OBJECTIVES
After studying this chapter, you should be able to:
Describe the various forms of memory.
Identify the parts of the brain involved in memory processing and storage.
Define synaptic plasticity, long-term potentiation (LTP), long-term depression (LTD), habituation, and sensitization, and their roles in learning and memory.
Describe the abnormalities of brain structure and function found in Alzheimer disease.
Define the terms categorical hemisphere and representational hemisphere and summarize the difference between these hemispheres.
Summarize the differences between fluent and nonfluent aphasia, and explain each type on the basis of its pathophysiology.
INTRODUCTION
The understanding of brain function in humans has been revolutionized by the development and widespread availability of positron emission tomographic (PET), functional magnetic resonance imaging (fMRI), computed tomography (CT) scanning, and other imaging and diagnostic techniques. PET is often used to measure local glucose metabolism, which is proportional to neural activity, and fMRI is used to measure local amounts of oxygenated blood. These techniques provide an index of the level of the activity in various parts of the brain in completely intact healthy humans and in those with different diseases or brain injuries (see Clinical Box 15–1). They have been used to study not only simple responses but complex aspects of learning, memory, and perception. Different portions of the cortex are activated when hearing, seeing, speaking, or generating words. Figure 15–1 shows examples of the use of imaging to compare the functions of the cerebral cortex in processing words in a male versus a female subject.
FIGURE 15–1
Comparison of the images of the active areas of the brain in a man (left) and a woman (right) during a language-based activity. Women use both sides of their brain whereas men use only a single side. This difference may reflect different strategies used for language processing. (Used with permission of Shaywitz et al, 1995. NMR Research/Yale Medical School.)
Other techniques that have provided information on cortical function include stimulation of the exposed cerebral cortex in conscious humans undergoing neurosurgical procedures and, in a few instances, studies with chronically implanted electrodes. Valuable information has also been obtained from investigations in laboratory primates. However, in addition to the difficulties in communicating with them, the brain of the rhesus monkey is only one-fourth the size of the brain of the chimpanzee, our nearest primate relative; and the chimpanzee brain is in turn one-fourth the size of the human brain.
LEARNING & MEMORY
A characteristic of animals and particularly of humans is their ability to alter behavior on the basis of experience. Learning is acquisition of the information that makes this possible and memory is the retention and storage of that information. The two are obviously closely related and are considered together in this chapter.
From a physiologic point of view, memory is divided into explicit and implicit forms (Figure 15–2). Explicit or declarative memory is associated with consciousness, or at least awareness, and is dependent on the hippocampus and other parts of the medial temporal lobes of the brain for its retention. Clinical Box 15–2 describes how tracking a patient with brain damage has led to an awareness of the role of the temporal lobe in declarative memory. Implicit or nondeclarative memory does not involve awareness, and its retention does not usually involve processing in the hippocampus.
FIGURE 15–2
Forms of memory. Explicit (declarative) memory is associated with consciousness and is dependent on the integrity of the hippocampus, temporal lobes, neocortex, and prefrontal cortex for its retention. Explicit memory is for factual knowledge about people, places, things, and events. Implicit (nondeclarative) memory does not involve awareness, and it does not involve processing in the hippocampus. It requires the integrity of the amygdala, cerebellum, striatum, and neocortex as well as reflex pathways. Implicit memory is important for training reflexive motor or perceptual skills, classical conditioning, and habituation and sensitization. (Modified with permission from Kandel ER, Schwartz JH, Jessell TM [editors]: Principles of Neural Science, 4th ed. New York, NY: McGraw-Hill; 2000.)
