CHAPTER 10 Neuroanatomy of the Autonomic Nervous System
AUTONOMIC EFFERENTS: SYMPATHETIC, PARASYMPATHETIC, AND ENTERIC DIVISIONS
The ANS is composed of a sympathetic and parasympathetic division. These two divisions are discussed here, followed by a description of a third division of the ANS. This third division is the enteric nervous system, which is a complex network of neurons located within the wall of the gut. Typically the anatomy and functions of the parasympathetic and sympathetic divisions are described separately. However, remember that fibers of both divisions are tonically active, and in most cases do not function as either “on” or “off.” Although some tissues are predominantly or exclusively innervated by one division (e.g., sympathetic peripheral effector tissues), many tissues have a dual innervation. A balance of activity exists between the division fibers in these cases, such that when one division increases its activity, the other division decreases its activity. The central nervous system (CNS) has the ability to alter the balance of activity between these divisions. In addition, the sympathetic and parasympathetic divisions interact with the somatic nervous system and different hormonal systems to stabilize the internal environment and maintain homeostasis during normal conditions or emergency situations.
The ANS can be described best after certain characteristics common to both the sympathetic and parasympathetic divisions have been reviewed. The parasympathetic and sympathetic innervation of autonomic effectors (i.e., organs, vessels, glands) is organized differently than the innervation of skeletal muscle (Fig. 10-1). Although the axons of alpha and gamma motor neurons course directly to skeletal muscles, the innervation of ANS effectors requires a chain of two neurons (see Fig. 10-1), called the preganglionic and postganglionic neurons. The cell body of the preganglionic neuron always is located in the CNS; either in the spinal cord or brain stem (Fig. 10-2). The axon is thinly myelinated and immediately leaves the CNS within a specific ventral root of the spinal cord or within certain cranial nerves exiting the brain stem. The cell body of the postganglionic neuron is located in an autonomic ganglion that may be found in numerous places outside the CNS (see Fig. 10-2). The preganglionic neuron synapses with the postganglionic neuron within this ganglion. The axon of the postganglionic neuron is unmyelinated and innervates the effector. Both the preganglionic and postganglionic neurons frequently travel in components of the peripheral nervous system (PNS) (i.e., spinal nerves, cranial nerves) and are intermingled with afferents and somatic motor neurons of peripheral nerves. As stated, most effectors are innervated by both sympathetic and parasympathetic fibers (Table 10-1; see also Fig. 10-2). These fibers produce antagonistic but coordinated responses in the effectors. Descending input from higher integrative centers such as the hypothalamus and areas of the brain stem reaches the cell bodies of preganglionic fibers to regulate and adjust their activity. This descending input is a part of several specific visceral reflex pathways and also is used by higher centers to institute widespread bodily changes.
Table 10-1 Functions of the Sympathetic and Parasympathetic Divisions
Structure | Sympathetic Function (Adrenergic Receptors) | Parasympathetic Function |
---|---|---|
Eye | ||
Sphincter muscle | — | Contraction → Constricts |
Dilator muscle | Contraction → Dilates (α1) | — |
Ciliary muscle | Relaxes (slightly;far vision) (β2) | Contracts (near vision) |
Heart | ||
Rate and force of atrial and ventricular contractions | Increases (β1 and some β2) | Decreases |
Lungs | ||
Bronchial muscle | Relaxation → Dilates airway (β2) | Contraction → Constricts airway |
Glands | — | Stimulates secretion |
Skin | ||
Sweat glands | Increases secretion (cholinergic)1 | — |
Arrector pili muscle | Contracts (α1) | — |
Glands of Head | ||
Lacrimal | Vasoconstriction → Reduces secretion (α) | Increases secretion |
Salivary | Vasoconstriction → Secretion reduced and viscid (α);amylase secretion (β2) | Secretion increased and watery |
Blood Vessels | ||
Arterioles:coronary, skeletal muscle, pulmonary, abdominal viscera, renal | Contraction → Vasoconstriction (α1) Relaxation → Vasodilation (β2) | Vasodilation |
Skin, cerebral | Contraction → Vasoconstriction (α1) | — |
Systemic veins | Contraction → Vasoconstriction (α1) | — |
Relaxation → Vasodilation (β2) | — | |
Gastrointestinal Tract | ||
Motility/tone | Inhibits (α1,β2) | Stimulates |
Sphincters | Constricts (α1) | Relaxes |
Secretion | Vasoconstriction → Inhibits secretion (α1) | Stimulates |
Liver | Breaks down glycogen (glycogenolysis), gluconeogenesis, decreased bile secretion (α, β2) | Glycogen synthesis;increases bile secretion |
Gallbladder | Relaxes (β2) | Contracts |
Pancreas | Inhibits secretion of digestive enzymes, glucagon, and insulin (α2);increases secretion of insulin and glucagon (β2) | Secretion of digestive enzymes, insulin, and glucagon |
Spleen Capsule | Contraction (α1) | — |
Relaxation (β2) | — | |
Adipose | Lipolysis (β1);release of fatty acids into blood (β1, β3; β3 in brown adipose tissue) | — |
Kidney (juxtaglomerular cells) | Secretion of renin (β1) | — |
Urinary Bladder | ||
Detrusor muscle | Relaxes (minimal role) (β2) | Contracts |
Sphincter (nonstriated) | Contracts (α1) | Relaxes |
Sex Organs | Contracts smooth muscle of vas deferens, seminal vesicle, prostate → Ejaculation (α1) | Vasodilation → Erection of clitoris (females) and penis (males) |
Uterus | Variable (depends on hormonal status, pregnancy, and other factors) (α1, β2) | Minimal effect |
Adrenal Medulla | Stimulates secretion of epinephrine and norepinephrine (nicotinic ACh receptors) via preganglionic fibers | — |
Pineal | Increases melatonin synthesis and secretion (β) | — |
1 Sweat glands are an exception;their sympathetic fibers release ACh, which binds to cholinergic receptors.
From Benarroch EE et al. (1999). Medical neurosciences (4th ed.). New York: Lippincott Williams & Wilkins; Bray JJ et al. (1994). Lecture notes on human physiology (3rd ed.). Cambridge, UK: Blackwell Science; FitzGerald MJT & Folan-Curran J. (2002). Clinical neuroanatomy and related neuroscience (4th ed.). Philadelphia: WB Saunders; Tortora GJ & Grabowski SR. (2003). Principles of anatomy and physiology (10th ed.). New York: John Wiley & Sons, Inc.; Waxman SG. (2003). Clinical neuroanatomy (25th ed.). Chicago: McGraw-Hill.
Sympathetic Division
Preganglionic Sympathetic Neurons.
The cell bodies of the preganglionic sympathetic neurons are located in the spinal cord in all thoracic segments and in the upper two or three lumbar segments (see Fig. 10-2). Because of the distribution of these preganglionic cell bodies, the sympathetic division of the ANS often is called the thoracolumbar division. These preganglionic neurons comprise a heterogeneous population within the spinal cord. The dendritic arrangement of these neurons ranges from simple to complex arborizations. The cell bodies are of different shapes, and their size falls in a range between the size of smaller dorsal horn neurons and larger somatic motor neurons. Of the total membranous surface area of these neurons, the cell body of each composes a maximum of 15%, which likely indicates the importance of the dendritic surface area of that neuron (Cabot, 1990).
