Fig. 21.1
Schematic illustrating the biomedical applications of MNPs
21.2 Separation of Biological Samples
MNPs functionalized with biologically specific components such as antibodies offer a unique opportunity to control and extract biological samples from a mixture. For the separation of large biological samples such as cells (10–100 μm), magnetic microparticles (above 1 μm) can be used. However, to get close contact with biological entities with smaller size, such as virus (20–450 nm), proteins (5–50 nm), or genes (2 nm wide and 10–100 nm long), materials in the nanodimension (i.e., NPs) are preferred. Moreover, the high surface-to-volume ratio of NPs compared to microparticles allows a higher ratio of labeling with less nonspecific binding [14]. Driven by these benefits, MNPs have been utilized to isolate and purify various different biological entities such as proteins, bacteria, and cells.
One of the earliest applications is the protein separation. In proteomics, the purification of native and recombinant proteins is always a time-consuming task. The job is usually performed using variety of chromatography, electrophoresis, ultrafiltration, precipitation, etc. Among them, affinity chromatography is one of the favorite techniques. However, this technique is unable to cope with samples containing particulate materials, so it is not suitable for early stages of the purification process where suspended solid and fouling components are presented in the sample [15]. Therefore, magnetic separation is attractive because of simple handling, low cost, and high efficiency for crude samples. For example, FePt MNPs functionalized with Ni(II)-chelated nitrilotriacetic acid (NTA-Ni2+) showed tight binding to the 6-histidine-tagged proteins, through the 6 coordination sites of the nickel ion. The 6-histidine-tagged protein was easily and rapidly separated from the cell lysate without any pretreatment. Following analysis revealed a high binding capacity of 2–3 mg proteins/1 mg of MNPs, which was about 200 times higher than that of commercial magnetic microbeads [16–18].
Bacteria separation is another exciting application, which was first demonstrated by Gu et al. [19]. Specifically, FePt MNPs were conjugated with vancomycin (Van), which bound to the terminal D-Ala-D-Ala dipeptide of bacterial cell wall precursors. By mixing Van-FePt MNPs with a solution containing Van-sensitive bacteria for 10 min, bacteria–MNPs conjugates could be separated by magnet. The detection limit was 8 cfu/mL for S. aureus, 10 cfu/mL for S. epidermidis and 4 cfu/mL for coagulase-negative staphylococci, which was comparable to the standard but time-consuming polymerase chain reaction (PCR) assay [19].
The last but not least one is cell capture and isolation such as the isolation of circulating tumor cells (CTCs). CTCs are cancer cells which slough off cancerous tissue and move through the bloodstream to a new site. Thus, they are considered as seeds for metastasis [20]. The presence of CTCs in the peripheral blood has been shown to be associated with decreased progression-free survival and decreased overall survival in patients treated for metastatic breast, colorectal, or prostate cancer. And quantification of CTCs can be used for both the prognosis of cancer metastasis and the reliable surrogate marker of treatment response. One method for the CTC isolation and quantification is the CellSearch® system that can specifically and efficiently separate CTCs from other types of cells in blood samples [21]. Typically, blood sample is firstly placed in a tube and centrifuged to removal solid blood from plasma. Then, MNPs conjugated with CTC-specific antibodies (i.e., anti epithelial cell adhesion molecule antibody) are mixed with blood samples for a few minutes before being magnetically separated and washed with buffers. The isolated cells are further stained with cytokeratin monoclonal antibodies (stain CTCs), CD45 monoclonal antibodies (stained leukocytes which may contaminate the sample), and DAPI (stain nuclei of both CTCs and leukocytes). Finally, CTCs are identified with positive signals for both cytokeratin and DAPI [21].
21.3 Diagnosis
Early, accurate, and in-time diagnoses of diseases are critical to prevent the deterioration, to identify the effective and efficient treatment, to evaluate the efficacy of treatment, to improve the quality of patient life, and to reduce the cost of treatment. With functionalized MNPs, disease progression can be examined by using ex vivo bioassays (colorimetric immunoassay and magnetic immunoassay) and/or in vivo imaging like magnetic resonance imaging (MRI).
