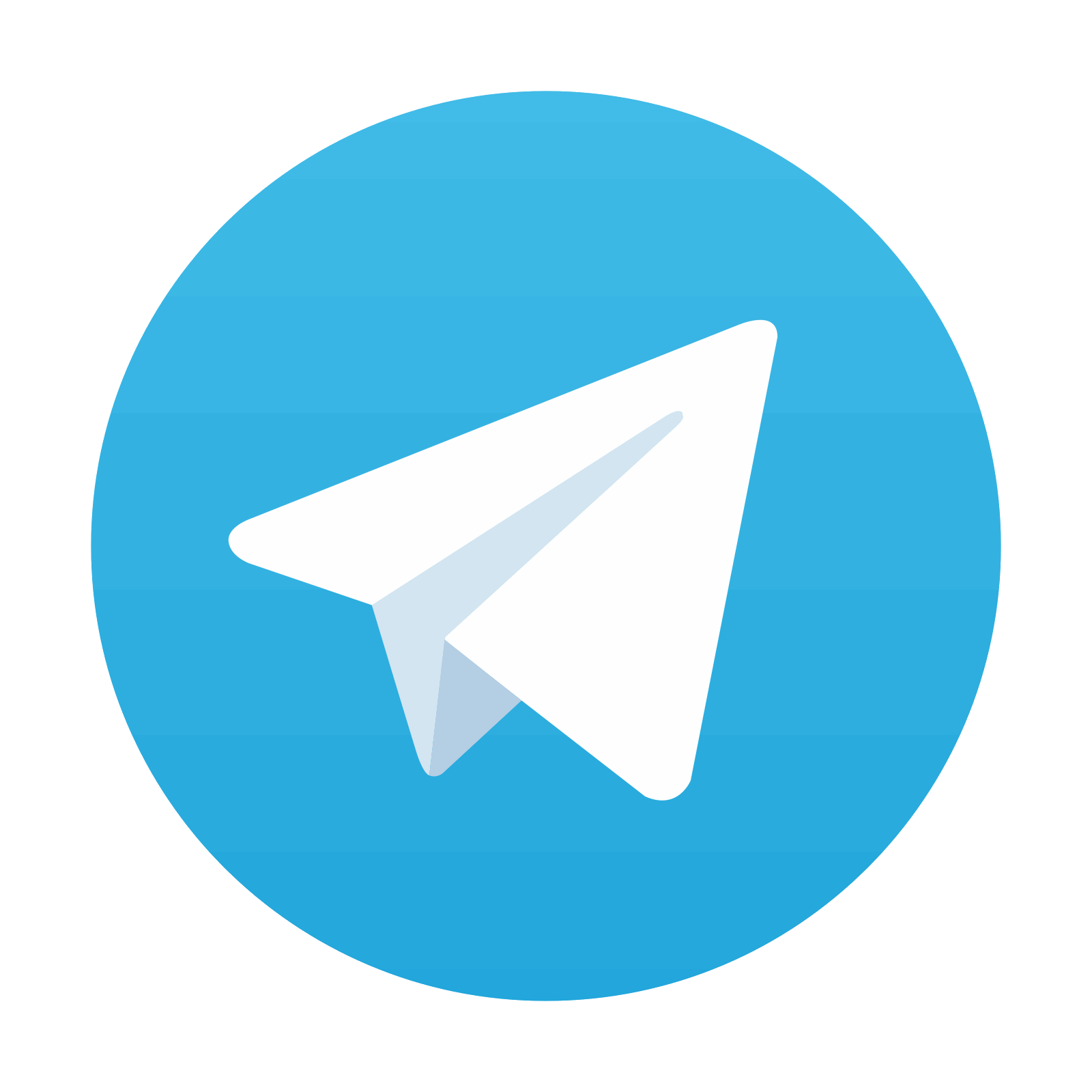
Stay updated, free articles. Join our Telegram channel

Full access? Get Clinical Tree
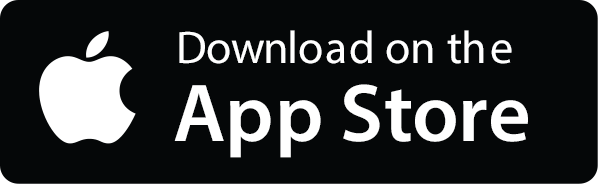
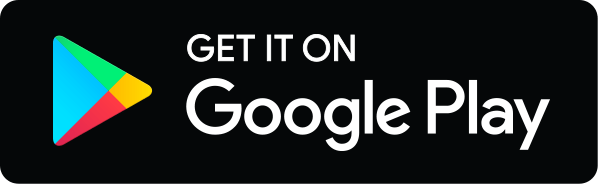