The cell bodies of the preganglionic neurons are found in four nuclei within the intermediate gray matter of the spinal cord (Fig. 10-3) (Cabot, 1990). The largest group of these cell bodies is the intermediolateral (IML) cell column that forms the lateral horn. Throughout this column are clusters of 20 to 100 neurons that are separated by distances ranging from 200 to 500 μm in the thoracic region and 100 to 300 μm in the lumbar region. The cell bodies are approximately 12 to 13 μm in diameter and histologically are similar to motor neurons (Harati, 1993). The diameters of the axons range from 2 to 5 μm, and their speed of conduction is approximately 3 to 15 m/sec. These fibers often are classified in the B group (see Chapter 9). At the T6 and T7 levels, the mean number of these cells is approximately 5000, but it has been shown that the number decreases with age at the rate of approximately 8% per decade (Harati, 1993).
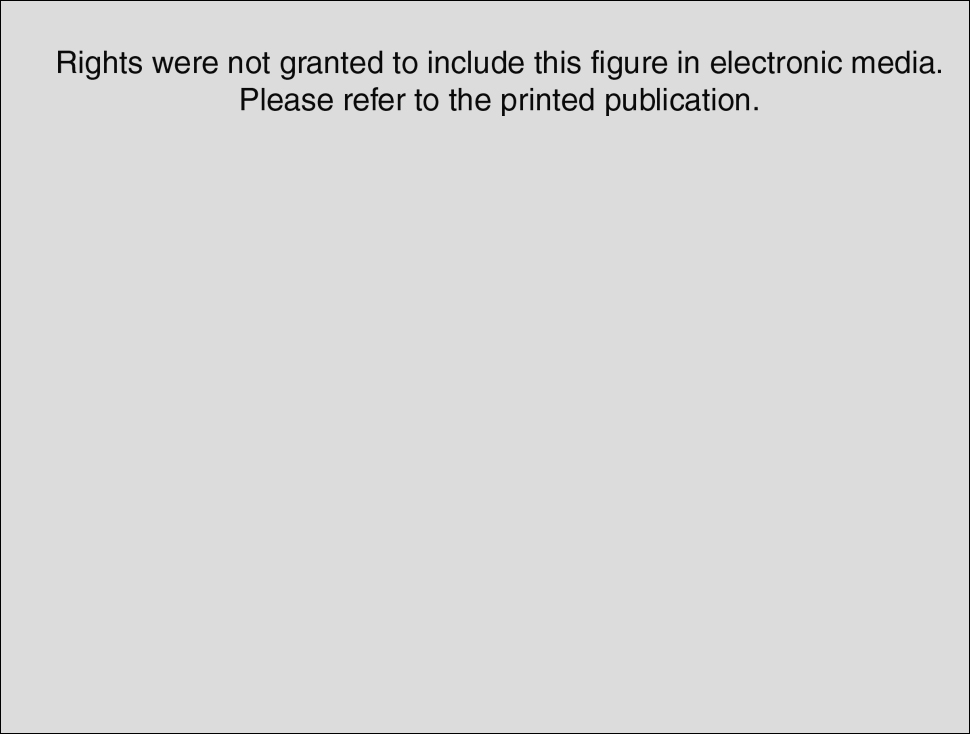
(From Cabot JB. [1990]. Sympathetic preganglionic neurons: Cytoarchitecture, ultrastructure, and biophysical properties. In AD Loewy & KM Spyer [Eds.]. Central regulation of autonomic functions. New York: Oxford University Press.)
The other three nuclear groups of preganglionic neurons have been described by Cabot (1990) and are the lateral funicular area (located lateral and dorsal to the intermediolateral group), the intercalated cell group (located medial to the IML column and possibly the same cluster of neurons typically called the intermediomedial group), and the central autonomic nucleus (located lateral and dorsal to the central canal). The combination of these groups forms a ladderlike structure in longitudinal sections in which the paired IML cell columns form the sides of the ladder and the interconnected central autonomic nucleus and intercalated cell group form the rungs (Fig. 10-3). The IML cell column is the origin of the vast majority of preganglionic fibers, but the other three nuclei also give rise to some preganglionic fibers. The four nuclei are the recipients of extensive input from higher centers such as the hypothalamus and brain stem nuclei. These sources release various neurotransmitters that have been identified as monoamines, neuropeptides, and amino acids. Although the anatomic characteristics of these four nuclei have been described, the exact functions of each specific nucleus still remain unclear.
Postganglionic Sympathetic Neurons.
According to the general rule of organization of the ANS, two neurons are necessary for the impulse to reach the effector. One is the preganglionic neuron, just discussed. The second neuron in the pathway to an autonomic effector is the postganglionic neuron. This neuron’s axon is classified as a group C fiber (see Chapter 9). Generally it is described as unmyelinated, with a diameter ranging from 0.3 to 1.3 μm and a slow conduction speed ranging from 0.7 to 2.3 m/sec (Carpenter and Sutin, 1983). The cell body is located outside the CNS in an autonomic ganglion. Unlike a sensory ganglion of cranial nerves and a dorsal root ganglion of spinal nerves, in which no synapses occur, an autonomic ganglion is the location of the synapse between the preganglionic and postganglionic neurons. Preganglionic fibers disseminate their information by diverging and synapsing on numerous postganglionic fibers. This principle of divergence is based on studies of the superior cervical ganglion of mammals. Results of different studies show preganglionic to postganglionic ratios of 1:4 (Loewy, 1990a), 1:15 to 1:20, and 1:196 in a human superior cervical ganglion (Williams et al., 1995). (The parasympathetic division also has been found to exhibit divergence, but to a lesser degree.) This divergence may allow the effects of sympathetic stimulation to be more widespread throughout the body and to be of greater magnitude.
The autonomic ganglion in which the synapse occurs may be one of a chain of ganglia (called the sympathetic chain, sympathetic trunk, or paravertebral ganglia) located near the vertebral bodies of the spinal column, or it may be a prevertebral ganglion, such as the celiac ganglion (see Fig. 10-2), found within one of the autonomic nerve plexuses.
These plexuses surround the large arteries in the abdominal and pelvic cavities. The ganglion, regardless of location, is encapsulated by connective tissue. The connective tissue capsule is continuous with the epineurium of the bundle of entering preganglionic neurons and the bundle of exiting postganglionic neurons. Within the capsule are predominantly multipolar, spheroidal-shaped postganglionic neurons (Fig. 10-4). These neurons consist of cell bodies that have diameters ranging from 25 to 50 μm and dendrites that branch in a complex pattern. These dendrites are the location where preganglionic neuron axons commonly synapse. Satellite cells (neuroglial cells) that are similar to those found in the dorsal root ganglia surround the cell bodies and dendrites. These cells provide support and help maintain the chemical environment. Interneurons are also located in the ganglion. One type, called small (cell bodies ranging in diameter from 15 to 20 μm) intensely fluorescent cells (SIFs), are present singly or in clusters. These cells contain the neurotransmitters epinephrine, serotonin, and dopamine (Hamill, 1996). When released, dopamine binds to postganglionic neurons, causing hyperpolarization. Another type of interneuron found in the ganglion is the small chromaffin cell, which also contains catecholamines. The exact difference between the chromaffin cell and SIF is unclear (Carpenter and Sutin, 1983; Harati, 1993; Williams et al., 1995).
Sympathetic Trunk.
