Figure 83-1. Anatomy of the cardiac circulation. A: Anterior view. B: Posterior view.
Venous anatomy is more predictable, and very little pathology within this system is encountered. Three named venous branches can be identified: the middle cardiac vein in the posterior interventricular groove, the posterior cardiac vein along the obtuse margin of the heart, and the great (or anterior) cardiac vein along the anterior interventricular groove. All three drain into one confluence known as the coronary sinus along the posterior atrioventricular groove (Fig. 83-1). The coronary sinus empties posteriorly into the right atrium between the inferior vena cava and the annulus of the tricuspid valve. In addition to these identifiable named veins, the myocardium also drains directly into all chambers of the heart via thebesian veins.
1 Myocardial work consumes an enormous amount of energy substrate. In fact, only 10% to 20% of myocardial energy requirements are related to basal functions; the remainder is utilized during the continuous energy-dependent calcium mobilization and myofilament cross-linking from cyclic contraction and relaxation. Even at rest, the heart maximally extracts oxygen delivered from the bloodstream, leaving venous saturation in the coronary sinus typically 20% or less. Thus, increased energy requirements must be met with augmentation in blood oxygen delivery. Contrarily, other less oxygen-dependent tissues, where venous saturation can be as high as 80%, can extract additional oxygen during stress. When insufficient supply of oxygen is available, as may be the case with extreme consumption or limited blood flow, anaerobic metabolism cannot generate enough energy substrate to perform myocardial contraction.2 As a result, cardiac function deteriorates immediately and can be observed clinically essentially within heartbeats. Increases in cardiac oxygen consumption must be met with proportional increases in myocardial blood flow.
The heart and its coronary circulation have a unique capacity to dramatically increase blood flow, and thus oxygen delivery, during periods of increased needs.3 While basal coronary blood flow is approximately 10 mL/kg, this value can increase up to sixfold with exercise by autoregulatory mechanisms and feedback loops. Specifically, adenosine accumulates from the breakdown of the energy substrate adenosine triphosphate (ATP). Vasodilatory receptors within the media of coronary arteries are particularly avid for adenosine, increasing blood flow to myocardial regions with increased energy consumption. In addition, increased myocardial activity activates nitric oxide synthase within coronary endothelial cells, producing the powerful vasodilator nitric oxide. By immediately altering the vessel diameter and thus changing the resistance properties of epicardial vessels, these two short-acting mediators can rapidly alter coronary flow rates to meet changing energy requirements.
In addition to the high metabolic requirements of the heart and its dependence on responsive changes in blood flow, the coronary circulation is further uniquely challenged. During systole, increased cavitary pressure compresses intramyocardial vessels and virtually eliminates forward flow. As a result, blood flow to the left ventricle occurs only during diastole, so that myocardial perfusion depends not only on coronary arterial patency and caliber, but also on the diastolic pressure gradient and the duration of diastole. Tachycardia not only increases oxygen consumption, but also reduces the time available for myocardial perfusion, increasing the demands for epicardial flow. The rise in ventricular diastolic pressure seen in heart failure also reduces perfusion pressure and can jeopardize ischemia in vulnerable territories. With an understanding of cardiac perfusion needs and mechanics, it is apparent why occlusive lesions in coronary arteries can restrict blood flow, producing ischemia and infarction.
CORONARY ATHEROSCLEROSIS
Atherosclerosis is a progressive multifocal disease of medium and large muscular arteries of the systemic circulation. Atherosclerotic plaques or atheromas can occur anywhere in the systemic circulation, but tend to present clinically in varying quantities in the coronary circulation, carotid arteries, splanchnic vessels, and lower extremities. The lesions tend to occur predominantly at vessel bifurcations, sharp curvatures, and other regions creating pressure wave reflections and recirculation.4 All plaques consist of smooth muscle proliferation and intracellular and extracellular lipid deposition within the arterial intima (Fig. 83-2). The predecessor “fatty streaks” seen in the first two decades of life are small and unobstructing. However, under certain clinical circumstances, endothelial injury from cigarette smoke, hypercholesterolemia, hyperglycemia, hypertension, or other causes of inflammation initiates a cascade of events. These include endothelial dysfunction with expression of adhesion molecules, which bind circulating inflammatory cells like monocytes. The activated monocyte transmigrates into the vessel wall and ingests accumulated lipids. In turn, they release cytokines, which enhance endothelial cell adhesion molecule expression; alter endothelial cell nitric oxide production, promoting vasoconstriction and platelet activation; and stimulate activation of other inflammatory cells including neutrophils and T lymphocytes. The activated monocytes accelerate further lipid accumulation and smooth muscle cell proliferation. The end result is an enlarging plaque encroaching on the arterial lumen, separated from the bloodstream by a collagen-rich fibrous plaque.
Figure 83-2. The atherosclerotic plaque. A: The normal muscular artery consists of an internal intima with endothelium and internal elastic lamina. The smooth muscle of the vessel wall is in the media, and the thin adventitial layer contains connective tissue and the vasa vasorum. B: The first phase of an atherosclerotic lesion consists of focal thickening of the intima with smooth muscle cells and extracellular matrix and an initial accumulation of intercellular lipid deposits. C: Extracellular lipid may also develop. D: Intercellular and extracellular lipid in the earliest phase is referred to as a fatty streak. E: A fibrous plaque results as fibroblasts that cover the proliferating smooth muscle cells laden with lipids and cell debris continue to accumulate. The lesion becomes more complex as continuing cell degeneration leads to an ingress of blood constituents and calcification.
