Introduction to Endocrinology: The Hypothalamic-Pituitary Axis
ENDOCRINOLOGY AND HORMONES: GENERAL CONCEPTS
Endocrinology analyzes the biosynthesis of hormones, their sites of production, and the sites and mechanisms of their action and interaction. The major functions of hormones include the regulation of energy storage, production, and utilization; the adaptation to new environments or conditions of stress; the facilitation of growth and development; and the maturation and function of the reproductive system. Although hormones were originally defined as products of ductless glands, we now appreciate that many organs not classically considered as “endocrine” (e.g., the heart, kidneys, GI tract, adipocytes, and brain) synthesize and secrete hormones that play key physiological roles. In addition, the field of endocrinology has expanded to include the actions of growth factors acting by means of autocrine and paracrine mechanisms, the influence of neurons—particularly those in the hypothalamus—that regulate endocrine function, and the reciprocal interactions of cytokines and other components of the immune system with the endocrine system.
Conceptually, hormones may be divided into 2 classes:
• Hormones that act predominantly via nuclear receptors to modulate transcription in target cells (e.g., steroid hormones, thyroid hormone, and vitamin D)
• Hormones that typically act via membrane receptors to exert rapid effects on signal transduction pathways (e.g., peptide and amino acid hormones)
The receptors for both classes of hormones provide tractable targets for a diverse group of compounds that are among the most widely used drugs in clinical medicine.
THE HYPOTHALAMIC-PITUITARY-ENDOCRINE AXIS
Many of the classic endocrine hormones (e.g., cortisol, thyroid hormone, sex steroids, growth hormone) are regulated by complex reciprocal interactions among the hypothalamus, anterior pituitary, and endocrine glands (Table 38–1). The basic organization of the hypothalamic-pituitary-endocrine axis is summarized in Figure 38–1.
Table 38–1
Hormones that Intergrate the Hypothalamic-Pituitary-Endocrine Axis
Figure 38–1 Organization of the anterior and posterior pituitary gland. Hypothalamic neurons in the supraoptic (SON) and paraventricular (PVN) nuclei synthesize arginine vasopressin (AVP) or oxytocin (OXY). Most of their axons project directly to the posterior pituitary, from which AVP and OXY are secreted into the systemic circulation to regulate their target tissues. Neurons that regulate the anterior lobe cluster in the mediobasal hypothalamus, including the PVN and the arcuate (ARC) nuclei. They secrete hypothalamic releasing hormones, which reach the anterior pituitary via the hypothalamic-adenohypophyseal portal system and stimulate distinct populations of pituitary cells. These cells, in turn, secrete the trophic (signal) hormones that regulate endocrine organs and other tissues. See Table 38–1 for abbreviations.
Discrete sets of hypothalamic neurons produce different releasing hormones, which are axonally transported to the median eminence. Upon stimulation, these neurons secrete their respective hypothalamic-releasing hormones into the hypothalamic-adenohypophyseal plexus, which flows to the anterior pituitary gland. The hypothalamic-releasing hormones bind to membrane receptors on specific subsets of pituitary cells and stimulate the secretion of the corresponding pituitary hormones. The pituitary hormones, which can be thought of as the master signals, then circulate to the target endocrine glands where they activate specific receptors to stimulate the synthesis and secretion of the target endocrine hormones. These interactions are feed-forward regulation in which the master (signal) hormones stimulate the production of target hormones by the endocrine organs.
Superimposed on this positive feed-forward regulation is negative feedback regulation, which permits precise control of hormone levels (Figure 38–2; see Figure 38–6). Typically, the endocrine target hormone circulates to both the hypothalamus and pituitary, where it acts via specific receptors to inhibit the production and secretion of both its hypothalamic-releasing hormone and the regulatory pituitary hormone. In addition, other brain regions have inputs to the hypothalamic-releasing neurons, further integrating the regulation of hormone levels in response to diverse stimuli.
Figure 38–2 Growth hormone secretion and actions. Two hypothalamic factors, growth hormone-releasing hormone (GHRH) and somatostatin (SST) stimulate or inhibit the release of growth hormone (GH) from the pituitary, respectively. Insulin-like growth factor-1 (IGF-1), a product of GH action on peripheral tissues, causes negative feedback inhibition of GH release by acting at the hypothalamus and the pituitary. The actions of GH can be direct or indirect (mediated by IGF-1). See text for discussion of the other agents that modulate GH secretion and of the effects of locally produced IGF-1. Inhibition, -; stimulation, +;.