Two sympathetic trunks are located in the body, each of which lies on the anterolateral side of the vertebral column (Fig. 10-5). They both extend from the base of the skull to the coccyx. The ganglia of the sympathetic trunks are also called the paravertebral ganglia because they lie next to the vertebral column. Inferiorly the two trunks join in the midline and terminate on the anterior surface of the coccyx as the ganglion impar.
Each sympathetic trunk shares important anatomic relationships with surrounding structures. In the neck it lies between the carotid sheath and prevertebral muscles, which cover the transverse processes (TPs) of the cervical vertebrae. It is found anterior to the heads of the ribs in the thorax, anterolateral to the bodies of the lumbar vertebrae in the abdomen, and medial to the anterior sacral foramina in the pelvis (Williams et al., 1995). As the name sympathetic chain ganglia implies, this structure consists of approximately 22 ganglia that are linked together by connective tissue surrounding ascending and descending fibers. The total number of ganglia does not correspond exactly to the number of spinal nerves because some of the ganglia have fused with one another. This fusion is most evident in the cervical region, where there are only three cervical ganglia. The thoracic portion of the sympathetic trunk includes 10 to 12 ganglia (70% of the time there are 11), the lumbar region exhibits 4 ganglia (although this number may vary), and 4 or 5 ganglia appear in the sacral region of the trunk. The union of the two sympathetic trunks forms the one coccygeal ganglion.
Autonomic fibers innervating peripheral blood vessels (including those in the skeletal muscles and skin), sweat glands, and arrector pili muscles of hair follicles travel in spinal nerves and subsequently peripheral nerves to innervate the appropriate effectors. These effectors are located in the area of distribution of each of the peripheral nerves. After entering the sympathetic trunk, preganglionic fibers associated with these effectors do one of three things (Fig. 10-6): ascend to synapse with postganglionic neurons in ganglia above T1 (for cervical nerves); synapse with postganglionic neurons at the level of entry into the trunk (i.e., T1 to L2 or L3 for those corresponding nerves); or descend to synapse with postganglionic neurons in ganglia below L2-3 (for lumbar and sacral nerves). From the sympathetic trunk the postganglionic fibers course through gray (these are unmyelinated fibers) rami communicantes (usually located proximal to the white rami), enter the spinal nerve at the location of its division into dorsal and ventral rami, and continue to the ANS effectors. Therefore the dorsal and ventral rami and subsequently formed peripheral nerves include sensory afferent fibers, motor neurons to skeletal muscle, and postganglionic sympathetic fibers. The ventral roots of T1 to L2-3 cord segments are unique in that they contain motor neurons to skeletal muscle and also preganglionic sympathetic fibers.
Sympathetic Preganglionic and Postganglionic Fibers.
Sympathetic preganglionic fibers sending nerve impulses to effectors in the head enter the sympathetic trunk, ascend to the superior cervical ganglion, and synapse with postganglionic neurons. The postganglionic fibers course with large blood vessels to reach effectors located in the head region (Fig. 10-7, A). Such effectors include glands, the smooth muscle of blood vessels, and the smooth muscle of the eye. Some preganglionic fibers sending impulses to smooth muscle, cardiac muscle, and glands of the thorax also ascend on entering the trunk and synapse at rostral levels, whereas others synapse with postganglionic fibers at the level of entry. These postganglionic fibers leave the chain as branches that merge with other nerve fibers, including parasympathetic vagal fibers, to form plexuses innervating the heart and lungs. Abdominal and pelvic effectors are innervated in a different manner than the effectors of the head, thorax, and cutaneous regions. Preganglionic fibers enter the sympathetic trunk via white rami communicantes but do not synapse in the chain ganglia. Instead they pass through the chain ganglia and emerge as a collection of fibers called sympathetic splanchnic (referring to the viscera) nerves. These nerves course inferiorly in an anteromedial direction, pass through the diaphragm, and end in various prevertebral ganglia. Here they synapse on postganglionic neurons that then continue to the effectors of the abdominal and pelvic cavities (Fig. 10-7, B). The sympathetic prevertebral ganglia are enmeshed in plexuses of sympathetic and parasympathetic fibers and are located near large arteries found in the abdominal cavity. Examples are the celiac, superior mesenteric, aorticorenal, and inferior mesenteric ganglia.
On entering the sympathetic trunk, a preganglionic neuron may either ascend or descend and, in each case, subsequently synapse in more than one ganglion. A preganglionic neuron also may synapse at the entry level and send collateral branches up or down to other ganglia. However, less than 2% of the neurons send a branch both up and down (Cabot, 1990). In all cases described thus far, a preganglionic neuron has synapsed with a postganglionic neuron. However, a notable exception is the innervation of the medulla of the adrenal gland. The adrenal medulla develops from the same embryonic neural crest as postganglionic neurons. Although the medullary chromaffin cells do not resemble postganglionic neurons in appearance, they do function in a similar manner. Preganglionic neurons innervate the medulla directly, which in turn releases epinephrine and some norepinephrine into the blood stream. These neurotransmitters circulate throughout the body, stimulating effectors and assisting in the overall sympathetic response.
A summary of the various sympathetic nerve pathways is provided in Figures 10-6 and 10-8.
Specific Regions of the Sympathetic Trunk
Cervical sympathetic trunk.
The fusion of the eight cervical ganglia results in three distinct ganglia in the region of the neck (Figs. 10-9, A and 10-10; see also Fig. 10-5). These are known as the superior, middle, and cervicothoracic (stellate) ganglia. (Twenty percent of the time the T1 ganglion is separate, in which case the cervicothoracic ganglion is called the inferior cervical ganglion [Harati, 1993].) The superior ganglion (Fig. 10-11, A; see also Figs. 10-9, A and 10-10) is the largest of the three and lies high in the neck adjacent to vertebrae C2 and C3, anterior to the longus capitis muscle and posterior to the cervical part of the internal carotid artery. It is also in the vicinity of the internal jugular vein and the glossopharyngeal, vagus, spinal accessory, and hypoglossal cranial nerves (Williams et al., 1995). The proximity of the ganglion to these nerves may account for the autonomic effects seen when these nerves are lesioned in this location (Cross, 1993b). The ganglion is formed by the fusion of the first four cervical ganglia, is 2.5 to 3.0 cm long, and includes more than 1 million neurons (Carpenter and Sutin, 1983; Harati, 1993; Williams et al., 1995). Postganglionic fibers leaving this ganglion course to various regions. Some ascend as perivascular plexuses on the internal and external carotid arteries. A large branch (internal carotid nerve) from the superior cervical ganglion ascends with the internal carotid artery and divides into branches that form the internal carotid plexus (see Fig. 10-9, A) (Williams et al., 1995). This plexus, which surrounds the artery and innervates its wall, continues to travel with that artery, and within the cranial cavity the fibers innervate the autonomic effectors. Examples of these effectors are the dilator pupillae muscle of the eye, the superior tarsal muscle (Müller’s muscle) of the eyelid, and sweat glands in the lateral part of the forehead (Watson and Vijayan, 1995; Salvesen, 2001). In addition, some are sympathetic vasoconstrictor fibers and innervate cerebral branches of the internal carotid artery. Other postganglionic fibers leave the ganglion as medial, lateral, and anterior branches and course directly to effectors. The lateral branches include slender filaments that communicate with the glossopharyngeal, vagus, and hypoglossal nerves; and gray rami that join the first four cervical spinal nerves. The latter travel with those spinal nerves to effectors in the areas of distribution of the nerves. The medial branches include laryngopharyngeal and cardiac (efferent) branches. The anterior branches travel with the common and external carotid arteries. Fibers continue with branches of the external carotid artery to innervate such structures as the facial sweat glands by traveling with terminal branches of the trigeminal nerve (cranial nerve [CN] V) (Williams et al., 1995).