2 Within the coronary circulation, atherosclerotic stenotic lesions are generally restricted to the proximal regions of the large epicardial coronary arteries. In particular, stenosis found in the LAD and circumflex vessels are frequently isolated, short, and in the proximal segments. The right coronary artery, however, develops diffuse obstructions, although rarely extending into the posterior descending or intramural branches. For reasons that are unclear, atherosclerosis is rarely found within intramyocardial segments of the coronary arteries. Symptoms from these atherosclerotic plaques can occur from a variety of proposed mechanisms. First, the lesion can cause flow limitations, particularly when the luminal cross-sectional area is reduced by at least 75%. With this degree of obstruction, the vasodilatory reserve required during increased myocardial demand is restricted, resulting in transient myocardial ischemia until demand returns to baseline, also known as exertional angina. The atherosclerotic plaque also causes coronary ischemia when the lesion becomes unstable. The fibrous plaque can fracture or rupture, exposing the bloodstream to the highly thrombogenic internal plaque contents. This can lead to complete epicardial thrombosis, the presumed mechanism of ST-segment elevation myocardial infarction (STEMI). Additionally, subtotal plaque disruption can cause vasoconstriction, platelet activation, and embolization, resulting in ischemia without total occlusion of the epicardial vessel. This is the presumed mechanism of unstable angina and non–ST-segment elevation myocardial infarction (NSTEMI). Some plaques are more prone to rupture than others, and the composition of the fibrous cap may be an identifiable feature of an unstable lesion. Activation of inflammatory cells may increase the activity of matrix metalloproteinases, which degrade components of the fibrous cap. Some theorize that certain stressors can stimulate activation of these inflammatory cells, such as acute infections, extreme cold, and even emotional distress.5 The inflammatory component of coronary artery disease has been demonstrated by the strong relationship between C-reactive protein and the incidence of acute myocardial infarctions.6
Although the characteristics, locations, and severity of lesions in each person can vary, a number of established risk factors appear to predispose to atherosclerosis. These include advanced age, family history, male sex, hypertension, diabetes mellitus, dyslipidemia, and cigarette smoking. Recognizing the presence of these risk factors can help identify patients at risk of coronary atherosclerosis. In addition, some of these risk factors are modifiable, and simple interventions can reduce their risk of future cardiac events.
Hypertension is strongly associated with the development of atherosclerotic heart disease and the risk of death from cardiovascular causes. Although the mechanism is uncertain, it has been suggested that an increase in shear stress at particular vessel locations may injure the vascular endothelium, predisposing to lipid deposition and plaque development. Systolic hypertension appears to be more predictive than diastolic hypertension, particularly in elderly patients. Numerous interventions can control hypertension, but the most effective appears to be lifestyle modifications including reduction in dietary sodium, exercise, and weight loss. Several classes of medications are used for blood pressure control, and each is variably efficacious in different populations of patients.7 Unequivocally, reduction in elevated systolic blood pressure reduces the risk of death from cardiovascular disease, and in particular of atherosclerotic coronary artery disease.
Patients with diabetes are two to four times more likely to have coronary artery disease, and even more so when they are insulin dependent and in diabetic women. In addition, patients with diabetes and coronary disease have worse outcomes than age-matched nondiabetics. Unfortunately, rigorous control of elevated blood glucose concentrations by insulin does not appear to affect coronary mortality as well as control of other risk factors such as hypertension, dyslipidemia, and smoking cessation does.8
Dyslipidemia is associated with accelerated coronary artery disease. This includes both elevation of total cholesterol levels and an imbalance in the ratio of the subcomponents of cholesterol: high-density lipoprotein (HDL) and low-density lipoprotein (LDL).9 HDL levels appear to be protective, as the total HDL level is inversely proportional to the risk for the development of coronary artery disease. Conversely, LDL levels are directly proportional to cardiovascular risk. Importantly, alteration in total cholesterol and the ratio of HDL to LDL by dietary changes, medications, and exercise can reduce the risk of cardiovascular events.10
Cigarette smoking is one of the most important risk factors for coronary artery disease. Carbon monoxide and nicotine directly adversely affect endothelial cell function. In addition, cigarette smoke can increase LDL levels, reduce HDL levels, increase fibrinogen levels, and increase platelet aggregation. Regular smokers have a four to five times higher rate of cardiovascular death than nonsmokers, and there appears to be a dose relationship, with heavier smokers having a higher prevalence of coronary disease than lighter smokers. Fortunately, the risk of developing coronary artery disease is reduced by 50% after 1 year of smoking abstinence, and at 10 years the risk is no different from that of those who never smoked.11
PATIENT PRESENTATION
The clinical manifestations of ischemic heart disease result from an imbalance of myocardial oxygen supply and consumption. The symptoms and acuity depend on the severity and nature of the patient’s occlusive lesions, but also on his or her other medical comorbidities. As many as 25% of patients diagnosed with coronary artery disease have no clear symptoms. The diagnosis is often made based on abnormalities identified on screening tests obtained because of the presence of worrisome risk factors.