CLINICAL BOX 15–1 Traumatic Brain Injury
Traumatic brain injury (TBI) is defined as a nondegenerative, noncongenital insult to the brain due to an excessive mechanical force or penetrating injury to the head. It can lead to a permanent or temporary impairment of cognitive, physical, emotional, and behavioral functions, and it can be associated with a diminished or altered state of consciousness. TBI is one of the leading causes of death or disability worldwide. According to the Centers for Disease Control and Prevention, each year at least 1.5 million individuals in the United States sustain a TBI. It is most common in children under age 4, in adolescents aged 15–19 years of age, and in adults over the age of 65. In all age groups, TBI occurs more often in males than in females (2:1). In about 75% of the cases, the TBI is considered mild and manifests as a concussion. Adults with severe TBI who are treated have a mortality rate of about 30%, but about 50% regain most if not all of their functions with therapy. The leading causes of TBI include falls, motor vehicle accidents, being struck by an object, and assaults. In some cases, areas remote from the actual injury also begin to malfunction, a process called diaschisis. TBI is often divided into primary and secondary stages. Primary injury is that caused by the mechanical force (eg, skull fracture and surface contusions) or acceleration–deceleration due to unrestricted movement of the head leading to shear, tensile, and compressive strains. These injuries can cause intracranial hematoma (epidural, subdural, or subarachnoid) and diffuse axonal injury. Secondary injury is often a delayed response and may be due to impaired cerebral blood flow that can eventually lead to cell death. A Glasgow Coma Scale is the most common system used to define the severity of TBI and evaluates motor responses, verbal responses, and eye opening to assess the levels of consciousness and neurologic functioning after an injury. Symptoms of mild TBI include headache, confusion, dizziness, blurred vision, ringing in the ears, a bad taste in the mouth, fatigue, disturbances in sleep, mood changes, and problems with memory, concentration, or thinking. Individuals with moderate or severe TBI show these symptoms as well as vomiting or nausea, convulsions or seizures, an inability to be roused, fixed and dilated pupils, slurred speech, limb weakness, loss of coordination, and increased confusion, restlessness, or agitation. In the most severe cases of TBI, the affected individual may go into a permanent vegetative state.
THERAPEUTIC HIGHLIGHTSThe advancements in brain imaging technology have improved the ability of medical personnel to diagnose and evaluate the extent of brain damage. Since little can be done to reverse the brain damage, therapy is initially directed at stabilizing the patient and trying to prevent further (secondary) injury. Medications that can be administered include diuretics (to reduce pressure in the brain), anticonvulsant drugs during the first week post injury (to avoid additional brain damage resulting from a seizure), and coma-inducing drugs (to reduce oxygen demands). This is followed by rehabilitation that includes physical, occupational, and speech/language therapies. Recovery of brain function can be due to several factors: brain regions that were suppressed but not damaged can regain their function, axonal sprouting and redundancy allows other areas of the brain to take over the functions that were lost due to the injury, and behavioral substitution, by learning new strategies to compensate for the deficits.
Explicit memory is for factual knowledge about people, places, and things. It is divided into semantic memory for facts (eg, words, rules, and language) and episodic memory for events. Explicit memories that are initially required for activities such as riding a bicycle can become implicit once the task is thoroughly learned.
Implicit memory is important for training reflexive motor or perceptual skills and is subdivided into four types. Priming is the facilitation of the recognition of words or objects by prior exposure to them and is dependent on the neocortex. An example of priming is the improved recall of a word when presented with the first few letters of it. Procedural memory includes skills and habits, which, once acquired, become unconscious and automatic. This type of memory is processed in the striatum. Associative learning relates to classical and operant conditioning in which one learns about the relationship between one stimulus and another. This type of memory is dependent on the amygdala for its emotional responses and the cerebellum for the motor responses. Nonassociative learning includes habituation and sensitization and is dependent on various reflex pathways.