The middle cervical ganglion (see Figs. 10-9, A and 10-10), formed by the fusion of the C5 and C6 ganglia, is the smallest (0.7 to 0.8 cm), and sometimes is absent. It lies adjacent to the C6 vertebra and near the inferior thyroid artery, which is a branch of the thyrocervical trunk. Postganglionic fibers include gray rami that enter the C5 and C6 spinal nerves (sometimes the fourth and seventh), thyroid branches, and the largest sympathetic cardiac branch. The ganglion is continuous with the cervicothoracic ganglion by anterior and posterior branches. Although there is variation to this connection, typically the posterior branch splits around the vertebral artery as it descends to the cervicothoracic ganglion; the anterior component descends and loops around the first part of the subclavian artery before connecting with the cervicothoracic ganglion. This loop is called the ansa subclavia (see Fig. 10-11, B).
The cervicothoracic (stellate) ganglion (see Figs. 10-9, A and 10-11, B) is formed by the fusion of the seventh, eighth, and first thoracic ganglia (and sometimes even the second, third, and fourth thoracic ganglia). It is approximately 2.8 cm long and is located between the base of the TP of C7 and the neck of the first rib lying on or just lateral to the longus colli muscle. A small detached portion of the stellate ganglion (or middle cervical ganglion), called the vertebral ganglion, may be present on the sympathetic trunk near the origin of the vertebral artery.
Some postganglionic fibers of the stellate (cervicothoracic) ganglion travel in gray rami communicantes to enter the C7, C8, and T1 spinal nerves, whereas others form a cardiac branch. Some other fibers form branches that course on the subclavian artery and its branches. One of these is large, and because it ascends with the vertebral artery (see Figs. 10-9, A and 10-11, B), frequently it is called the vertebral nerve (see Chapter 5 and Fig. 5-22). This nerve is joined by other branches and forms the vertebral plexus. Deep rami communicantes branch from the vertebral plexus and travel with ventral rami of the first five or six cervical spinal nerves. This plexus travels into the cranial cavity on the vertebral artery and continues on the basilar artery (and its branches) as far as the posterior cerebral artery, where it continues anteriorly to join the internal carotid artery plexus. Some consider the vertebral plexus to be the major continuation of the sympathetic system into the cranium (Williams et al., 1995).
Although the cervical sympathetic chain has no white rami communicantes associated with it, numerous gray rami may be associated with each spinal nerve. For example, as many as five gray rami may connect with the C7 spinal nerve and the C8 spinal nerve may receive as many as three to six gray rami (Carpenter and Sutin, 1983). Also, cervical gray rami may pierce the longus capitis and scalenus anterior muscles as they course to the cervical spinal nerves (Williams et al., 1995).
Thoracic sympathetic trunk.
Eleven small ganglia usually (70% of the time) are found in the thoracic sympathetic chain (Fig. 10-12; see also Fig. 10-9, B). (Note that 80% of the time the T1 ganglion is fused with the inferior cervical ganglion, in which case the succeeding ganglion is still named the second.) Each ganglion includes 90, 000 to 100, 000 neurons (Harati, 1993). The thoracic chain lies adjacent to the heads of the ribs and posterior to the costal pleura. In this region of the chain, white rami communicantes, as well as the gray rami communicantes, are clearly evident (see Fig. 10-12, B). The white rami lay more distal (lateral) than the gray rami, and two or more rami may be connected to one spinal nerve. A mixed ramus formed by the fusion of the gray and white rami sometimes may be present. Postganglionic fibers originating from all thoracic ganglia enter the thoracic spinal nerves and travel with them to effectors. Some postganglionic fibers from the T1 to T5 ganglia form direct branches to the thoracic aortic, cardiac, and pulmonary plexuses of the thorax. Other large branches of the T5 to T12 ganglia supply the aorta and are associated with the three splanchnic nerves involved with the sympathetic innervation of the abdominal and pelvic viscera. These splanchnic nerves consist of preganglionic fibers that synapse in prevertebral ganglia located in the abdominal cavity.
The greater splanchnic nerve (see Figs. 10-9, B and 10-12, A) contains preganglionic fibers exiting from the T5 to T9 or T10 ganglia. As it descends obliquely on the vertebral bodies, it sends branches to the descending thoracic aorta and then pierces the diaphragm. It courses to the medulla of the adrenal gland, the celiac ganglion, and sometimes the aorticorenal ganglion. In the ganglia, the preganglionic fibers of the greater splanchnic nerve synapse on postganglionic neurons. The lesser splanchnic nerve consists of preganglionic fibers from the T9 and T10 or T10 and T11 ganglia and is present 94% of the time. It traverses the diaphragm with the greater splanchnic nerve and enters the abdominal cavity to synapse in the aorticorenal ganglion (the detached lower part of the celiac ganglion). The third splanchnic nerve is the lowest or least splanchnic nerve and is present 56% of the time. Sometimes this nerve is called the renal nerve and emerges from the T12 (or lowest) ganglion to terminate in many small ganglia located in the renal plexus (Harati, 1993; Williams et al., 1995). From these prevertebral ganglia, postganglionic fibers participate in the formation of the various perivascular plexuses as they travel to abdominal effectors.
Lumbar sympathetic trunk.
The thoracic sympathetic trunk passes posterior to the medial arcuate ligament (or sometimes through the crura of the diaphragm) to become continuous with the lumbar sympathetic trunk found within the abdominal cavity. The trunk has been described as consisting of four interconnected lumbar ganglia (each of which contains 60, 000 to 85, 000 neurons) (Harati, 1993; Williams et al., 1995). However, other data indicate that the number of ganglia varies (Mitchell, 1953; Rocco, Palombi, and Raeke, 1995). Murata et al. (2003) studied cadaveric specimens and looked at the anatomic variations of the ganglia and associated rami. They found that the number of ganglia on one side ranged from 2 to 6 (mean, 3.9) and that the majority of ganglia were located on the L2 and L3 vertebrae. Typically no ganglia were found on the L1 and L4 vertebrae. Approximately 40% of the cadavers showed the same number of ganglia on both sides, and those were asymmetrically located. In addition, Murata et al. (2003) found 5 to 12 rami communicantes per side (mean of 7.2) and noted that more than one rami communicantes could connect to a lumbar ventral ramus and that a lumbar ventral ramus often received rami communicantes from more than one ganglion. In fact, one third of the ganglia associated with the L2 and L3 vertebrae included rami that traveled to three spinal nerves. Rami associated with the L1-5 spinal nerves were measured, and it was found that the ramus of the L4 spinal nerve was significantly similar in length to the L2 and L3 rami. Although this is contrary to what would be expected because the sympathetic chain lies closer to the intervertebral foramina (IVFs) at the L4-5 vertebral levels, it may be due to the fact that there are fewer ganglia at the L4 level and the rami have farther to travel to reach a more superiorly located ganglion. The L1 rami also were significantly longer than the other lumbar rami, most likely for the same reason. The rami of L5 were significantly shorter than those of L1-4. The presence of anatomic variations in the rami and ganglia in this region may be of importance relative to the nociceptive pathway for lower lumbar structures. Based on studies in rats that may apply to humans as well, it has been suggested that nociceptive input from low back structures is carried in two different pathways. Some nociceptive fibers from low back pain generators travel in a segmental fashion, directly within spinal nerves, and terminate in local cord segments. Other nociceptive fibers terminate centrally in a nonsegmental fashion. These course in lower lumbar spinal nerves, enter the sympathetic chain and ascend, and then exit the chain through more superiorly located rami connected to the L2 spinal nerve. These fibers terminate in the lower region of the thoracolumbar cord segments associated with the sympathetic division (Murata et al., 2003). If this pathway is present in humans it may contribute to the wide referral pattern seen in lower lumbar intervertebral discs, lower lumbar zygopophysial (Z) joints, and sacroiliac joint pain conditions (Murata et al., 2000) (see Chapters 7 and 11 for further information).
