CHAPTER 37 Introduction to the Endocrine System
The ability of cells to communicate with each other represents an underpinning of human biology. As discussed in Chapter 3, cell-to-cell communication exists at various levels of complexity and distance. Endocrine signaling involves (1) the regulated secretion of an extracellular signaling molecule, called a hormone, into the extracellular fluid; (2) diffusion of the hormone into the vasculature and its circulation throughout the body; and (3) diffusion of the hormone out of the vascular compartment into the extracellular space and binding to a specific receptor within cells of a target organ. Because of the spread of hormones throughout the body, one hormone often regulates the activity of several target organs. Conversely, cells frequently express receptors for multiple hormones.
The endocrine system is a collection of glands whose function is to regulate multiple organs within the body to (1) meet the growth and reproductive needs of the organism and (2) respond to fluctuations within the internal environment, including various types of stress. The endocrine system comprises the following major glands (Fig. 37-1):
These endocrine glands synthesize and secrete bioactive hormones and, with the exception of gonads, which perform both endocrine and gametogenic functions, are dedicated to hormone production (Table 37-1). A transitory organ, the placenta, also performs a major endocrine function.
Table 37-1 Hormones and Their Sites of Production in Nonpregnant Adults
Gland | Hormone |
---|---|
Hormones Synthesized and Secreted by Dedicated Endocrine Glands | |
Pituitary gland | |
Thyroid gland | |
Parathyroid glands | Parathyroid hormone (PTH) |
Islets of Langerhans (endocrine pancreas) | |
Adrenal gland | |
Ovaries | |
Testes | |
Hormones Synthesized in Organs with a Primary Function Other Than Endocrine | |
Brain (hypothalamus) | |
Brain (pineal gland) | Melatonin |
Heart | Atrial natriuretic peptide (ANP) |
Kidney | Erythropoietin |
Adipose tissue | |
Stomach | |
Intestines | |
Liver | Insulin-like growth factor type I (IGF-I) |
Hormones Produced to a Significant Degree by Peripheral Conversion | |
Lungs | Angiotensin II |
Kidney | 1,25-Dihydroxyvitamin D (vitamin D) |
Adipose, mammary glands, other organs | Estradiol-17β |
Liver, sebaceous gland, other organs | Testosterone |
Genital skin, prostate, other organs | 5-Dihydrotestosterone (DHT) |
Many organs | T3 |
In addition to dedicated endocrine glands, there are endocrine cells within organs whose primary function is not endocrine (Table 37-1). These include cells within the heart that produce atrial natriuretic peptide, liver cells that produce insulin-like growth factor type I (IGF-I), cells within the kidney that produce erythropoietin, and numerous cell types within the gastrointestinal tract that produce gastrointestinal hormones. There also exist collections of cell bodies (called nuclei) within the hypothalamus that secrete peptides, called neurohormones, into capillaries associated with the pituitary gland.
A third arm of the endocrine system is represented by numerous cell types that express intracellular enzymes, ectoenzymes, or secreted enzymes that modify inactive precursors or less active hormones into highly active hormones (Table 37-1). An example is the generation of angiotensin II from the inactive polypeptide angiotensinogen by two subsequent proteolytic cleavages (see Chapter 42). Another example is activation of vitamin D by two subsequent hydroxylation reactions in the liver and kidney to produce the highly bioactive hormone 1,25-dihydroxyvitamin D (vitamin D).
CONFIGURATION OF FEEDBACK LOOPS WITHIN THE ENDOCRINE SYSTEM
There are two basic configurations of negative-feedback loops within the endocrine system: a physiological response–driven feedback loop (referred to simply as “response-driven feedback”) and an endocrine axis–driven feedback loop (Fig. 37-2). The response-driven feedback loop is observed in endocrine glands that control blood glucose levels (pancreatic islets), blood Ca++ and Pi levels (parathyroid glands, kidney), blood osmolarity and volume (hypothalamus/posterior pituitary), and blood Na+, K+, and H+ (zona glomerulosa of the adrenal cortex and atrial cells). In the response-driven configuration, secretion of a hormone is stimulated or inhibited by a change in the level of a specific extracellular parameter (e.g., an increase in blood glucose stimulates insulin secretion). Altered hormone levels lead to changes in the physiology of target organs (e.g., decreased hepatic gluconeogenesis, increased uptake of glucose by muscle) that directly regulate the parameter (i.e., blood glucose) in question. The change in the parameter (i.e., decreased blood glucose) then inhibits further secretion of the hormone (i.e., insulin secretion drops as blood glucose falls).