PITUITARY HORMONES AND THEIR HYPOTHALAMIC-RELEASING FACTORS
The anterior pituitary hormones can be classified into 3 different groups based on their structural features (Table 38–2):
Table 38–2
Properties of the Protein Hormones of the Human Adenohypophysis and Placenta
• Pro-opiomelanocortin (POMC)-derived hormones include corticotropin (adrenocorticotrophic hormone [ACTH]) and α-melanocyte-stimulating hormone (α-MSH). These are derived from POMC by proteolytic processing (see Chapters 18 and 42).
• Somatotropic hormones include growth hormone (GH) and prolactin. In humans the somatotropic family also includes placental lactogen.
• The glycoprotein hormones—thyroid-stimulating hormone (TSH; also called thyrotropin), luteinizing hormone (LH; also called lutropin), and follicle-stimulating hormone (FSH; also called follitropin). In humans, the glycoprotein hormone family also includes human chorionic gonadotropin (hCG).
The synthesis and release of anterior pituitary hormones are influenced by the central nervous system (CNS). Their secretion is positively regulated by a group of peptides referred to as hypothalamic-releasing hormones (see Figure 38–1). These include corticotropin-releasing hormone (CRH), growth hormone-releasing hormone (GHRH), gonadotropin-releasing hormone (GnRH), and thyrotropin-releasing hormone (TRH). Somatostatin (SST), another hypothalamic peptide, negatively regulates secretion of pituitary GH and TSH. The neurotransmitter dopamine inhibits the secretion of prolactin by lactotropes.
The posterior pituitary gland, also known as the neurohypophysis, contains the endings of nerve axons arising from the hypothalamus that synthesize either arginine vasopressin or oxytocin (see Figure 38–1). Arginine vasopressin plays an important role in water homeostasis (see Chapter 25); oxytocin plays important roles in labor and parturition and in milk letdown, as discussed in the following sections and in Chapter 66.
SOMATOTROPIC HORMONES: GROWTH HORMONE AND PROLACTIN
GH and prolactin are structurally related members of the somatotropic hormone family and share many biological features. The somatotropes and lactotropes, the pituitary cells that produce and secrete GH and prolactin, respectively, are subject to strong inhibitory input from hypothalamic neurons; for prolactin, this negative dopaminergic input is the dominant regulator of secretion. GH and prolactin act via membrane receptors that belong to the cytokine receptor family and modulate target cell function via very similar signal transduction pathways (see Chapter 3). Several drugs that are used to treat excessive secretion of these hormones are effective to varying degrees for both GH and prolactin.
PHYSIOLOGY
Table 38–2 presents some features of the somatotrophic hormones.
GH is secreted by somatotropes as a heterogeneous mixture of peptides; the principal form is a single polypeptide chain of 22 kDa that has 2 disulfide bonds and is not glycosylated. Alternative splicing produces a smaller form (~20 kDa) with equal bioactivity that makes up 5-10% of circulating GH. Recombinant human GH consists entirely of the 22 kDa form, which provides a way to detect GH abuse. In the circulation, a 55 kDa protein binds approximately 45% of the 22 kDa and 25% of the 20 kDa forms. A second protein unrelated to the GH receptor also binds approximately 5-10% of circulating GH with lower affinity. Bound GH is cleared more slowly and has a biological t1/2 ~10 times that of unbound GH, suggesting that the bound hormone may provide a GH reservoir that dampens acute fluctuations in GH levels associated with its pulsatile secretion.
REGULATION OF SECRETION
GH secretion is high in children, peaks during puberty, and then decreases in an age-related manner in adulthood. GH is secreted in discrete but irregular pulses. The amplitude of secretory pulses is greatest at night. GHRH, produced by hypothalamic neurons, stimulates GH secretion (see Figure 38–2) by binding to a specific GPCR on somatotropes. The stimulated GHRH receptor couples to Gs to raise intracellular levels of cyclic AMP and Ca2+, thereby stimulating GH synthesis and secretion. Loss-of-function mutations of the GHRH receptor cause a rare form of short stature in humans. GH and its major peripheral effector, insulin-like growth factor 1 (IGF-1), act in negative feedback loops to suppress GH secretion. The negative effect of IGF-1 is predominantly through direct effects on the anterior pituitary gland, while the negative feedback action of GH is mediated in part by SST, synthesized in more widely distributed neurons.