3 Chronic stable angina is the most frequent complaint of the patient with coronary artery disease. At rest, coronary blood flow is adequate to meet myocardial demand, and patients are without symptoms. However, during exercise or stress, as myocardial oxygen demand increases, the autoregulatory mechanisms to vasodilate and increase myocardial blood flow are constrained. Significant coronary obstructing atheromas become flow limiting, resulting in an imbalance of oxygen demand and supply. Chest pain develops rapidly and builds up quickly, typically described as tightness, squeezing, constricting, or aching. It is usually midsternal and radiates to the left shoulder, arm, jaw, or neck. The classically described Levine sign with a clenched fist over the sternum is a common finding. Some patients, however, will describe symptoms that are collectively referred to as “anginal equivalents.” These include dyspnea, diaphoresis, nausea, abdominal pain, heartburn, and dizziness or presyncope. Although the differential diagnoses of these “atypical” symptoms are broad, they should prompt the clinician to think of coronary disease, particularly if risk factors predominate. Interestingly, women are more likely to describe atypical symptoms, resulting in late or missed diagnosis of coronary atherosclerosis. Although the clinical manifestations of angina are variable, the pathognomonic feature of chronic stable angina is that the symptoms occur predictably with exertion and are always relieved with rest. Symptoms of patients with coronary atherosclerosis can be categorized according to the Canadian Heart Association Classification scheme. Class I patients have no symptoms, class II patients have angina with significant exertion, class III patients describe angina with mild exertion, and class IV patients have angina at rest.
Acute coronary syndromes (ACSs) refer to a spectrum of accelerated coronary occlusive disease states and include unstable angina, NSTEMI, and STEMI. Approximately 1 million people are hospitalized in the United States each year with a diagnosis of ACS. Unstable angina refers to patients with chest pain or an anginal equivalent that is new in onset, occurs at rest, or occurs with increasing severity and frequency from their baseline chronic stable symptoms, also referred to as crescendo angina. Patients who develop an NSTEMI have evidence of myocardial injury with elevated blood levels of myocardial enzymes (troponin and the MB fraction of creatine kinase). Unstable angina and NSTEMI are important prognostic indicators, as 10% of patients will die of cardiovascular causes within 6 months.
STEMI represents the consequences of large epicardial vessel occlusion, typically associated with plaque rupture. Patients describe a severe retrosternal pain that persists for at least 30 minutes, but usually for several hours. The pain is frequently characterized as crushing, squeezing, or boring, and can radiate down the left arm or into the jaw. Patients with previous chronic stable angina will report that the current pain is similar to but more intense than their baseline symptoms and has not resolved with rest or nitroglycerine. However, many patients that present with an STEMI never had chronic stable angina. Patients will often describe additional symptoms such as diaphoresis, nausea, dizziness, and epigastric pain. In emergency departments, the pain is often mistaken for gastroenteritis or indigestion, particularly in women. Often in elderly patients and those with diabetes, chest pain may not be present at all, and the only symptom associated with STEMI is heart failure from progressive loss of myocardial contractile function. Although improvements in health systems have drastically increased survival from myocardial infarction, mortality for STEMI remains near 10%.
COMPLICATIONS OF ACUTE MYOCARDIAL INFARCTIONS
4 STEMIs can create widespread myocyte necrosis, often resulting in catastrophic complications that require urgent intervention. Anticipation and early diagnosis can improve outcomes. These complications include cardiogenic shock, postinfarct ventricular septal defect (VSD), free wall rupture, and acute mitral valve regurgitation (Table 83-1).
Cardiogenic Shock
Cardiogenic shock is a state in which cardiac output is insufficient to meet metabolic demands, despite adequate intravascular filling pressures. Between 5% and 10% of patients with acute myocardial infarctions develop cardiogenic shock. A large proportion of these patients progress to shock not upon arrival, but more than 24 hours after initial presentation.12 Overall mortality is approximately 60%, with very little improvement over recent decades. Treatments include fluids and inotropic agents to optimize myocardial contraction. Insertion of an intra-aortic balloon counterpulsation pump (IABP) can improve coronary perfusion by diastolic augmentation of perfusion pressure. This can have a profound impact by improving contractile function of peri-infarct territories. Although there is significant afterload reduction afforded by the IABP, cardiac output typically increases by only 15% to 20%. Emergent salvage revascularization can reduce mortality, with sustained benefits seen over long-term follow-up.13 When shock persists despite using these aggressive strategies, consideration must be made regarding the patient’s candidacy for mechanical circulatory support using ventricular assist devices or extracorporeal membrane oxygenation.14 While these options may only be available in selected regional medical centers, durable long-term survival can be obtained in a highly selected patient population.15
Postinfarction Ventricular Septal Defect