CLINICAL BOX 15–2 The Case of HM: Defining a Link between Brain Function & Memory
HM was a patient who suffered from bilateral temporal lobe seizures that began following a bicycle accident at age 9. His case has been studied by many scientists and has led to a greater understanding of the link between the temporal lobe and declarative memory. HM had partial seizures for many years, and then several tonic-clonic seizures by age 16. In 1953, at the age of 27, HM underwent bilateral surgical removal of the amygdala, large portions of the hippocampal formation, and portions of the association area of the temporal cortex. HM’s seizures were better controlled after surgery, but removal of the temporal lobes led to devastating memory deficits. He maintained long-term memory for events that occurred prior to surgery, but he suffered from anterograde amnesia. His short-term memory was intact, but he could not commit new events to long-term memory. He had normal procedural memory, and he could learn new puzzles and motor tasks. His case was the first to bring attention to the critical role of temporal lobes in formation of long-term declarative memories and to implicate this region in the conversion of short-term to long-term memories. Later work showed that the hippocampus is the primary structure within the temporal lobe involved in this conversion. Because HM retained memories from before surgery, his case also shows that the hippocampus is not involved in the storage of declarative memory. HM died in 2008 and only at that time was his identity released; his full name was Henry Gustav Molaison. An audio-recording by National Public Radio from the 1990s of HM talking to scientists was released in 2007 and is available at http://www.npr.org/templates/story/story.php?storyId=7584970.
Explicit memory and many forms of implicit memory involve (1) short-term memory, which lasts seconds to hours, during which processing in the hippocampus and elsewhere lays down long-term changes in synaptic strength; and (2) long-term memory, which stores memories for years and sometimes for life. During short-term memory, the memory traces are subject to disruption by trauma and various drugs, whereas long-term memory traces are remarkably resistant to disruption. Working memory is a form of short-term memory that keeps information available, usually for very short periods, while the individual plans action based on it.
The key to memory is alteration in the strength of selected synaptic connections. Second messenger systems contribute to the changes in neural circuitry required for learning and memory. Alterations in cellular membrane channels are often correlated to learning and memory. In all but the simplest of cases, the alteration involves the synthesis of proteins and the activation of genes. This occurs during the change from short-term working memory to long-term memory.
In animals, acquisition of long-term learned responses is prevented if, within 5 min after each training session, the animals are anesthetized; given electroshock; subjected to hypothermia; or given drugs, antibodies, or oligonucleotides that block the synthesis of proteins. If these interventions are performed 4 h after the training sessions, there is no effect on acquisition. The human counterpart of this phenomenon is the loss of memory for the events immediately preceding a brain concussion or electroshock therapy (retrograde amnesia). This amnesia encompasses longer periods than it does in experimental animals (sometimes many days) but remote memories remain intact.
Short- and long-term changes in synaptic function can occur as a result of the history of discharge at a synapse; that is, synaptic conduction can be strengthened or weakened on the basis of past experience. These changes are of great interest because they represent forms of learning and memory. They can be presynaptic or postsynaptic in location.
One form of plastic change is posttetanic potentiation, the production of enhanced postsynaptic potentials in response to stimulation. This enhancement lasts up to 60 s and occurs after a brief tetanizing train of stimuli in the presynaptic neuron. The tetanizing stimulation causes Ca2+ to accumulate in the presynaptic neuron to such a degree that the intracellular binding sites that keep cytoplasmic Ca2+ low are overwhelmed.
Habituation is a simple form of learning in which a neutral stimulus is repeated many times. The first time it is applied it is novel and evokes a reaction (the orienting reflex or “what is it?” response). However, it evokes less and less electrical response as it is repeated. Eventually, the subject becomes habituated to the stimulus and ignores it. This is associated with decreased release of neurotransmitter from the presynaptic terminal because of decreased intracellular Ca2+. The decrease in intracellular Ca2+ is due to a gradual inactivation of Ca2+ channels. It can be short term, or it can be prolonged if exposure to the benign stimulus is repeated many times. Habituation is a classic example of nonassociative learning.