SST is synthesized as a 92–amino acid precursor and processed by proteolytic cleavage to generate 2 peptides: SST-28 and SST-14 (Figure 38–3). SST exerts its effects by binding to and activating a family of 5 related GPCRs that signal through Gi to inhibit cyclic AMP formation and to activate K+ channels and protein phosphotyrosine phosphatases.
Figure 38–3 Structures of somatostatin-14 and selected synthetic analogs. Residues that play key roles in binding to SST receptors are shown in red. Octreotide and lanreotide are clinically available synthetic analogs of somatostatin. D-Nal, 3-(2-napthyl)-D-alanyl.
Ghrelin, a 28-amino acid peptide, stimulates GH secretion. Ghrelin is synthesized predominantly in endocrine cells in the fundus of the stomach but also is produced at lower levels at a number of other sites. Both fasting and hypoglycemia stimulate circulating ghrelin levels. Ghrelin acts primarily through a GPCR called the GH secretagogue receptor. Ghrelin also stimulates appetite and increases food intake, apparently by central actions on NPY and agouti-related peptide neurons in the hypothalamus. Thus, ghrelin and its receptor act in a complex manner to integrate the functions of the GI tract, the hypothalamus, and the anterior pituitary.
Several neurotransmitters, drugs, metabolites, and other stimuli modulate the release of GHRH and/or SST and thereby affect GH secretion. DA, 5HT, and α2 adrenergic receptor agonists stimulate GH release, as do hypoglycemia, exercise, stress, emotional excitement, and ingestion of protein-rich meals. In contrast, β adrenergic receptor agonists, free fatty acids, glucose, IGF-1, and GH itself inhibit release. Many of the physiological factors that influence prolactin secretion also affect GH secretion. Thus, sleep, stress, hypoglycemia, exercise, and estrogen increase the secretion of both hormones.
Prolactin is unique among the anterior pituitary hormones in that hypothalamic regulation of its secretion is predominantly inhibitory. The major regulator of prolactin secretion is DA, which interacts with the D2 receptor, a GPCR on lactotropes, to inhibit prolactin secretion (Figure 38–4). Prolactin acts predominantly in women, both during pregnancy and in the postpartum period in women who breast-feed. During pregnancy, the maternal serum prolactin level starts to increase at 8 weeks of gestation, peaks to 250 ng/mL at term, and declines thereafter to prepregnancy levels unless the mother breast-feeds the infant. Suckling or breast manipulation in nursing mothers cause elevation of circulating prolactin levels. Prolactin levels can rise 10- to 100-fold within 30 min of stimulation. This response is distinct from milk letdown, which is mediated by oxytocin release from the posterior pituitary gland. The suckling response becomes less pronounced after several months of breastfeeding, and prolactin concentrations eventually decline to prepregnancy levels. Prolactin also is synthesized in lactotropes near the end of the luteal phase of the menstrual cycle and by decidual cells early in pregnancy (accounting for the high levels of prolactin in amniotic fluid during the first trimester of human pregnancy).
Figure 38–4 Prolactin secretion and actions. Prolactin is the only anterior pituitary hormone for which a unique stimulatory releasing factor has not been identified. Thyrotropin-releasing hormone (TRH), however, can stimulate prolactin release; dopamine inhibits it. Suckling induces prolactin secretion, and prolactin affects lactation and reproductive functions but also has effects on many other tissues. Prolactin is not under feedback control by peripheral hormones.
MOLECULAR AND CELLULAR BASES OF SOMATOTROPIC HORMONE ACTION
Receptors for GH and prolactin belong to the cytokine receptor superfamily; they contain an extracellular hormone-binding domain, a single membrane-spanning region, and an intracellular domain that mediates signal transduction.
GH receptor activation results in the binding of a single GH to 2 receptor monomers and to form a GH-GH receptor ternary complex (initiated by high-affinity interaction of GH with 1 monomer of the GH receptor dimer [mediated by GH site 1], followed by a second, lower affinity interaction of GH with the GH receptor [mediated by GH site 2]); these interactions induce a conformational change that activates downstream signaling. The ligand-occupied GH receptor dimer lacks inherent tyrosine kinase activity but provides docking sites for 2 molecules of JAK2, a cytoplasmic tyrosine kinase of the Janus kinase family. The juxtaposition of 2 JAK2 molecules leads to trans-phosphorylation and autoactivation of JAK2, with consequent tyrosine phosphorylation of cytoplasmic proteins that mediate downstream signaling events (Figure 38–5). Pegvisomant is a GH analog with amino acid substitutions that disrupt the interaction at site 2; pegvisomant binds to the receptor and causes its internalization but cannot trigger the conformational change that stimulates downstream events in the signal transduction pathway.