21.3.1 Magnetic Immunoassay
Magnetic immunoassay (MIA) is a novel type of diagnostic assay, which utilizes MNPs as labels instead of conventional radioisotopes (radioimmunoassay), fluorescent dyes (fluorescent immunoassay), substrates and enzymes (enzyme-linked immunosorbent assay, ELISA). MNPs are usually conjugated with an antigen or antibody for the recognition of interested molecules. The binding between MNPs and interested molecules causes the clustering of MNPs, which results in an increase of magnetic moment for the detection [22, 23]. For example, Lee et al. [23] developed a handheld diagnostic magnetic resonance (DMR) system, which consisted of planar microcoils, microfluidic channels, and a portable magnet. It used T2 relaxation time as detection signal, and could be performed in turbid samples (e.g., blood, urine, and sputum) with few or no preparation steps. In a recent report, they determined the accuracy of the DMR system by comparing its performance to a large benchtop NMR relaxometer. In addition to the smaller sample volume required (~5 μL for DMR vs. 300 μL for NMR relaxometer), the DMR system showed a mass detection limit improvement of two orders of magnitude (1 ng vs. 80 ng for avidin detection). This technology could also be extended for the detection of bacteria, cancer cells, and protein biomarkers with a high mass detection sensitivity (more than 800-fold improvement relative to a benchtop relaxometer). The sensitivity could be further improved by preparing new classes of water-soluble MNPs with higher magnetization [23].
21.3.2 Colorimetric Immunoassay
Colorimetric immunoassay quantifies the analytes through absorbance generated from a color reaction between substrates and enzymes. One of the most widely used enzymes is peroxidase, which catalyzes the oxidation of organic substrates and produces a color change for detection. Peroxidase enzymes and their mimics contain Fe2+ and Fe3+ in the reaction centers, which is essential for the catalytic activity. Although MNPs like Fe3O4 NPs have been conjugated with horseradish peroxidase (HRP) to introduce peroxidase activity in a number of applications including commercially available magnetic ELISA kits, their peroxidase-like activity has been ignored for a long time. Until recently, Fe3O4 NPs have been reported to possess intrinsic peroxidase-like activity. In the presence of H2O2, all different sizes (30, 150, and 300 nm) of Fe3O4 MNPs catalyzed the reaction and produced a blue color for substrate 3,3,5,5-tetramethylbenzidine (TMB), a brown color for diazoaminobenzene (DAB), and an orange color for o-phenylenediamine (OPD) [24]. If AH represents the substrate, which is a hydrogen donor, the mechanism of the catalytic reaction could be written as follow:


(21.1)
Through a series of studies, the peroxidase-like activity of Fe3O4 MNPs was found to be H2O2, pH, and temperature dependent. Meanwhile, the reaction followed Michaelis–Menten kinetics. Two formats of immunoassay based on this concept have been performed to detect hepatitis B virus surface antigen and cardiac troponin I (a biomarker for myocardial infarction) [24].
More recently, the peroxidase-like activity of Fe3O4 MNPs was used for targeting and visualizing tumor tissues. Magnetoferritin (M-HFn) NPs were prepared by encapsulating iron oxide NPs inside a HFn shell. The schematic process is shown in Fig. 21.2a. TEM images (Fig. 21.2b–d) are shown a core–shell structure with a 4.7-nm core of iron oxide (Fig. 21.2e). The color reactions are shown in Fig. 21.2f, g. The HFn protein shell could specifically bind to tumor cells that overexpressed transferring receptor 1 (TfR1). These M-HFn NPs allowed to the visualization of the tumor tissue through the peroxidase-like activity of iron oxide core without any additional recognition ligands on their surface. This strategy simplified the modification process of conventional NPs and prevented nonspecific binding induced by an excess of ligands on the NP surface; 474 clinical specimens from patients with nine types of cancers were examined. They confirmed that M-HFn NPs could distinguish cancerous cells from normal cells with a sensitivity (positive/cases) of 98 % and a specificity (negative/cases) of 95 % [25].