A postinfarction VSD is an infrequent complication occurring after fewer than 1% of acute myocardial infarctions. The infarct is most commonly in the LAD territory, with the defect in the distal septum. Alternatively, a postinfarct VSD can form from acute right coronary occlusion, with the infarct predominantly in the posterobasilar septum. They typically present 5 to 10 days following initial presentation, but can occur earlier, particularly if late thrombolytic therapy was administered. The diagnosis should be considered in a patient in whom cardiogenic shock with refractory hypotension and pulmonary edema develops suddenly following a myocardial infarction. On physical examination, an unmistakable holosystolic murmur can be heard over the entire precordium. The diagnosis is confirmed with echocardiography using Doppler techniques to demonstrate flow across the interventricular septum. Right heart catheterization will reveal a step-up in oxygen saturation from the right atrium to the pulmonary artery. Medical therapy involves stabilization to optimize cardiac output and end-organ perfusion. Refractory hypotension is typical, excluding the use of afterload reducing agents. Intra-aortic balloon counterpulsation can occasionally be helpful for afterload reduction and to optimize coronary perfusion. Emergent surgical intervention is required if there is any hope of survival. Infarcted muscle is débrided, and the defect is typically closed using prosthetic material. For surgical candidates, survival rates of approximately 50% can be expected.16
Papillary Muscle Rupture
Severe regurgitation of the mitral valve can occur after acute myocardial infarctions, with a frequency of less than 1%. The mechanism is related to infarction of the tip or trunk of one of the papillary muscles, with resultant disruption of the mitral subvalvar apparatus and failed leaflet coaptation. Posteroinferior MIs lead to this complication two to three times more frequently than anterior infarctions, presumably because there is more collateral blood flow to the anterolateral papillary muscle compared to the posteriomedial. While chronic mitral regurgitation can be well tolerated through adaptive changes in the left atrium and pulmonary vascular bed, severe acute mitral regurgitation results in immediate heart failure, as the small left atrium offers little compliance for the massive volume overload. Papillary muscle rupture develops typically between the third and fifth days following myocardial infarction, when infarcted myocardium is at its weakest. However, it can present within the first 24 hours. Patients will describe acute-onset dyspnea, pulmonary edema, and possibly signs of cardiogenic shock. The diagnosis should be suspected in any patient after myocardial infarction with a new holosystolic murmur heard at the apex, associated with dyspnea and hypotension. Bedside surface echocardiography typically demonstrates the mitral regurgitation, and the flail leaflet may be seen. For more definitive imaging, transesophageal echocardiography (TEE) can be performed. Not only will the mitral valve pathology be clearly seen, but also alternative diagnoses such as a postinfarct VSD can be excluded. In addition to the treatments typically employed for myocardial infarctions, immediate medical therapy of ruptured papillary muscles involves afterload reduction when tolerated, with infusions of nitroglycerine or nitroprusside. If cardiac output cannot be maintained, intra-aortic balloon counterpulsation can be helpful. Definitive therapy involves prompt surgical correction. Although mortality rates are generally high, there are no survivors without surgical correction.17 If coronary angiography demonstrated occlusive epicardial lesions in territories other than the acute infarction, simultaneous coronary bypass grafting can be performed. Under most circumstances, the diseased mitral valve is excised and replaced. Because the ventricular cavity is typically small, the valve is usually replaced with a low-profile mechanical prosthesis to avoid left ventricular outflow obstruction from the protruding struts of stented bioprosthetic valves.
Table 83-1 Complications of Acute Myocardial Infarctions
Ventricular Free Wall Rupture
Ventricular free wall rupture after myocardial infarction is infrequently encountered clinically, likely because of the exceedingly high mortality. The actual incidence is unknown, but may represent up to 30% of all deaths after acute myocardial infarctions. Like postinfarct VSD and papillary muscle ruptures, they tend to occur between the third and sixth days after infarction, when the myocardium is at its weakest. Free wall ruptures can present in essentially one of two ways: (a) a simple full-thickness tear with catastrophic hemorrhage and death from tamponade or (b) a complex serpiginous tear, partially contained. The latter is more likely to require surgical intervention, often presenting weeks from the initial infarction with symptoms of delayed cardiac tamponade. In rare cases, it is completely contained and may go unrecognized until a pseudoaneurysm develops, which is often diagnosed at a later date. Patients with free wall rupture will typically present with cardiogenic shock and may have features suggesting tamponade. Echocardiography will demonstrate a large pericardial effusion, frequently with echo-dense clot or ventricular wall defects. Urgent surgical intervention is required, often without coronary angiography or other time-consuming diagnostic tests. Once the pericardium is opened and cardiac tamponade is relieved, hemodynamic stability is often achieved. The site of hemorrhage is identified by the necrotic-appearing myocardium and adherent thrombus. The defect can be closed with large mattress sutures reinforced with Teflon felt strips. Care must be taken while tying the suture, as the necrotic muscle is weak and friable. Some have advocated covering the defect with a large Teflon felt patch using biologic adhesives, avoiding sutures altogether.18 Whatever the technique, outcomes are likely determined by the hemodynamic status of the patient prior to arrival in the operating room and the quality of the remaining viable myocardium.
Figure 83-3. The electrocardiogram in an acute myocardial infarction.
DIAGNOSIS
The initial diagnostic tool for patients presenting with chest pain is the resting electrocardiogram (ECG). Although the majority of patients with chronic stable angina will have a normal ECG pattern, evidence of previous myocardial infarctions may be identified, either with the presence of Q waves or conduction abnormalities. Patients with an acute myocardial infarction may demonstrate ST-segment elevation (Fig. 83-3), prompting urgent intervention. Occasionally, more subtle ECG findings may provide clues that the chest pain is ischemic in etiology. Although nonspecific, these changes may include mild ST-segment depression or T-wave inversions.