Figure 38–5 Mechanisms of growth hormone and prolactin action and of GH receptor antagonism. A. Binding of GH to a growth hormone receptor (GHR) homodimer induces autophosphorylation of JAK2. JAK2 then phosphorylates cytoplasmic proteins that activate downstream signaling pathways, including STAT5 and mediators upstream of MAPK, which ultimately modulate gene expression. The structurally related prolactin receptor also is a ligand activated homodimer that recruits the JAK-STAT signaling pathway. GHR also activates IRS-1, which may mediate the increased expression of glucose transporters on the plasma membrane. B. Pegvisomant, a recombinant pegylated variant of human GH, is a high affinity GH antagonist that interferes with GH signaling. JAK2, Janus kinase 2; IRS-1, insulin receptor substrate-1; PI3K, phosphatidyl inositol-3 kinase; STAT, signal transducer and activator of transcription; MAPK, mitogen-activated protein kinase; SHC, Src homology-containing proteins.
The effects of prolactin on target cells also result from interactions with a cytokine family receptor that is widely distributed and signals through many of the same pathways as the GH receptor. Unlike human GH and placental lactogen, which also bind to the prolactin receptor and thus are lactogenic, prolactin binds specifically to the prolactin receptor and has no somatotropic (GH-like) activity.
PHYSIOLOGICAL EFFECTS OF THE SOMATOTROPIC HORMONES
The most striking physiological effect of GH is the stimulation of the longitudinal growth of bones. GH also increases bone mineral density after the epiphyses have closed. GH also increases muscle mass (in human subjects with GH deficiency), increases glomerular filtration rate, and stimulates of preadipocyte differentiation into adipocytes. Growth hormone acts directly on adipocytes to increase lipolysis and on hepatocytes to stimulate gluconeogenesis, but its anabolic and growth-promoting effects are mediated indirectly through the induction of IGF-1. IGF-1 interacts with receptors on the cell surface that mediate its biological activities.
Prolactin effects are limited to tissues that express the prolactin receptor, particularly the mammary gland. Prolactin plays an important role in inducing growth and differentiation of the ductal and lobuloalveolar epithelia and is essential for lactation. Prolactin receptors are present in many other sites, including the hypothalamus, liver, adrenal, testes, ovaries, prostate, and immune system, suggesting that prolactin may play multiple roles outside of the breast. The physiological effects of prolactin at these sites remain poorly characterized.
PATHOPHYSIOLOGY OF THE SOMATOTROPIC HORMONES
EXCESS PRODUCTION OF SOMATOTROPIC HORMONES. Syndromes of excess secretion of GH and prolactin typically are caused by somatotrope or lactotrope adenomas that oversecrete the respective hormones.
Clinical Manifestations of Excess GH. GH excess causes distinct clinical syndromes depending on the age of the patient. If the epiphyses are unfused, GH excess causes increased longitudinal growth, resulting in gigantism. In adults, GH excess causes acromegaly. The symptoms and signs of acromegaly (e.g., arthropathy, carpal tunnel syndrome, generalized visceromegaly, macroglossia, hypertension, glucose intolerance, headache, lethargy, excess perspiration, and sleep apnea) progress slowly, and diagnosis often is delayed. Mortality is increased at least 2-fold relative to age-matched controls, predominantly due to increased death from cardiovascular disease.
Clinical Manifestations of Excess Prolactin. Hyperprolactinemia is a relatively common endocrine abnormality that can result from hypothalamic or pituitary diseases that interfere with the delivery of inhibitory dopaminergic signals, from renal failure, from primary hypothyroidism associated with increased TRH levels, or from treatment with dopamine receptor antagonists. Most often, hyperprolactinemia is caused by prolactin-secreting pituitary adenomas. Manifestations of prolactin excess in women include galactorrhea, amenorrhea, and infertility. In men, hyperprolactinemia causes loss of libido, erectile dysfunction, and infertility.
IMPAIRED PRODUCTION OF THE SOMATOTROPIC HORMONES
Clinical Manifestations of Growth Hormone Deficiency.