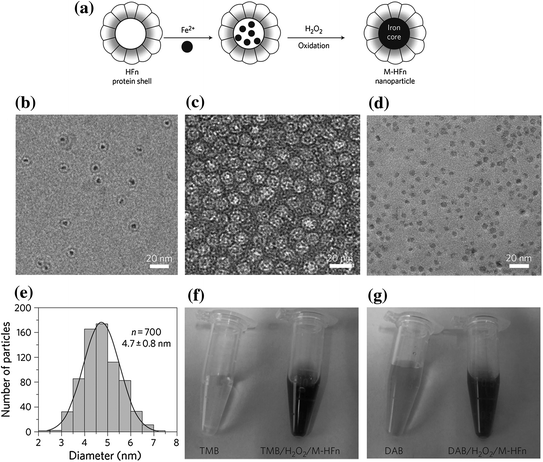
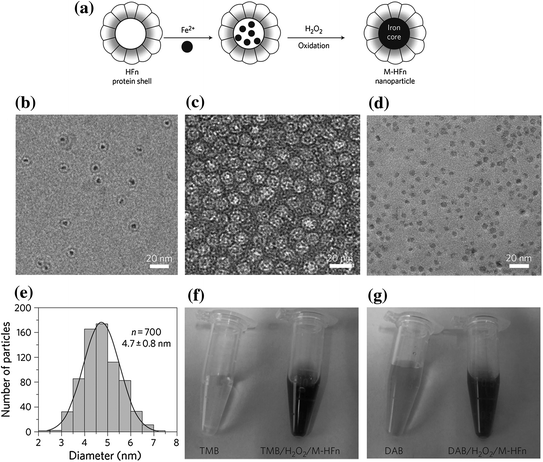
Fig. 21.2
Preparation and characterization of M-HFn NPs. a Schematic showing the preparation of M-HFn NPs and their structure. b CryoTEM image of M-HFn NPs. c, d TEM images of protein shells (c) and iron oxide cores (d). HFn protein shells were negatively stained with uranyl acetate for TEM observations, and iron oxide cores in HFn were unstained. e Size distribution of iron oxide cores, with a median diameter of 4.7 ± 0.8 nm. f, g Characterization of peroxidase activity of M-HFn NPs. M-HFn catalyzed the oxidation of peroxidase substrates TMB (f) and DAB (g) in the presence of H2O2 to give a colored product. Recreated with kind permission of (© 2012 Macmillan Publishers Limited) [25]
21.3.3 Magnetic Resonance Imaging
MRI is a noninvasive imaging modality that has been widely used in clinical diagnosis [26]. This technique is based on the property that the magnetization of hydrogen protons in human body will be aligned around an applied external magnetic field. The presence of MNPs shortens the spin–spin relaxation time and thus produces a negative (decreased) signal in T2– and T2*-weighted MR images [27]. Therefore, by labeling the interested cells/tissues with MNPs, we can visualize them noninvasively. So far, MNPs have been applied as MRI contrast agents to improve the sensitivity of detection and diagnosis of major diseases including cancers, cardiovascular diseases, and diabetes which in total account for nearly 2 of every 3 deaths in the USA—close to 1.5 million people in the year 2001.
In cancer prognosis, the status of lymph node is an independent adverse prognostic factor. The enlargement of lymph node usually indicates the potential malignancy or metastasis [28]. Given that intravenously or subcutaneously injected MNPs would be taken up by lymph nodes by means of interstitial-lymphatic fluid transport, we can label lymph nodes with MNPs for cancer diagnosis with MRI. In 2003, 80 patients with presurgical clinical stages T1, T2, or T3 prostate cancer were examined by MRI before and 24 h after the intravenous administration of MNPs. The imaging results were correlated with histological findings; 71.4 % of nodes did not fulfill the usual imaging criteria for malignancy. MNPs have effectively identified small (with a diameter of 5–10 mm) and otherwise undetectable lymph node metastases in patients with prostate cancer [28].
Besides cancer, MNPs are also used in the diagnosis of the cardiovascular diseases such as myocardial injury, atherosclerosis, and vascular disease [29]. The wash-in kinetics of MNPs, which are normally confined to the intravascular space, can be used to demarcate the area of myocardium at risk. The hyperacute stage of myocardial injury increases the capillary permeability and hyperemia, which finally enhances the contrast of the injured areas [30]. To prevent the wash-out of MNPs from acutely injured myocardium, an active ligand binding which achieves specific targeted imaging is also highly promising [31]. Atherosclerosis is another cardiovascular condition in which an artery wall thickens as a result of the accumulation of fatty materials and formation of multiple plaques within the arteries. Long-circulating MNPs are able to penetrate an atherosclerotic plaque and then taken up by the macrophage [32]. Thus, plaque macrophage content could be gauged by MRI for atherosclerosis diagnosis. In addition, MNPs labeled with linear peptides (screened by phage display) can specifically bind to the vascular adhesion molecule-1 (VCAM-1), which is expressed on vascular endothelium. These VCAM-1-targeted MNPs have been demonstrated as sensitive contrast agents for detection of VCAM-1 expression in the aortic roots of statin-treated mice [33].