Sensitization is in a sense the opposite of habituation. Sensitization is the prolonged occurrence of augmented postsynaptic responses after a stimulus to which one has become habituated is paired once or several times with a noxious stimulus. At least in the sea snail Aplysia, the noxious stimulus causes discharge of serotonergic neurons that end on the presynaptic endings of sensory neurons. Thus, sensitization is due to presynaptic facilitation. Sensitization may occur as a transient response, or if it is reinforced by additional pairings of the noxious stimulus and the initial stimulus, it can exhibit features of short-term or long-term memory. The short-term prolongation of sensitization is due to a Ca2+-mediated change in adenylyl cyclase that leads to a greater production of cAMP. The long-term potentiation (LTP) also involves protein synthesis and growth of the presynaptic and postsynaptic neurons and their connections.
LTP is a rapidly developing persistent enhancement of the postsynaptic potential response to presynaptic stimulation after a brief period of rapidly repeated stimulation of the presynaptic neuron. It resembles posttetanic potentiation but is much more prolonged and can last for days. There are multiple mechanisms by which LTP can occur, some are dependent on changes in the N-methyl-D-aspartate (NMDA) receptor and some are independent of the NMDA receptor. LTP is initiated by an increase in intracellular Ca2+ in either the presynaptic or postsynaptic neuron.
LTP occurs in many parts of the nervous system but has been studied in greatest detail in a synapse within the hippocampus, specifically the connection of a pyramidal cell in the CA3 region and a pyramidal cell in the CA1 region via the Schaffer collateral. This is an example of an NMDA receptor-dependent form of LTP involving an increase in Ca2+ in the postsynaptic neuron. Recall that NMDA receptors are permeable to Ca2+ as well as to Na+ and K+. The hypothetical