The lumbar trunk lies adjacent to the anterolateral aspect of the upper lumbar vertebrae and becomes more posterior relative to lower lumbar vertebrae (Murata et al., 2003). It also lies adjacent to the medial margin of the psoas major muscle (Fig. 10-13; see also Fig. 10-9, C). The inferior vena cava, right ureter, and lumbar lymph nodes lie anterior to the right sympathetic trunk. The left sympathetic trunk lies posterior to the aortic lymph nodes and lateral to the aorta. These relationships are important surgically because lumbar ganglia may have to be removed (lumbar sympathectomy) to treat certain arterial diseases of the lower extremities (Moore, 1980).
White rami communicantes are associated with the upper two or three ganglia. The gray rami are long (see previous section) as they course with lumbar arteries along the sides of the vertebral bodies to join each lumbar spinal nerve (see Fig. 10-13). The majority of these postganglionic fibers are thought to use the femoral nerve, obturator nerve, and their muscular and cutaneous branches to reach the adjoining blood vessels and cutaneous effectors. In a manner similar to the lower thoracic ganglia, some preganglionic fibers pass through the lumbar ganglia to form lumbar splanchnic nerves. In general, each lumbar splanchnic nerve corresponds to its ganglion of the same number, although the second lumbar splanchnic nerve receives additional fibers from the third ganglion, and the third lumbar splanchnic nerve also receives a contribution from the fourth ganglion. The four splanchnic nerves course into the abdomen and become part of the abdominal plexuses: the first splanchnic nerve courses within the celiac, abdominal aortic (intermesenteric), and renal plexuses; the second splanchnic nerve contributes to the inferior part of the abdominal aortic (intermesenteric) plexus; the third splanchnic nerve travels within the superior hypogastric plexus (see Figs. 10-9, C and 10-13); the fourth splanchnic nerve contributes to the lowest portion of the superior hypogastric plexus (or hypogastric nerve) (Williams et al., 1995). The lumbar portion of the sympathetic trunk passes inferiorly, posterior to the common iliac vessels, and becomes continuous with the pelvic portion of the trunk.
Pelvic sympathetic trunk.
The pelvic chain consists of four or five ganglia that lie on the anterior aspect of the sacrum. Each side unites to form the ganglion impar on the anterior aspect of the coccyx (Fig. 10-14; see also Fig. 10-9, C). Postganglionic fibers leave the chain in gray rami to enter the sacral spinal nerves and coccygeal nerve. Fibers destined for blood vessels in the leg and foot course primarily with the tibial nerve to connect subsequently with (and supply) the popliteal artery and its branches in the leg and foot. Other fibers travel with the pudendal and gluteal nerves to the internal pudendal artery and gluteal arteries. In addition, some fibers from the first two sacral ganglia send postganglionic branches into the inferior hypogastric plexus (or hypogastric nerve).
Plexuses of the Autonomic Nervous System.
The cardiac, pulmonary, celiac, and hypogastric plexuses are the major plexuses (Williams et al., 1995), although secondary plexuses may emanate from each one. The cardiac plexus, which is divided into deep and superficial parts, consists of cardiac branches from cervical and upper thoracic ganglia mixed with cardiac branches of the vagus nerve (see Fig. 10-9, B). A continuation of the cardiac plexus forms secondary coronary and atrial plexuses. The pulmonary plexus is an extension of fibers of the cardiac plexus that course with the pulmonary arteries to the lungs. Therefore the cardiac and pulmonary plexuses consist of the same sympathetic and vagal branches.
The celiac plexus is the largest autonomic plexus (see Fig. 10-9, C). It is located at the level of the T12 and L1 vertebrae and surrounds the celiac artery and the base of the superior mesenteric artery. This plexus is a dense fibrous network that interconnects the paired celiac ganglia. Mingling with the celiac plexus and ganglia are the greater and lesser splanchnic nerves and also branches of the vagus and phrenic nerves (Williams et al., 1995). Numerous subsidiary ganglia and fibers extend from the celiac plexus and course along abdominal blood vessels to autonomic effectors. These fibers and ganglia form (in some cases with the help of the lesser and least splanchnic nerves) plexuses that include the phrenic, hepatic, gastric, splenic, testicular, ovarian, superior mesenteric (to small and large intestines), renal, inferior mesenteric (to lower GI tract), and abdominal aortic (intermesenteric). The latter three plexuses also include lumbar splanchnic nerves. The hepatic plexus is the largest of these smaller plexuses and supplies innervation to the liver, gallbladder, bile ducts, stomach, duodenum, and pancreas. It contains afferent and efferent sympathetic branches and parasympathetic fibers. Consequently the celiac plexus and its secondary plexuses are responsible for the innervation of the abdominal viscera. Anterior to the bifurcation of the aorta at the level of the L4 to L5 vertebral bodies, the superior hypogastric plexus (Fig. 10-13, A, and see Fig. 10-9, C) is formed by the third and fourth lumbar splanchnic nerves and fibers of the aortic plexus. This plexus sends branches to the testicular, ureteric, ovarian, and common iliac plexuses. As the superior hypogastric plexus descends into the pelvic cavity, it divides into left and right hypogastric nerves that continue caudally to form the inferior hypogastric (pelvic) plexuses (see Fig. 10-9, C). Within the pelvis, pelvic splanchnic parasympathetic fibers (originating from the S2-4 cord segments) join each inferior hypogastric plexus. Preganglionic sympathetic fibers in the plexus originate in the T10-L2 cord segments and travel as lumbar splanchnics or postganglionic fibers of the lumbar and sacral chain ganglia. Extensions of the inferior hypogastric plexus, which include the middle rectal, prostatic, uterovaginal, and vesical plexuses, continue along the branches of the internal iliac artery to innervate autonomic effectors of the pelvis. The ANS innervation of the most clinically important effectors of the pelvis is discussed later in this chapter.
Parasympathetic Division
The parasympathetic division generally is concerned with conserving and restoring energy. It is coordinated with the sympathetic division in the dual and antagonistic innervation of autonomic effectors (see Table 10-1). However, there is no parasympathetic innervation of autonomic effectors located in the extremities and body wall (i.e., sweat glands, arrector pili muscles, peripheral blood vessels). Because the sympathetic division has been nicknamed the fight-or-flight division, the parasympathetic division could appropriately be named the rest-and-digest division, because in general, parasympathetic activation results in decreased heart rate and increased GI glandular secretion and peristalsis. In contrast to the widespread control by the sympathetic system, the parasympathetic division controls effectors at a more local level. This relates to the overall pattern of organization of the parasympathetic division of the ANS. Compared with the sympathetic division, each parasympathetic preganglionic neuron synapses with fewer postganglionic neurons, and the location of the parasympathetic ganglia is near, or frequently within, the wall of the effector organ. Although the ganglia are the site of synapses between preganglionic and postganglionic neurons, afferent fibers, postganglionic sympathetic fibers, and even branchial arch efferent fibers can course through them.