During a myocardial infarction, the dying myocytes release enzymes specific to cardiac muscle into the bloodstream. These enzymes can be detected from laboratory analysis and are pathognomonic of myocardial infarction. The MB fraction of creatine kinase is cardiac specific and rises within 8 to 24 hours of infarction and returns to baseline within 2 days. Cardiac troponin has even greater specificity and serum levels rise faster than creatine kinase, improving both the speed and accuracy in the laboratory diagnosis of an acute myocardial infarction in the absence of clear ECG changes.19
For asymptomatic patients, or those with chronic stable angina, the most widely used diagnostic test to evaluate for coronary artery disease is exercise electrocardiography, or a “stress test.” Using standardized protocols, patients are exercised on a treadmill while the 12-lead ECG is continuously recorded. Alternatively, for those patients unable to walk, a bicycle ergometer may be used. Testing is continued until patient symptoms are noted or until the development of significant downward-sloping ST-segment depression, suggesting myocardial ischemia (Fig. 83-4). The diagnostic accuracy of exercise stress ECG testing can be enhanced with myocardial perfusion imaging. Several radioactive tracers are used clinically, the most frequent of which is thallium-201. Because of its similarities to potassium ions, it is taken up preferentially by viable cardiac myocytes. Its distribution within the myocardium is proportional to the rate of perfusion. During stress, regions of underperfused myocardium will take up less thallium, resulting in a visible defect. Following a period of rest and reperfusion, thallium uptake normalizes, demonstrating a “reversible defect.”
Figure 83-4. Electrocardiogram during an exercise test showing precordial leads V1 through V6. A: During exercise, depression of the ST segment and ischemia are seen in leads V4 through V6. B: These resolve after exercise is stopped.
In some cases, patients are unable to exercise because of additional physical or psychological limitations. Pharmacologic agents can substitute for exercise by increasing myocardial oxygen demand (dobutamine) or by directly vasodilating coronary arteries (adenosine or dipyridamole), thus demonstrating regions with fixed restrictions in myocardial blood flow. Echocardiography can be used as an alternative to nuclear perfusion imaging to improve the accuracy of exercise ECG testing. Regional changes in wall motion will be observed during myocardial ischemia, followed by restoration of normal myocardial contraction after rest or discontinuation of dobutamine. Echocardiography can also identify valvular abnormalities or other conditions that may influence treatment choices.
Coronary angiography, also known as cardiac catheterization, is the definitive tool for diagnosing coronary atherosclerotic disease. Indications include symptomatic patients with typical angina refractory to medical treatments, patients with significant ischemia on exercise or pharmacologic stress testing, patients with a STEMI, or patients with an NSTEMI and persistent pain despite medical treatment. Patients with valvular heart disease who are scheduled to undergo surgical correction should also undergo coronary angiography to identify coexisting coronary occlusions, which can be addressed at the time of the valve surgery. After percutaneous access is obtained in the femoral, brachial, or radial arteries, preformed catheters of varying sizes are advanced fluoroscopically to selectively engage the ostia of the left and right coronary arteries. Radio-opaque contrast is injected with imaging of the opacified coronary artery. Standardized views are obtained of both the right and left coronary systems to provide different projections to clearly define the vascular anatomy and to quantify the severity of occlusive lesions (Fig. 83-5). Automated computer analysis systems can calculate area reduction, improving interobserver consistency. Additionally, catheters can be inserted across the aortic valve into the left ventricular cavity, providing information about ventricular pressure throughout the cardiac cycle. Contrast injection for ventriculography can illustrate ventricular systolic function, cavity size, and the presence of left-sided valvular abnormalities. New microtipped pressure sensors can be advanced across coronary lesions and document a clinically significant drop in perfusion pressure, either at rest or after provocation with vasodilating agents.20 In addition, intravascular ultrasound imaging sensors can now be loaded onto tiny catheters and advanced into the left main coronary artery, helping to clarify the significance of lesions that may appear equivocal in severity on standard angiography.21
Newer imaging techniques using high-resolution multislice computed tomography (CT) scanning with three-dimensional reconstruction and magnetic resonance imaging are increasingly being utilized. These noninvasive approaches have the potential to improve the safety and convenience of coronary imaging; however, resolution remains inferior to standard coronary angiography. These techniques are often used as screening tests and are frequently followed up with traditional angiography.22
MEDICAL TREATMENT
5 Medical therapy of coronary artery disease is designed to slow the rate of progression of coronary artery atherosclerosis, to reduce the rate of complications from these lesions, and to control symptoms. Treatments are aimed at slowing plaque growth, reducing risk of plaque rupture, and reducing myocardial oxygen consumption. While advances in pharmacologic agents have improved the treatment of atherosclerosis and reduced mortality, lifestyle modification remains the most important intervention and the most difficult to achieve. These lifestyle modifications include cessation of cigarette smoking, weight loss, dietary control of diabetes, salt restriction, reduction in the consumption of foods high in cholesterol and fat, and exercise.