Diabetes is another major health and development challenge of the twenty-first century. Worldwide, there are already more than 360 million people with diabetes and another 280 million at identifiably high risk of developing diabetes. One major type of diabetes is type 1 diabetes (3–5 % of diabetes globally) is an autoimmune disease that destroys the insulin-producing cells (beta cells) of the pancreas. The initial immune infiltration, termed insulitis, starts many years before the development of type 1 diabetes (T1D) and progresses slowly until a critical mass of beta cells has been annihilated. In the progress of immune infiltration, lymphocytes migrate to the pancreas and destroy beta cells. Thus, pancreatic biopsy is one approach to diagnose insulitis but has been resisted by patients due to its invasiveness. A more attractive alternative to biopsy is provided by noninvasive MRI imaging, in which microvascular leakage was taken as an indicator of inflammation [34]. Specifically, Denis and colleagues developed long-circulating magnetofluorescent NPs with half-lives of over 10 h in blood vessel for both fluorescent and MR imaging, which could follow the microvascular changes accompanying inflammation. Results from mouse showed a positive correlation in magnetofluorescent NPs accumulation in the pancreas and insulitis aggressivity [34]. With this technology, the same group could predict the onset of diabetes (from 6 to 10 weeks) in nonobese diabetic (NOD) mice [35]. In general, MNPs-based imaging holds potential as a useful tool for performing long-term, longitudinal studies of the progression of diabetes in humans.
21.4 Therapeutics
21.4.1 MNPs as Carriers for Drugs and Genes
Targeted drug delivery using MNPs was first proposed in the late 1970s and has been one of the most desirable applications of MNPs for chemotherapy [14]. Following the early studies of Widder and Senyi, the efficacy of this approach was demonstrated in numerous small animal studies and even resulted in a small number of clinical trials. However, despite these efforts and achievements, this technique has yet to develop into a workable clinical application.
One of the reasons is the low payload capacity of existing MNPs because payload (i.e., drugs) can only be attached on the surface or embedded in the double-layer coating around MNPs. To address this issue, a novel platform of engineered Fe3O4 porous hollow NPs (HMNPs) was designed for the controlled release of cisplatin. Specifically, cisplatin was encapsulated in the interior cavities, and the targeting agent, Herceptin, was attached on the surface of MNPs. These NPs could then efficiently target and deliver cisplatin to ErbB2-/Neu-positive breast cancer cells (SK-BR-3). The percentage of loaded cisplatin was improved from 4.82 % of Fe3O4 MNPs to 24.8 % of Fe3O4 HMNPs. Meanwhile, the pores were subject to acid etching in low pH environment and subsequently facilitated cisplatin release. The amount of cisplatin released when pH < 6 was more than 3 times compared to a release in neutral physiological conditions. Once HMNPs were internalized to the endosomes/lysosomes, pH-sensitive pores were further opened up to accelerate the cisplatin release and finally kill the cancer cells [36].
Besides the effort of improving the drug loading efficiency, another focus is to integrate drug delivery and molecular imaging into the same system. For example, dumbbell-shaped Au-Fe3O4 NPs were synthesized (Fig. 21.3a, b) and conjugated with cisplatin complexes to serve as a multifunctional platform for targeted cisplatin delivery (Fe3O4 part) and optical imaging (Au part) [37, 38]. Herceptin was immobilized onto Fe3O4 through PEG3000-CONH-Herceptin to offer specific SK-BR-3 cell targeting ability. While cisplatin complexes were attached onto Au side by reacting with Au-S-CH2CH2N(CH2COOH)2. Reflection images of SK-BR-3 and MCF-7 cells after incubation with the same concentration of cisplatin-Au-Fe3O4-Herceptin NPs (Fig. 21.3d, e) showed the specificity and efficacy of these NPs. Au-Fe3O4 NPs did not inhibit cell growth at tested Fe concentration. However, once coupled with cisplatin, these NPs showed remarkable cytotoxicity with a low half-maximal inhibitory concentration (IC50) toward SK-BR-3 cells of 1.76 μg/mL of Pt (Fig. 21.3f) compared to free cisplatin needed (3.5 μg/mL of Pt). This methodology was expected to have a great potential to function as nanovehicles for highly sensitive diagnostic and highly efficient therapeutic applications [38].