Much of the endocrine system is organized into endocrine axes, with each axis consisting of the hypothalamus and the pituitary and peripheral endocrine glands (Fig. 37-2). Thus, the endocrine axis–driven feedback loop involves a three-tiered configuration. The first tier is represented by hypothalamic neuroendocrine neurons that secrete releasing hormones. Releasing hormones stimulate (or, in a few cases, inhibit) the production and secretion of tropic hormones from the pituitary gland (second tier). Tropic hormones stimulate the production and secretion of hormones from peripheral endocrine glands (third tier). The peripherally produced hormones, namely, thyroid hormone, cortisol, sex steroids, and IGF-I, typically have pleiotropic actions (e.g., multiple phenotypic effects) on numerous cell types. However, in endocrine axis–driven feedback, the primary feedback loop involves feedback inhibition of pituitary tropic hormones and hypothalamic releasing hormones by the peripherally produced hormone. In contrast to response-driven feedback, the physiological responses to the peripherally produced hormone play only a minor role in regulation of feedback within endocrine axis–driven feedback loops.
An important aspect of the endocrine axes is the ability of descending and ascending neuronal signals to modulate release of the hypothalamic releasing hormones and thereby control the activity of the axis. A major neuronal input to releasing hormone–secreting neurons comes from another region of the hypothalamus called the suprachiasmatic nucleus (SCN). SCN neurons impose a daily rhythm, called a circadian rhythm, on the secretion of hypothalamic releasing hormones and the endocrine axes that they control (Fig. 37-3). SCN neurons represent an intrinsic circadian clock, as evidenced by the fact that they demonstrate a spontaneous peak of electrical activity at the same time every 24 to 25 hours. The 24- to 25-hour cycle can be “entrained” by the normal environmental light-dark cycle created by the earth’s rotation such that the periodicity of the clock appears to be environmentally controlled (Fig. 37-4). Neural input is generated from specialized light-sensitive retinal cells that are distinct from rods and cones and from signals to the SCN via the retinohypothalamic tract. Under constant conditions of light or dark, however, the SCN clock becomes “free running” and slightly drifts away from a 24-hour cycle each day.
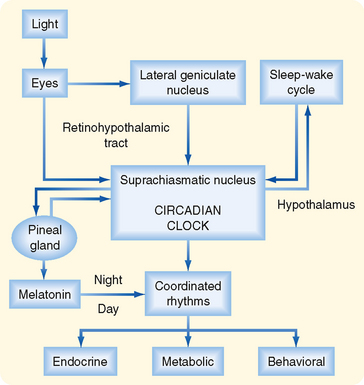
Figure 37-4 Origin of circadian rhythms in endocrine gland secretion, metabolic processes, and behavioral activity.
(Modified from Turek FW: Recent Prog Horm Res 49:43, 1994.)
The pineal gland forms a neuroendocrine link between the SCN and various physiological processes that require circadian control. This tiny gland, close to the hypothalamus, synthesizes the hormone melatonin from the neurotransmitter serotonin, of which tryptophan is the precursor. The rate-limiting enzyme for melatonin synthesis is N-acetyltransferase. The amount and activity of this enzyme in the pineal gland vary markedly in a cyclic fashion, which accounts for the cycling of melatonin secretion and its plasma levels. Synthesis of melatonin is inhibited by light and markedly stimulated by darkness (Fig. 37-4). Thus, melatonin may transmit the information that nighttime has arrived, and body functions are regulated accordingly. Melatonin feedback to the SCN at dawn or dusk may also help evoke day-night entrainment of the SCN 24- to 25-hour clock. Melatonin has numerous other actions, including induction of sleep.