Medical therapy begins with controlling risk factors that contribute to formation and destabilization of the atherosclerotic plaque. Medical treatment alone is often satisfactory for many patients with coronary artery disease affecting only one or two epicardial vessel territories. Statins can decrease cholesterol levels and improve the ratio of LDL to HDL and have been demonstrated to reduce rates of myocardial infarction and death.23 Statins also appear to reduce macrophage accumulation within atheromatous plaques, matrix metalloprotease activity, and collagen degradation. Angiotensin-converting enzyme (ACE) inhibitors have been shown to reduce mortality and myocardial infarction in patients with coronary artery disease.24 Aspirin inhibits cyclooxygenase-1 (COX-1) activity and thromboxane production, irreversibly inhibiting platelet aggregation by inhibiting platelet activity, and should be prescribed to all patients unless significant contraindications exist. Aspirin has been shown to reduce death and myocardial infarctions in patients with significant coronary artery disease. By binding the catecholamine-mediated B1 receptor on cardiac myocytes, beta-blocking agents reduce myocardial oxygen consumption by reducing heart rate and contractility, and increase perfusion by extending diastolic perfusion time. Beta blockers are effective at controlling angina26 and, in selected patients, reduce cardiovascular mortality.27 Nitrates also reduce myocardial oxygen demand by decreasing cardiac preload via venodilation and by reducing afterload via arterial vasodilation. Some epicardial coronary vasodilation will occur, improving coronary blood flow. Nitrates can be given sublingually for immediate relief or as an oral long-acting agent for continuous control of symptoms. Headaches and tolerance are notable side effects. For patients who have refractory angina despite all revascularization and traditional medical options, a newer agent, ranolazine, has recently been introduced. It alters cardiac myocyte membrane ion channel permeability and can relieve angina with few side effects.28
Figure 83-5. Coronary angiography. A: Left anterior oblique view of the left coronary artery. B: Right anterior oblique view of the left coronary artery. C: Left anterior oblique view of the right coronary artery. (Images courtesy of Brahmajee Nallamothu.)
A patient admitted to the hospital with a diagnosis of acute myocardial infarction or possible ACS should be placed in an environment with continuous ECG monitoring and the capacity to perform emergent defibrillation, since these patients are at risk of life-threatening malignant ventricular arrhythmias. Patients with evidence of ST-segment elevation should undergo emergent cardiac catheterization when possible, as will be discussed later. For those patients in which the ECG is normal but an ACS is suspected, a stress test should be performed expeditiously to identify their risk for future coronary events and to identify patients in need of urgent cardiac catheterization.
Unless there is a strong contraindication, aspirin should be administered immediately, ideally chewed to increase the rate of bioavailability. Typically, aspirin is given by emergency transport personnel. Patients may be instructed by 911 operators to take aspirin if they describe chest pain. Institution of oxygen therapy is important to maximizing oxygen delivery, particularly for patients in whom hypoxemic respiratory dysfunction is also present. Intravenous nitroglycerine as a continuous infusion is typically effective at controlling symptoms of pain, which is essential in reducing unnecessary myocardial strain. Small randomized trials have suggested that nitroglycerine can reduce hospital mortality, although this has not been validated in larger trials.29 When necessary, narcotics should be added, as unrelenting pain not only increases myocardial oxygen consumption, but also may contribute to plaque instability. Beta-blocking agents can reduce myocardial oxygen consumption but can also exacerbate heart failure, particularly in the setting of severely decreased systolic ventricular function. They should be considered cautiously in hemodynamically stable patients. ACE inhibitors reduce mortality after acute myocardial infarctions, particularly those with decreased ventricular function.30 Benefits are not just restricted to the afterload reduction effects. Some evidence suggests they have a role in alteration of the myocardial intercellular matrix and scar formation.31
Anticoagulation is among the most important interventions in patients presenting with an acute myocardial infarction. In recent years, patients with myocardial infarctions were placed on newer antiplatelet glycoprotein (Gp) IIb/IIIa inhibitors, which affect adenosine diphosphate (ADP)-mediated platelet adhesion. Thienopyridines are a newer class of antiplatelet agents that act by binding to the ADP receptors on the platelet surface and inhibiting the GpIIb/IIIa pathway. Specific agents include clopidogrel (Plavix) and ticlopidine (Ticlid). There is strong evidence that the addition of clopidogrel to aspirin reduces death, myocardial infarction, and stroke in the setting of ACS.32 Clopidogrel is irreversible and has a long half-life, resulting in bleeding complications, particularly if surgery is required. Surgical intervention should ideally be delayed for 3 to 5 days.33 Abciximab (ReoPro) is a murine antibody that binds directly to the GpIIb/IIIa receptor on platelet surfaces, preventing fibrinogen binding and activation. The half-life is short, and platelet activity generally returns to normal within 24 hours. Eptifibatide (Integrilin) is a synthetic antagonist of the GpIIa/IIIb receptor and has a half-life of 3 to 4 hours. The benefit of these agents in patients with ACS treated medically is unclear, but they have become routine in the setting of percutaneous treatment of coronary stenoses. Unless contraindications exist, inhibition of the coagulation cascade is also recommended for patients with acute myocardial infarctions. Although standard unfractionated heparin has been used for many years, newer low–molecular-weight agents and direct Xa inhibitors may have advantages and are used with increasing frequency.34,35
With STEMI, significant myocardium is at risk, potentially resulting in either cardiogenic shock or ventricular arrhythmias. Emergency recanalization of acutely occluded vessels can salvage cardiac function and save lives. Various fibrinolytic drugs, which convert plasminogen to plasmin, can be given emergently and restore flow in vessels obstructed by acute thrombus. A number of clinical trials were performed in the 1980s using streptokinase and demonstrated reduced survival when it was given within 12 hours.36 Newer agents are now available including tissue plasminogen inhibitor (tPA) and reteplase, which are faster in onset and may further improve outcomes, although they are more costly.