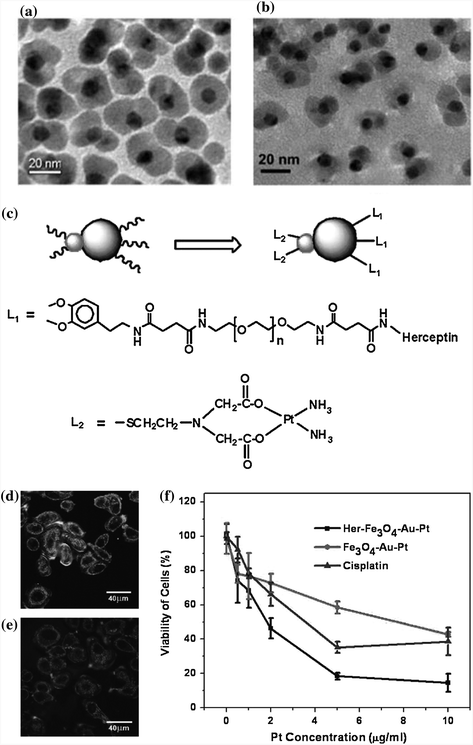
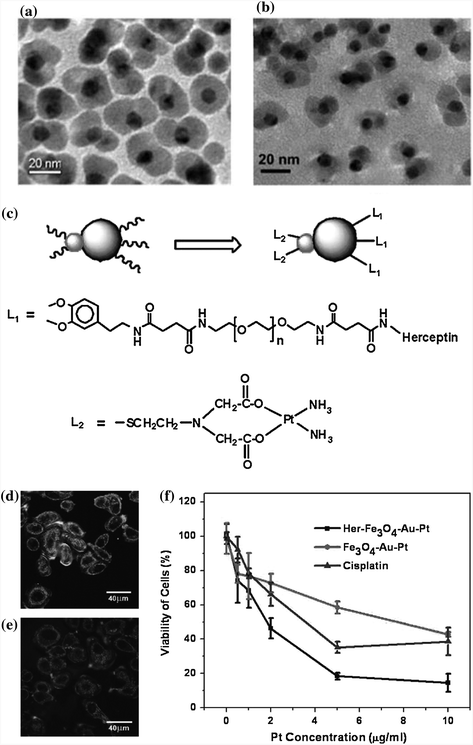
Fig. 21.3
a, b TEM images of the 8–20 nm dumbbell-like Au-Fe3O4 NPs before (a) and after (b) surface modification. c Schematic illustration of dumbbell-like NPs coupled with Herceptin and a cisplatin complex for target-specific cisplatin delivery. d, e Reflection images of SK-BR-3 (d) and MCF-7 cells (e) after incubation with the same concentration of cisplatin-Au-Fe3O4-Herceptin NPs. f Viability of Sk-Br3 cells after incubation with platin-Au-Fe3O4 NPs, platin-Au-Fe3O4-Herceptin NPs, and free cisplatin. Recreated with kind permission of (© 2008 Wiley-VCH Verlag GmbH and Co. KGaA, Weinheim), [37] and (© 2009 American Chemical Society) [38]
Finally, MNPs could also deliver peptide/protein, oligonucleotide, plasmid, etc. One example is magnetofection which uses magnetic field to concentrate MNPs containing nucleic acids into cells. MNPs can be incorporated into viral or nonviral platforms to facilitate gene delivery. Recently, recombinant adeno-associated virus 2 (rAAV) was conjugated onto MNPs to achieve therapeutic levels of transgene expression. This platform enhanced the transfection efficacy. The same level of transfection seen with free vector can be achieved using 1 % of vector conjugated MNPs [39]. For nonviral deliver systems, MNPs can be coated with polyethyleneimine (PEI) or polyethylene glycol-grafted PEI (to reduce cytotoxicity of PEI), which allows the efficient loading and protection of genes and holds a great potential for advanced gene delivery and therapy [40].