The parasympathetic division also is called the craniosacral division. As with the thoracolumbar (sympathetic) division, this name refers to the location of the cell bodies of preganglionic neurons (see Fig. 10-2). These cell bodies are located in autonomic nuclei of the brain stem (cranio) and in the second, third, and fourth sacral cord segments (sacral). The parasympathetic efferents of the sacral cord course within the ventral roots and subsequently form pelvic splanchnic nerves. These nerves do not use the sympathetic trunk. Axons of the cell bodies located in the brain stem leave the brain stem in the oculomotor, facial, glossopharyngeal, and vagus cranial nerves. Although numerous branches of various cranial nerves include these parasympathetic efferents, only the major branches are described. The somatic functions of the four cranial nerves are not discussed because this chapter is devoted to autonomic effectors.
Cranial Portion of the Parasympathetic Division
Oculomotor nerve.
The oculomotor nerve (CN III) emerges from the ventral surface of the midbrain of the brain stem (see Chapter 9, Fig. 9-20). The origin of the autonomic efferents is in the Edinger-Westphal nucleus, which is located in the midbrain ventral to the cerebral aqueduct of Sylvius. These preganglionic fibers course within the oculomotor nerve to the ciliary ganglion, where they synapse with postganglionic neurons (Fig. 10-15). This ganglion is less than 2 mm long and contains 3000 multipolar neurons (Harati, 1993). It is located in the orbit just anterior to the superior orbital fissure. Postganglionic fibers course in the short ciliary nerves to the eye and travel between the choroid and sclera of the eye wall. Here the fibers innervate the smooth muscle of the iris (sphincter pupillae) and ciliary body (ciliary muscle). The sphincter muscle of the iris functions to constrict the pupil during the pupillary light reflex and during the accommodation-convergence reflex. Contraction of the ciliary muscle occurs during the accommodation-convergence reflex. The result of this contraction is a thickening of the lens, which improves near vision.
Facial nerve.
The facial nerve (CN VII) also contains preganglionic fibers. The cell bodies of these fibers are located in the superior salivatory nucleus. This nucleus is located in the caudal part of the pons near the facial motor nucleus. The fibers emerge from the pontomedullary junction in the nervus intermedius portion of CN VII (see Chapter 9, Fig. 9-20). Some of the fibers travel in the chorda tympani nerve, which in turn joins the lingual branch of the mandibular division of the trigeminal nerve (CN V). These preganglionic fibers continue to the submandibular (sublingual) ganglion, where they synapse with postganglionic neurons (see Fig. 10-15). The postganglionic fibers are secretomotor and course to minor salivary glands, as well as to the larger submandibular and sublingual salivary glands. (It has been reported also that stimulation of the chorda tympani nerve results in vasodilation in the salivary glands [Williams et al., 1995].) In addition to the preganglionic fibers en route to the submandibular ganglion, other secretomotor preganglionic fibers from the lacrimal portion of the superior salivatory nucleus course in the greater petrosal nerve to the pterygopalatine ganglion (see Fig. 10-15). This ganglion is approximately 3 mm long and contains 56, 500 compactly arranged neurons (Harati, 1993). It is located in the pterygopalatine fossa behind and below the orbit. Postganglionic fibers exit from here and travel in the zygomatic nerve (a branch of the maxillary division of the trigeminal nerve) and terminate in the lacrimal gland. Other secretomotor branches of the pterygopalatine ganglion course to the glands and mucous membranes of the palate and nasal mucosa.
Glossopharyngeal nerve.
The glossopharyngeal nerve is CN IX. Preganglionic neurons that course in this nerve originate in the inferior salivatory nucleus, which is located caudal to the superior salivatory nucleus. CN IX emerges as three to five rootlets from the dorso-olivary sulcus on the lateral side of the medulla of the brain stem (see Chapter 9, Fig. 9-20). The preganglionic fibers travel in the lesser petrosal nerve to the otic ganglion, where they synapse with postganglionic neurons (Fig. 10-16, A). The postganglionic fibers are secretomotor, and the axons of these neurons travel in the auriculotemporal nerve (a branch of the mandibular division of the trigeminal nerve) to reach the parotid gland that they innervate. Evidence shows that stimulation of the lesser petrosal nerve results in vasodilation in the parotid gland, as well as serous secretion (Williams et al., 1995).
Vagus nerve.
The vagus nerve (CN X) also conveys parasympathetic fibers. In fact 75% of the total parasympathetic efferents are carried by the vagus nerve. This nerve is closely related to the glossopharyngeal nerve both anatomically and functionally. Just caudal to the glossopharyngeal nerve the vagus nerve emerges as 8 to 10 rootlets from the dorso-olivary sulcus of the medulla (see Chapter 9, Fig. 9-20). Most preganglionic fibers (some of which are extremely long) arise from the dorsal motor nucleus, which is a column of cell bodies located in the medulla of the brain stem. Some (possibly the majority) of preganglionic fibers destined for cardiac muscle originate in or near the nucleus ambiguus (Loewy and Spyer, 1990; Noback, Strominger, and Demarest, 1991; Wang, Holst, and Powley, 1995; Williams et al., 1995; Kiernan, 1998; Iversen, Iversen, and Saper, 2000; Nolte, 2002), which is located in the medulla ventral to the dorsal motor nucleus. However, the nucleus ambiguus is involved primarily with supplying skeletal muscles via CNs IX, X, and XI. All the preganglionic fibers travel in the vagus nerve and its numerous branches (see Fig. 10-16, B). Some mingle with sympathetic fibers to form the extensive autonomic plexuses of the thoracic and abdominal cavities. The long preganglionic fibers are destined to synapse in small ganglia located within plexuses near the effector organ or ganglia within the wall of the organ itself. Some of the specific branches that conduct preganglionic parasympathetic fibers are the following: in the thorax-cardiac, pulmonary, and esophageal branches that join the plexuses of the same name; and in the abdomen-gastric and intestinal branches that join in the celiac plexus (and its subsidiary plexuses) en route to the stomach, small intestine, ascending colon and most of the transverse colon, accessory glands, and kidneys.
The vagus nerve has an extensive area of distribution as can be seen in Figure 10-16, B. However, note that the vagus nerve does not supply autonomic effectors of the head. These are innervated by CNs III, VII, and IX. Although vagal efferents are important, the afferent fibers conveying sensory information in the vagus nerve outnumber the efferent fibers (Williams et al., 1995).
Sacral Portion of the Parasympathetic Division.
Most effectors innervated by parasympathetic fibers are served by cranial nerves as can be noted from the previous discussion. The remaining effectors (e.g., the smooth muscle and glands of the pelvis) not innervated by the vagus nerve are innervated by the sacral portion of the craniosacral parasympathetic division. The origin of these preganglionic fibers is in the sacral autonomic nucleus of lamina VII of sacral cord segments two, three, and four (Fig. 10-17). The preganglionic fibers exit the cord in the ventral roots of these cord segments and leave the ventral rami as pelvic splanchnic nerves. These fibers course through the hypogastric plexuses, which are formed by both parasympathetic and sympathetic fibers. They synapse in ganglia within those plexuses or in ganglia within the wall of the effector organ. Generally, these fibers supply motor innervation to part of the transverse colon, descending colon, sigmoid colon, rectum, bladder, and reproductive organs. Also, some are vasodilatory to the penis and clitoris (erectile tissue), testes, ovaries, uterine tubes, and uterus. In addition, these parasympathetic fibers convey important sensory information (Williams et al., 1995; Kiernan, 1998) that provides reflex control of normal bladder, colon, and sexual organ function.