The main drawbacks of fibrinolytic therapy are bleeding complications and failure of recanalization. Systemic intravenous thrombolytic therapy unquestionably decreases morbidity and mortality after MI and continues to be used in 40% to 50% of eligible patients. The earlier the treatment, the greater the impact, with the greatest benefit accruing in patients treated within 1 to 2 hours after the onset of symptoms.
In 1977, Dr. Andreas Gruentzig performed the first percutaneous intervention (PCI) with balloon angioplasty on a stenotic lesion in the LAD. This pioneer laid the foundation for a revolution in the treatment of coronary artery disease worldwide. Percutaneous treatment of acute STEMI is now standard practice in centers in which this therapy is available, with outcomes that are superior to pharmacologic reperfusion.37 Although percutaneous intervention may be better than fibrinolysis, the treatment requires numerous experienced personnel and highly technologic equipment, which is not always available in every facility. Complex interhospital and intrahospital systems are now in place to improve the efficiency and application of this treatment strategy, and guidelines have defined minimum “door to balloon” times to salvage myocardium at risk.38
PERCUTANEOUS CORONARY INTERVENTIONS
6 Since the inception of percutaneous transcoronary balloon angioplasty (PTCA), catheter-directed treatment of coronary artery disease has evolved into one of the most commonly performed procedures worldwide. Advances in technology have improved outcomes and expanded the indications, particularly for the acutely ischemic heart. Using the same techniques described for cardiac catheterization, small, highly flexible and steerable guide wires can be advanced through the lumen of epicardial coronary arteries. Over this guide wire, balloon-tipped catheters can be advanced and centered across discrete stenotic lesions and inflated to 4 to 10 atmospheric pressures, stretching and dilating the affected vessel (Fig. 83-6). Typically, the vessel tears or cracks at the junction of the plaque and the normal vessel wall. Acute thrombosis or coronary dissection could result in immediate closure of the vessel, often necessitating emergent surgical coronary bypass grafting in approximately 5% of cases. Restenosis is common, occurring in nearly 40% to 50% of patients, either from mechanical elastic recoil or progressive neointimal hyperplasia.
The addition of stents, nitinol scaffolding devices, has nearly eliminated acute vessel occlusion, and the need for salvage surgical revascularization is below 1%. In addition, stents have greatly reduced restenosis rates down to nearly 20% and are used in nearly 90% of percutaneous procedures in the United States. The stents are delivered crimped onto a deflated balloon. With balloon inflation, the stent expands, restoring the vessel lumen to its anatomic dimensions. The stent prevents elastic recoil and coronary dissection, improving immediate outcomes, but the problem of neointimal hyperplasia remains, resulting in the persistent rates of restenosis. Stents have been improved even further by impregnating antiproliferative drugs onto the scaffolds. Sirolimus and paclitaxel are immunosuppressants that act by different mechanisms. Paclitaxel is a mitotic inhibitor, which retards microtubule breakdown. Sirolimus binds intracellularly to FK-binding protein and inhibits interleukin-2 (IL-2) production via the target of rapamycin pathway. Both sirolimus- and paclitaxel-eluting stents have further reduced the rate of target vessel restenosis and the need for repeat interventions.39 However, reports of late stent thrombosis have raised concerns, and indefinite use of antiplatelet regimens has been recommended.40
CORONARY BYPASS GRAFTING
Indications
Coronary artery bypass grafting (CABG) is one of the most common and most studied surgical procedures performed in the United States. As one might expect, CABG consumes more resources than any other single cardiovascular procedure. Dozens of multicenter, prospective, randomized trials have been performed comparing the surgical treatment of coronary artery disease to both medical and percutaneous options. Some of these landmark studies will be discussed in detail later in the chapter, as proper understanding of these trials is important in determining appropriate treatment strategies for individual patients. In summary, these studies consistently demonstrate that CABG continues to be the best revascularization strategy, reducing rate of both myocardial infarctions and the need for repeat revascularization in patients with a broad degree of occlusive disease. A task force made up of numerous medical societies including the American College of Cardiology, the American Heart Association, the Society of Thoracic Surgeons (STS), and the American Association of Thoracic Surgeons recently published guidelines on the indications for coronary artery revascularization and made recommendations regarding the mode of revascularization.41 The group considered a number of variables, including clinical symptoms and mode of presentation, the degree of ischemia determined by noninvasive imaging, and the severity of coronary disease based on angiographic imaging. In summary, patients are more likely to benefit from surgical revascularization if they have worse symptoms, more severe myocardial ischemia, and more extensive coronary artery occlusions. The recommendations are summarized in Figure 83-7, where recommendations are categorized as “appropriate,” “uncertain,” and “inappropriate.” Interestingly, these guidelines have not changed markedly over the last several decades, despite vast improvements in both medical treatment and percutaneous technology. Today, CABG remains the best mode of cardiac revascularization in symptomatic patients.