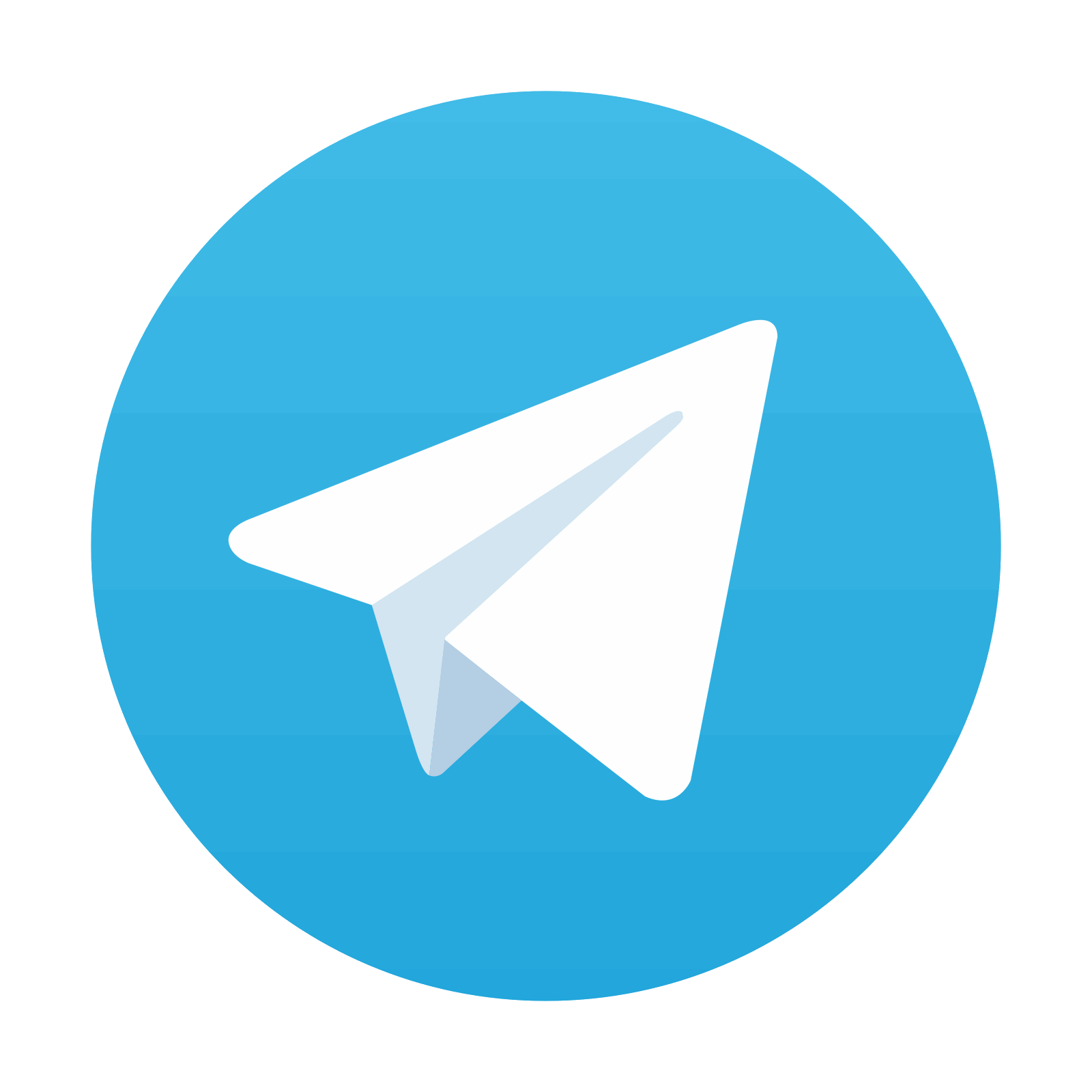
Stay updated, free articles. Join our Telegram channel

Full access? Get Clinical Tree
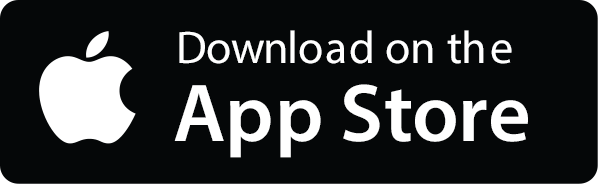
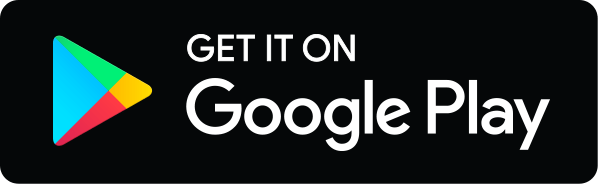