Enteric Nervous System
The third division of the ANS is the enteric nervous system, which was first recognized as such by Langley in 1921 (Gershon, 1981). In 1899 it was first acknowledged that motility of the GI system was under autonomous control by an intrinsic nervous system, when well-coordinated and purposeful motility still occurred independently after severing nerves to the GI system (Gershon, 1981). Since that time the concept that an intrinsic group of neurons exists in the wall of the gut, pancreas, and gall bladder has been fully accepted (Williams et al., 1995). This group of neurons extends from the esophagus to the rectum; it regulates GI vasomotor tone and motility and helps to regulate secretion and reabsorption. All of these activities are necessary for maintaining homeostasis. However, extrinsic postganglionic sympathetic fibers from prevertebral ganglia (splanchnic nerves) and preganglionic parasympathetic fibers via the vagus and pelvic splanchnic nerves provide input into these enteric neurons. This input can adjust, regulate, and (in some emergency situations) override this intrinsic system (Loewy, 1990a; Dodd and Role, 1991).
The enteric nervous system is found within the four layers of the wall of the GI tract and is considered to contain as many neurons as the spinal cord itself, approximately 100 million (Noback, Strominger, and Demarest, 1991; Camilleri, 1993; Kiernan, 1998). The enteric system consists of two major interconnected plexuses of neuron cell bodies (ganglia) and their processes (Fig. 10-18). One of these is the myenteric plexus of Auerbach, which is located between the inner circular and outer longitudinal smooth muscle layers of the muscularis externa. This plexus extends from the esophagus to the internal anal sphincter and regulates the motility of the GI tract. The second plexus is the submucosal plexus of Meissner, which is found in the submucosa of the GI tract. The submucosal plexus, which consists of deep and superficial plexuses, extends from the stomach to the internal anal sphincter and mediates the epithelial functions of secretion and absorption (Jänig, 1988; Taylor and Bywater, 1988; Loewy, 1990a; Williams et al., 1995). Nonganglionated nerve plexuses also are located in and supply various layers of the wall of the gut (Williams et al., 1995).
These plexuses are more than just large, extensive parasympathetic ganglia, as previously thought. A closer look at these areas reveals that they also receive input from sympathetic postganglionic fibers, as well as preganglionic parasympathetic fibers (Fig. 10-19). In addition, they consist of cell populations different from and more complex than other autonomic ganglia. Each plexus contains clusters of neurons that are interconnected, and each cluster is made up of a heterogeneous population of neurons (Loewy, 1990a; Camilleri, 1993; Kiernan, 1998). These neurons can be classified according to their morphology, electrical properties, chemical coding, and function (Williams et al., 1995; Furness, 2000; Hansen, 2003a). Based on size and branching patterns of the processes, seven types of neurons have been classified, although the majority are classified into three of those seven types. The neurons form multiple kinds of synapses, such as axosomatic, axoaxonal, and axodendritic. Evidence indicates that more than 30 neurotransmitters are involved in the synaptic neurotransmission. These are small molecules, peptides, or gases. The two most common chemicals are acetylcholine (ACh) and norepinephrine (NE) (Hansen, 2003a). Moreover it is suggested that one neuron may have as many as six different neuropeptides that function as neuromodulators. These modulate the release or action of the primary neurotransmitter or provide a trophic influence (Williams et al., 1995). Based on function, three types of neurons, which form complex circuits, have been classified. These are motor neurons, interneurons, and sensory neurons (see Fig. 10-19). Motor neurons are classified as one of three different types: muscle, secretomotor (some of which are vasodilators), and neurons supplying enteroendocrine cells. Muscle motor neurons innervate the smooth muscle of the GI system and are excitatory or inhibitory. The excitatory neurons use the neurotransmitters acetylcholine, substance P, and neurokinin A. The inhibitory neurons fire continuously, and thus their activity determines the amount of contraction that occurs. These neurons use numerous neurotransmitters such as nitric oxide, vasoactive intestinal polypeptide (VIP), adenosine triphosphate (ATP), gamma-aminobutyric acid (GABA), neuropeptide Y, and carbon monoxide.
A population of motor neurons also innervates enteroendocrine cells. These specialized cells are located in the mucosa and function to “taste” or detect the contents of the lumen of the gut. In response to specific stimuli (e.g., hyperosmolarity, mechanical distortion of the mucosa, bacterial products) in the lumen, they release chemical mediators, such as secretin, somatostatin, cholecystokinin (CCK), and 5 hydroxytryptamine (5-HT), that stimulate nearby afferent fibers that in turn synapse on inhibitory and excitatory neurons. The enteroendocrine cells also activate sensory neurons and extrinsic neurons (vagal and spinal afferents). The motor innervation to these cells is extensive, although the specific motor function to all enteroendocrine cells is unknown. However, research has shown that there is a direct cholinergic innervation to one population of cells, the enterochromaffin cells, and this innervation controls the release of 5-HT into the lumen of the gut (Hansen, 2003a).
The sensory neurons of the enteric nervous system have dendrites that project into the mucosal layer (near the lumen), where they may play a role in epithelial functions (e.g., secretion and absorption). This allows them to act as mechanoreceptors, thermoreceptors, and chemoreceptors (e.g., detecting pH and glucose concentration) (Jänig, 1988; Dodd and Role, 1991; Camilleri, 1993). Mechanoreceptors are stimulated by distention and reflexively cause tonic muscle contractions. Peristaltic contractions are produced if the distention is maintained. Chemoreceptors and mechanoreceptors also can be stimulated by nutrients such as glucose that are located in the lumen. This produces a reflex response by stimulating secretomotor fibers that results in secretion. This response is not only local but also can be widespread throughout the GI tract by means of the integrated circuitry of the plexuses that activates secretomotor neurons at different levels. The local reflex responses are mediated by neurons primarily using substance P and VIP. However, secondary neurotransmitters such as ATP, 5-HT, ACh, and VIP also are involved in the secretory process (Hansen, 2003a,b). The axonal process of the sensory neurons synapses with interneurons and motor neurons (to smooth muscle). The peristalsis reflex is an example of the interaction of these neurons. Stretching or chemical stimuli activate the sensory neurons that project to interneurons. The interneurons in turn synapse with orally directed excitatory motor neurons and anally directed inhibitory motor neurons (Williams et al., 1995), thus creating peristalsis.