basis of the Schaffer collateral LTP is summarized in Figure 15–3. At the resting membrane potential, glutamate release from a presynaptic neuron binds to both NMDA and non-NMDA receptors on the postsynaptic neuron. In the case of the Schaffer collateral the non-NMDA receptor of interest is the α-amino-3-hydroxy-5-methylisoxazole-4 propionic acid (AMPA) receptor. Na+ and K– can flow only through the AMPA receptor because the presence of Mg2+ on the NMDA receptor blocks it. However, the membrane depolarization that occurs in response to high frequency tetanic stimulation of the presynaptic neuron is sufficient to expel the Mg2+ from the NMDA receptor, allowing the influx of Ca2+ into the postsynaptic neuron. This leads to activation of Ca2+/calmodulin kinase, protein kinase C, and tyrosine kinase which together induce LTP. The Ca2+/calmodulin kinase phosphorylates the AMPA receptors, increasing their conductance, and moves more of these receptors into the synaptic cell membrane from cytoplasmic storage sites. In addition, once LTP is induced, a chemical signal (possibly nitric oxide, NO) is released by the postsynaptic neuron and passes retrogradely to the presynaptic neuron, producing a long-term increase in the quantal release of glutamate.
FIGURE 15–3
Production of LTP in Schaffer collaterals in the hippocampus. Glutamate (Glu) released from the presynaptic neuron binds to AMPA and NMDA receptors in the membrane of the postsynaptic neuron. The depolarization triggered by activation of the AMPA receptors relieves the Mg2+ block in the NMDA receptor channel, and Ca2+ enters the neuron with Na+. The increase in cytoplasmic Ca2+ activates Ca2+/calmodulin kinase, protein kinase C, and tyrosine kinase which together induce LTP. The Ca2+
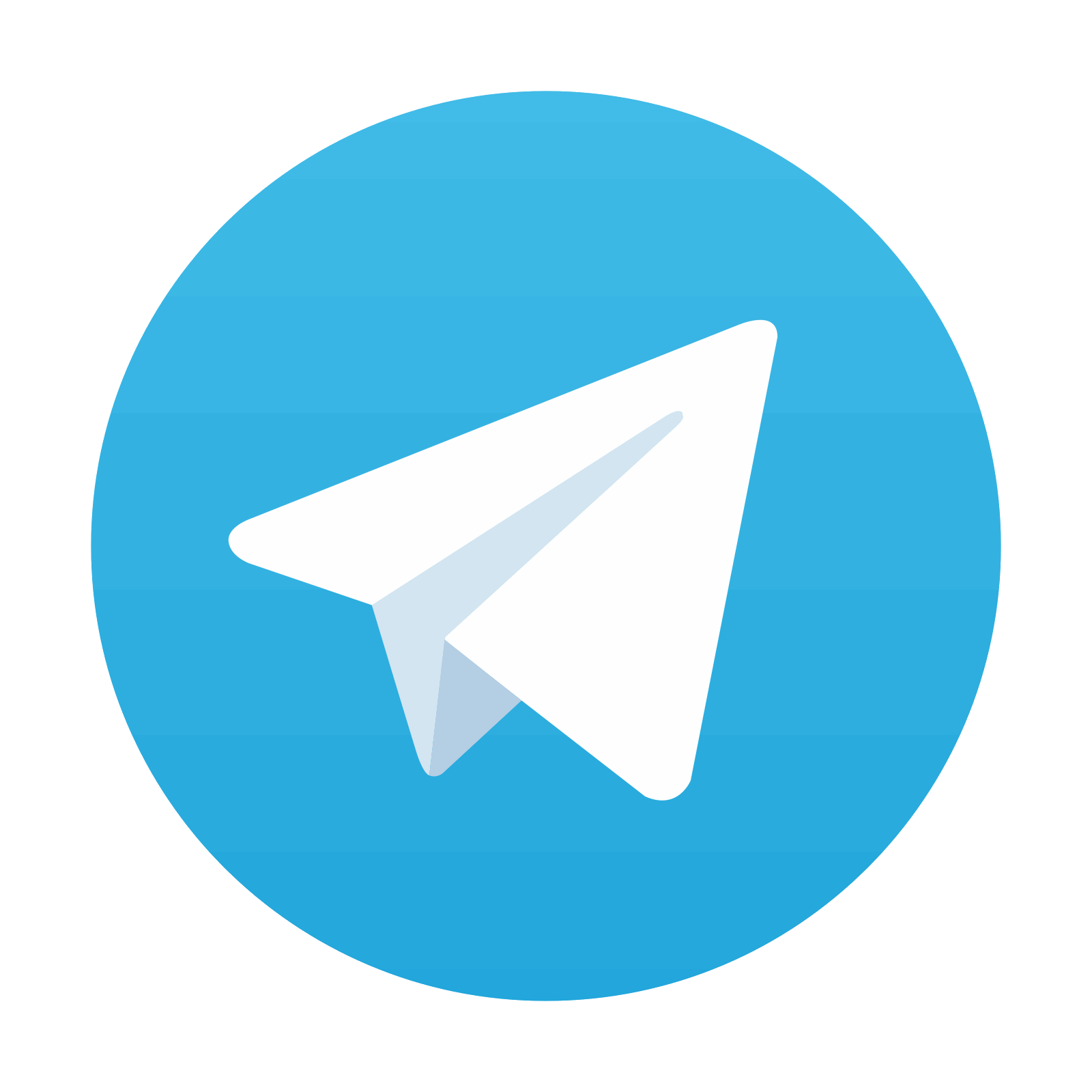
Stay updated, free articles. Join our Telegram channel

Full access? Get Clinical Tree
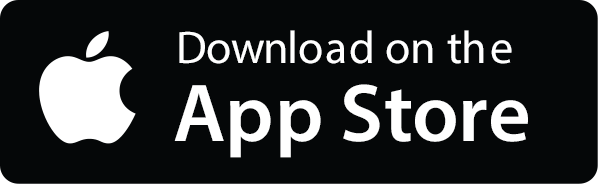
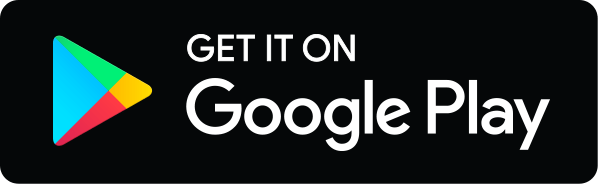