Figure 83-6. Percutaneous coronary intervention. A: A stenotic midright coronary artery lesion. B: Balloon angioplasty. C: Postintervention result. (Images courtesy of Brahmajee Nallamothu.)
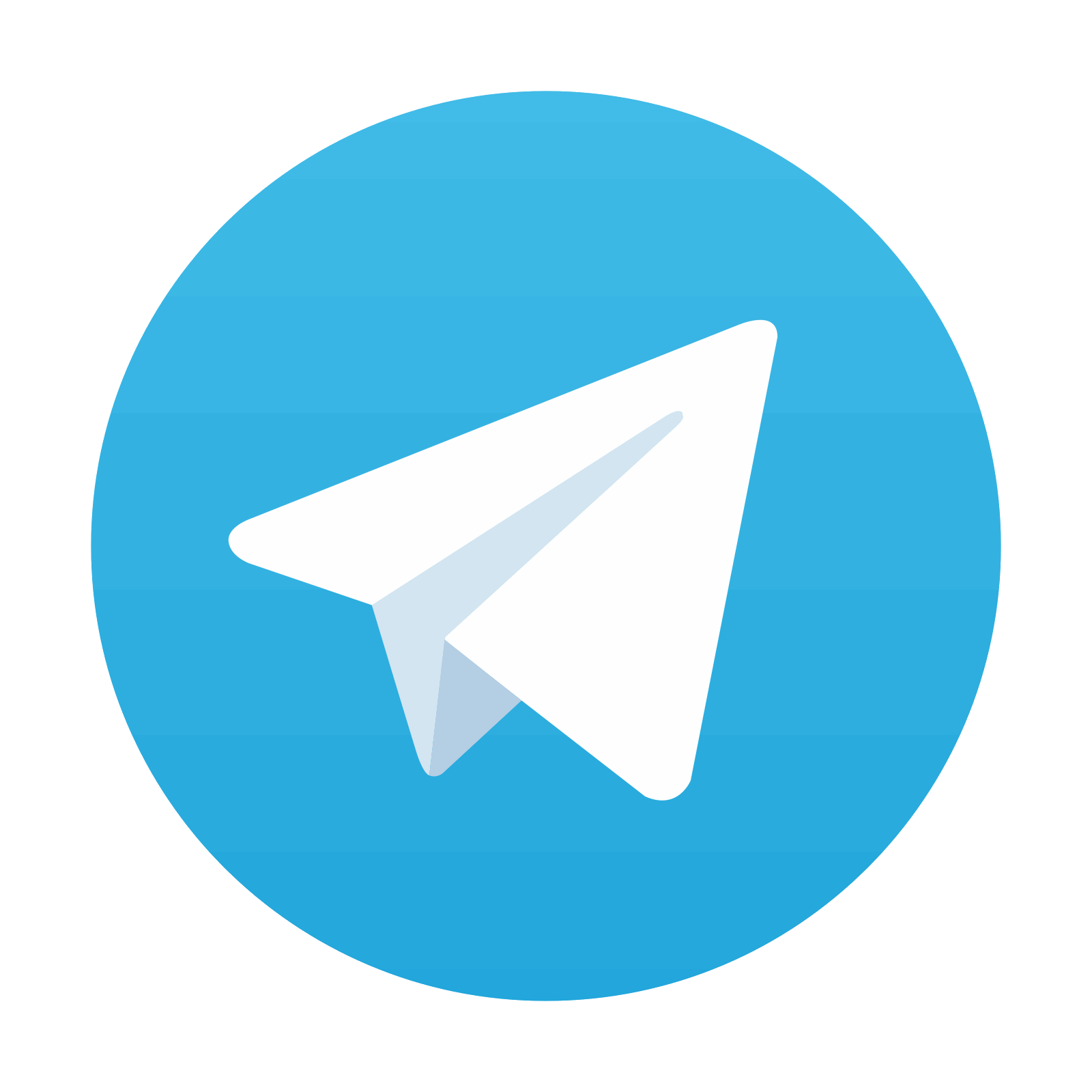
Stay updated, free articles. Join our Telegram channel

Full access? Get Clinical Tree
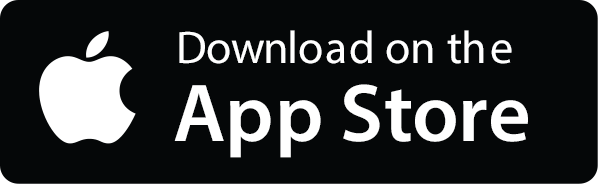
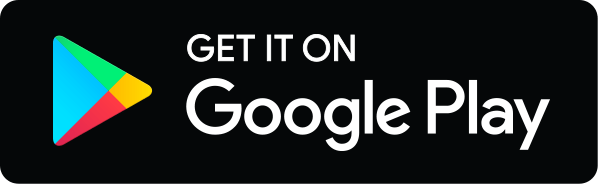