The extrinsic afferent neurons are other sensory neurons located in the GI system. These neurons send input to the CNS about the GI environment with regard to normal physiologic processes and the feelings of discomfort and pain. They become the sensory arc for sympathetic and parasympathetic reflex responses. These afferents course in the vagus nerve, pelvic splanchnic nerves, and spinal afferent nerves, and encode activity within physiologic levels. However, the spinal afferents are also activated by pathophysiologic stimuli and mediate the sensation of pain. The peripheral receptors of the spinal afferents are found in the mucosal layers, muscle, serosal membrane, and mesentery (Grundy, 2002). Spinal afferent fibers use calcitonin gene-related peptide (CGRP) and substance P as their neurotransmitters. These are known to be chemical mediators involved in the neurogenic inflammatory process and GI inflammation. Two types of vagal afferent endings are mechanosensory. One type is associated with branches that are interspersed among the circular and longitudinal muscle cell layers. These have been called “in series tension receptor endings,” and they respond to muscle tension. The second type consists of endings that surround the myenteric ganglia. These monitor the stress and strains produced by muscle stretch or contraction. Also, there is evidence to show that some terminal endings of gut afferent fibers function as “efferent” sensorimotor fibers. These have collateral branches coursing to blood vessels and to the enteric ganglia where blood flow and reflexes can be modulated. Studies also indicate that some afferent fibers supplying the mucosa and coursing in the vagus nerve are sensitive to specific mediators released from the mucosal enteroendocrine cells in response to luminal nutrients. These chemical mediators are thought to initiate the neurotransmission events originating in the gut. This process is called “nutrient tasting” (Raybould, 1999; Grundy, 2002). One of these mediators is CCK, which is secreted in response to the presence of fats and protein in the intestine. In response to CCK stimulation, vagal afferent fibers become the sensory reflex limb for reflex responses controlling GI motility, secretion, and the regulation of food intake. Serotonin, in response to bacterial toxins, also functions as a mediator that stimulates vagal afferent fibers. The resulting reflex response stimulates motility and secretion and functions to protect the gut by removing the potentially harmful agent (Grundy, 2002).
The enteric sensory neurons also appear to have a broader function that involves the sympathetic system. These neurons are involved with reflexes mediated by postganglionic sympathetic fibers. Postganglionic sympathetic neurons project to numerous effectors in the GI system, including blood vessels, smooth muscle of the sphincters, myenteric plexus (concerning motility), submucosal plexus (regulating secretion and absorption), and even the organized lymphatic tissue of the GI wall (Jänig, 1988). The axons of the sensory (enteric) afferent neurons project from the myenteric plexus to sympathetic prevertebral ganglia (see Fig. 10-19). Animal studies of the prevertebral celiac ganglion show that three populations of noradrenergic neurons innervate the gut. One population, which is also immunoreactive for neuropeptide Y, functions as vasoconstrictors and projects to the spleen, mesenteric blood vessels and arterioles in the gut wall. A second population, which is immunoreactive for somatostatin, inhibits secretomotor neurons. This population terminates in the submucosal ganglia of the small intestine, cecum, and proximal colon. The third population inhibits motility and projects to the myenteric ganglia. Enteric sensory neurons project to the two populations of neurons that inhibit secretion and inhibit motility but not to the vasomotor neurons (Furness, 2003
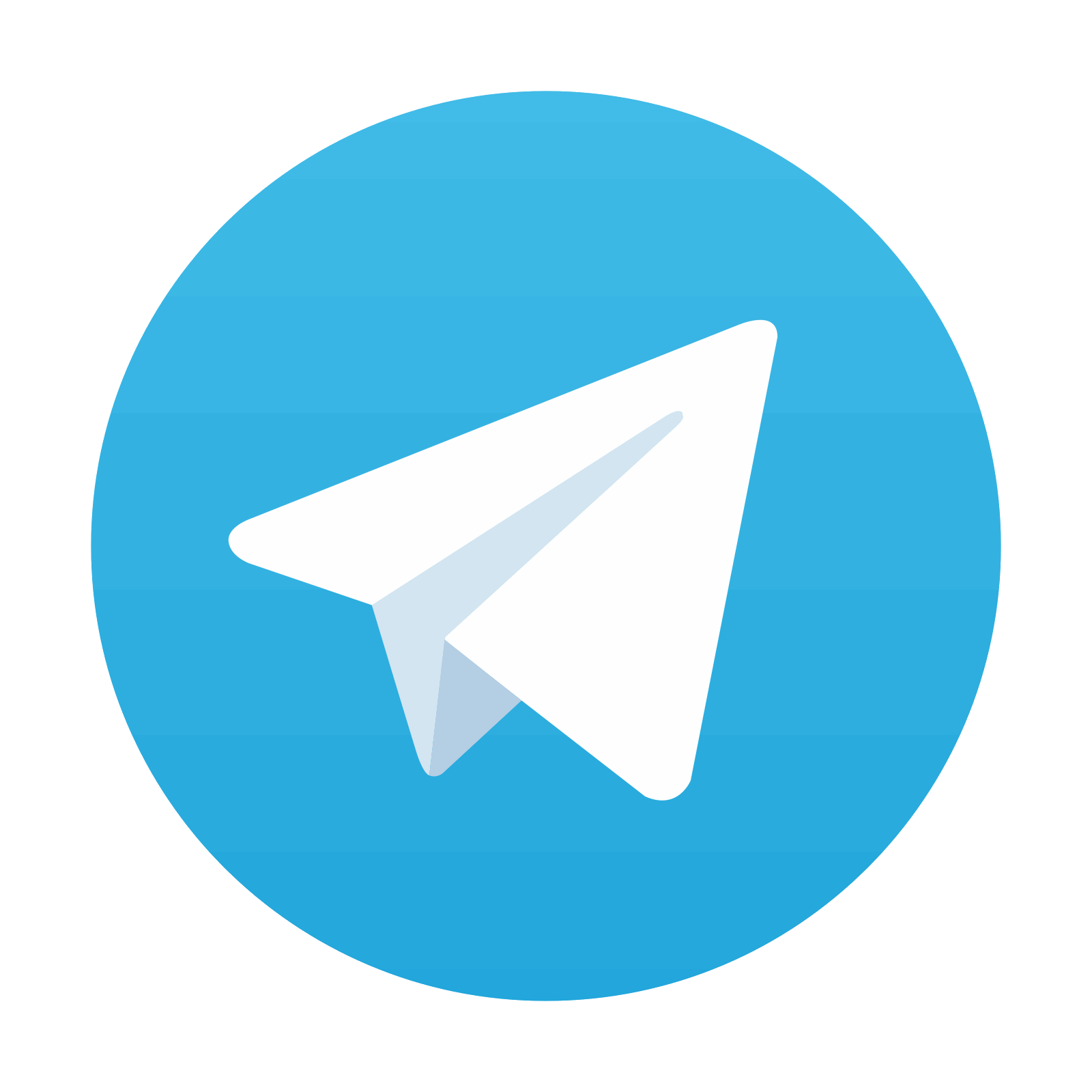
Stay updated, free articles. Join our Telegram channel

Full access? Get Clinical Tree
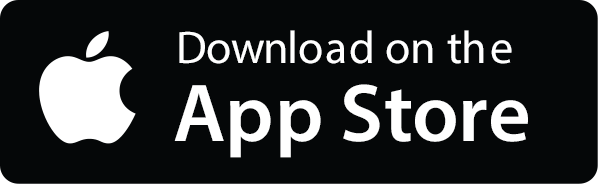
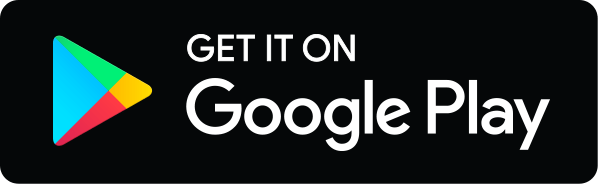