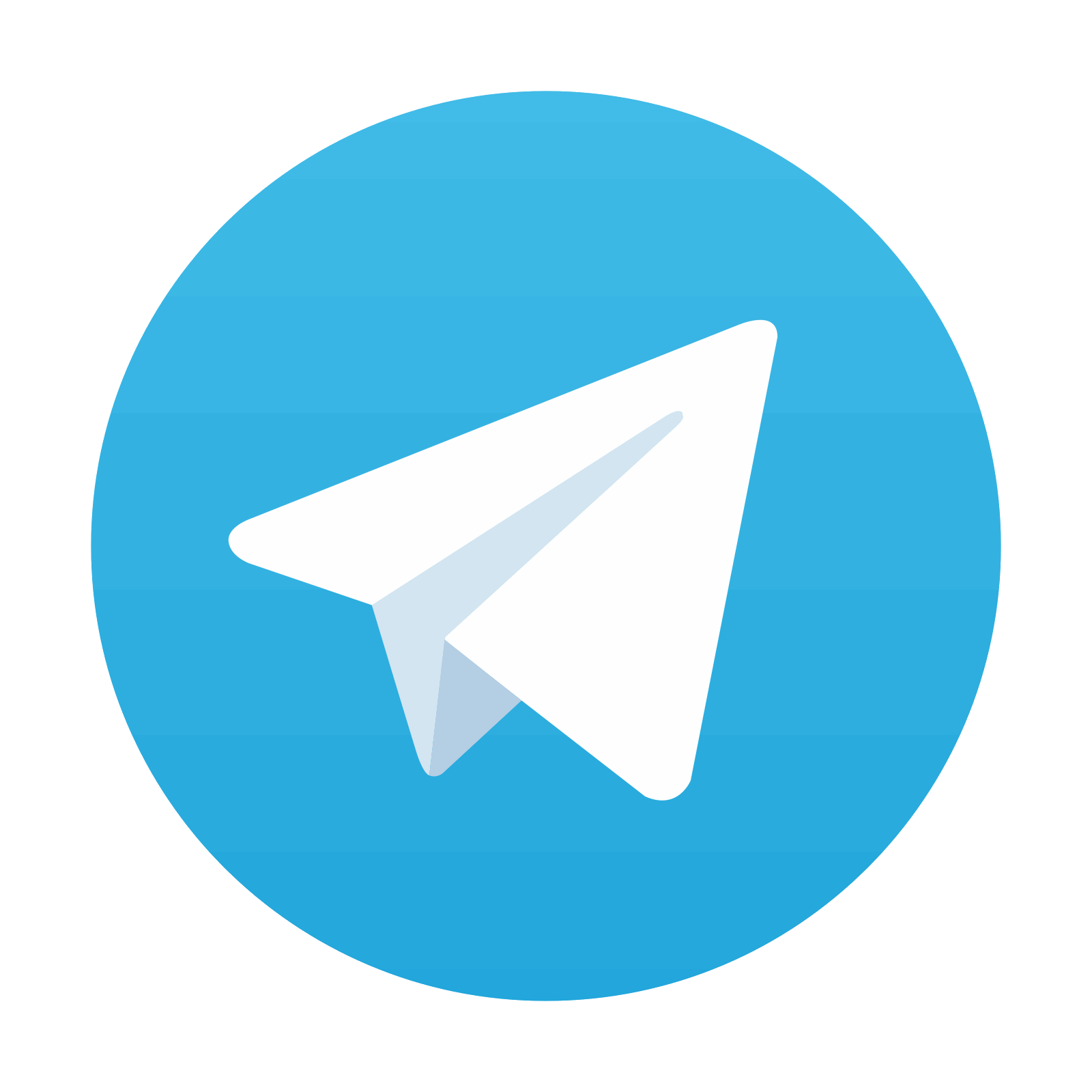