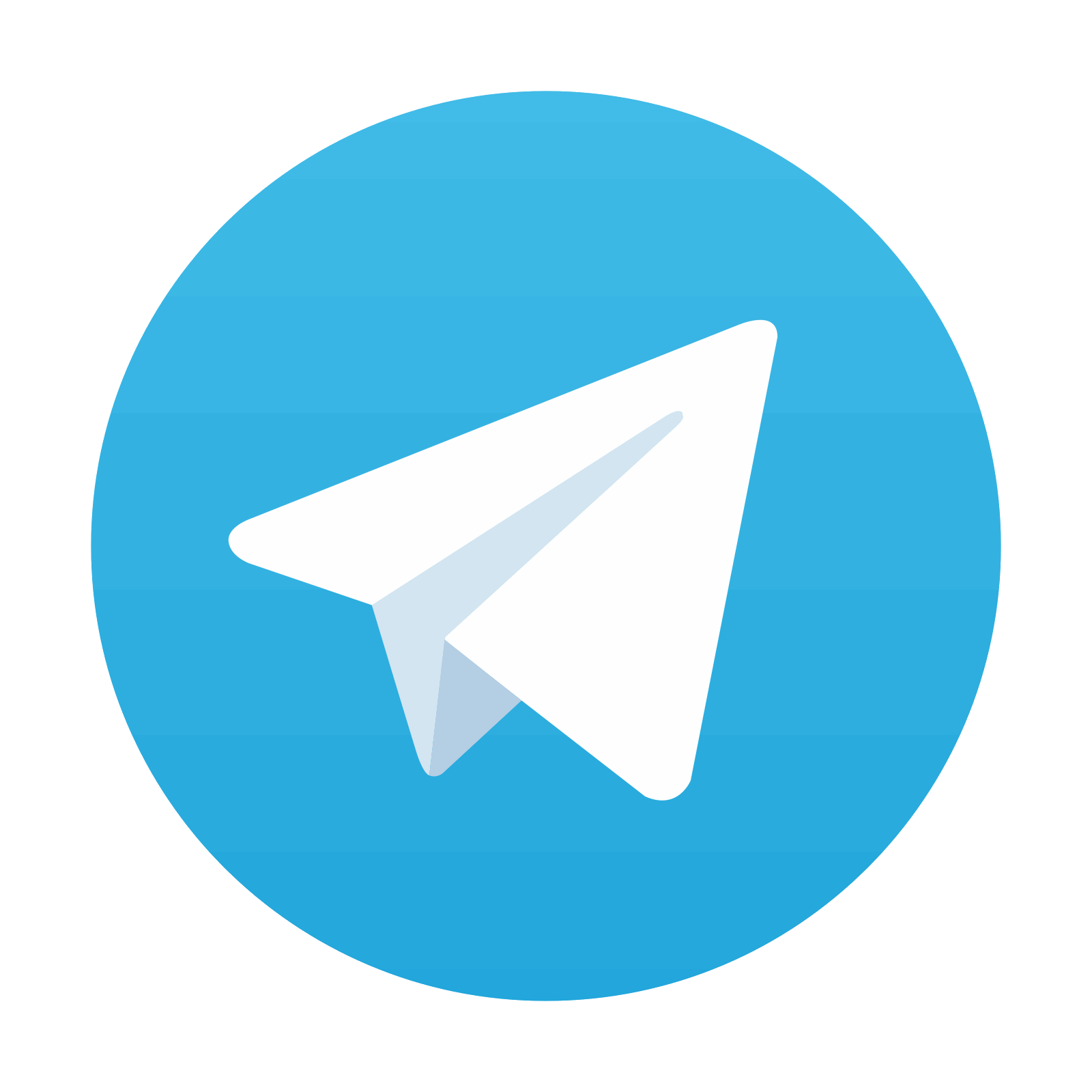
Stay updated, free articles. Join our Telegram channel

Full access? Get Clinical Tree
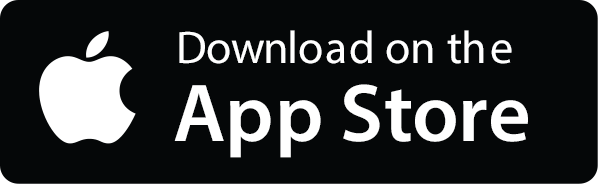
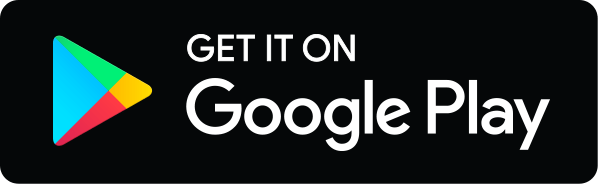